C0
Enzymes: A Practical Introduction to Structure, Mechanism, and Data Analysis.
Robert A. Copeland
Copyright 2000 by Wiley-VCH, Inc.
ISBNs: 0-471-35929-7 (Hardback); 0-471-22063-9 (Electronic)
Life depends on a well-orchestrated series of chemical reactions. Many of thesereactions, however, proceed too slowly on their own to sustain life. Hencenature has designed catalysts, which we now refer to as
enzymes, to greatlyaccelerate the rates of these chemical reactions. The catalytic power of enzymesfacilitates life processes in essentially all life-forms from viruses to man. Manyenzymes retain their catalytic potential after extraction from the living organ-ism, and it did not take long for mankind to recognize and exploit the catalyticpower of enzyme for commercial purposes. In fact, the earliest known refer-ences to enzymes are from ancient texts dealing with the manufacture ofcheeses, breads, and alcoholic beverages, and for the tenderizing of meats.
Today enzymes continue to play key roles in many food and beveragemanufacturing processes and are ingredients in numerous consumer products,such as laundry detergents (which dissolve protein-based stains with the helpof proteolytic enzymes). Enzymes are also of fundamental interest in the healthsciences, since many disease processes can be linked to the aberrant activitiesof one or a few enzymes. Hence, much of modern pharmaceutical research isbased on the search for potent and specific inhibitors of these enzymes. Thestudy of enzymes and the action of enzymes has thus fascinated scientists sincethe dawn of history, not only to satisfy erudite interest but also because of theutility of such knowledge for many practical needs of society. This brief chaptersets the stage for our studies of these remarkable catalysts by providing ahistoric background of the development of enzymology as a science. We shallsee that while enzymes are today the focus of basic academic research, muchof the early history of enzymology is linked to the practical application ofenzyme activity in industry.
A BRIEF HISTORY OF ENZYMOLOGY
ENZYMES IN ANTIQUITY
The oldest known reference to the commercial use of enzymes comes from adescription of wine making in the Codex of Hammurabi (ancient Babylon,circa 2100 ..). The use of microorganisms as enzyme sources for fermentationwas widespread among ancient people. References to these processes can befound in writings not only from Babylon but also from the early civilizationsof Rome, Greece, Egypt, China, India. Ancient texts also contain a number ofreferences to the related process of vinegar production, which is based on theenzymatic conversion of alcohol to acetic acid. Vinegar, it appears, was acommon staple of ancient life, being used not only for food storage andpreparation but also for medicinal purposes.
Dairy products were another important food source in ancient societies.
Because in those days fresh milk could not be stored for any reasonable lengthof time, the conversion of milk to cheese became a vital part of foodproduction, making it possible for the farmer to bring his product to distantmarkets in an acceptable form. Cheese is prepared by curdling milk via theaction of any of a number of enzymes. The substances most commonly usedfor this purpose in ancient times were ficin, obtained as an extract from figtrees, and rennin, as rennet, an extract of the lining of the fourth stomach of amultiple-stomach animal, such as a cow. A reference to the enzymatic activityof ficin can, in fact, be found in Homer's classic, the Iliad:
As the juice of the fig tree curdles milk, and thickens it in a moment though it beliquid, even so instantly did Paee¨on cure fierce Mars.
The philosopher Aristotle likewise wrote several times about the process ofmilk curdling and offered the following hypothesis for the action of rennet:
Rennet is a sort of milk; it is formed in the stomach of young animals while stillbeing suckled. Rennet is thus milk which contains fire, which comes from the heatof the animal while the milk is undergoing concoction.
Another food staple throughout the ages is bread. The leavening of bread
by yeast, which results from the enzymatic production of carbon dioxide, waswell known and widely used in ancient times. The importance of this processto ancient society can hardly be overstated.
Meat tenderizing is another enzyme-based process that has been used since
antiquity. Inhabitants of many Pacific islands have known for centuries thatthe juice of the papaya fruit will soften even the toughest meats. The activeenzyme in this plant extract is a protease known as papain, which is used eventoday in commercial meat tenderizers. When the British Navy began exploringthe Pacific islands in the 1700s, they encountered the use of the papaya fruitas a meat tenderizer and as a treatment for ringworm. Reports of these nativeuses of the papaya sparked a great deal of interest in eighteenth-century
Europe, and may, in part, have led to some of the more systematic studies ofdigestive enzymes that ensued soon after.
While the ancients made much practical use of enzymatic activity, these earlyapplications were based purely on empirical observations and folklore, ratherthan any systematic studies or appreciation for the chemical basis of theprocesses being utilized. In the eighteenth and nineteenth centuries scientistsbegan to study the actions of enzymes in a more systematic fashion. Theprocess of digestion seems to have been a popular subject of investigationduring the years of the enlightenment. Wondering how predatory birds manageto digest meat without a gizzard, the famous French scientist Re´aumur(1683
—1757) performed some of the earliest studies on the digestion ofbuzzards. Re´aumur designed a metal tube with a wire mesh at one end thatwould hold a small piece of meat immobilized, to protect it from the physicalaction of the stomach tissue. He found that when a tube containing meat wasinserted into the stomach of a buzzard, the meat was digested within 24 hours.
Thus he concluded that digestion must be a chemical rather than a merelyphysical process, since the meat in the tube had been digested by contact withthe gastric juices (or, as he referred to them, ‘‘a solvent''). He tried the sameexperiment with a piece of bone and with a piece of a plant. He found thatwhile meat was digested, and the bone was greatly softened by the action ofthe gastric juices, the plant material was impervious to the ‘‘solvent''; this wasprobably the first experimental demonstration of enzyme specificity.
Re´aumur's work was expanded by Spallanzani (1729
—1799), who showed
that the digestion of meat encased in a metal tube took place in the stomachsof a wide variety of animals, including humans. Using his own gastric juices,Spallanzani was able to perform digestion experiments on pieces of meat invitro (in the laboratory). These experiments illustrated some critical features ofthe active ingredient of gastric juices: by means of a control experiment inwhich meat treated with an equal volume of water did not undergo digestionSpallanzani demonstrated the presence of a specific active ingredient in gastricjuices. He also showed that the process of digestion is temperature dependent,and that the time required for digestion is related to the amount of gastricjuices applied to the meat. Finally, he demonstrated that the active ingredientin gastric juices is unstable outside the body; that is, its ability to digest meatwanes with storage time.
Today we recognize all the foregoing properties as common features of
enzymatic reactions, but in Spallanzani's day these were novel and excitingfindings. The same time period saw the discovery of enzyme activities in a largenumber of other biological systems. For example, a peroxidase from thehorseradish was described, and the action of -amylase in grain was observed.
These early observations all pertained to materials — crude extract from plantsor animals — that contained enzymatic activity.
A BRIEF HISTORY OF ENZYMOLOGY
During the latter part of the nineteenth century scientists began to attempt
fractionations of these extracts to obtain the active ingredients in pure form.
For example, in 1897 Bertrand partially purified the enzyme laccase from treesap, and Buchner, using the ‘‘pressed juice'' from rehydrated dried yeast,demonstrated that alcoholic fermentation could be performed in the absenceof living yeast cells. Buchner's report contained the interesting observation thatthe activity of the pressed juice diminished within 5 days of storage at icetemperatures. However, if the juice was supplemented with cane sugar, theactivity remained intact for up to 2 weeks in the ice box. This is probably thefirst report of a now well-known phenomenon — the stabilization of enzymesby substrate. It was also during this period that Ku¨hne, studying catalysis inyeast extracts, first coined the term ‘‘enzyme'' (the word derives from themedieval Greek word
enzymos, which relates to the process of leavening bread).
THE DEVELOPMENT OF MECHANISTIC ENZYMOLOGY
As enzymes became available in pure, or partially pure forms, scientists'attention turned to obtaining a better understanding of the details of thereaction mechanisms catalyzed by enzymes. The concept that enzymes formcomplexes with their substrate molecules was first articulated in the latenineteenth century. It is during this time period that Emil Fischer proposed the‘‘lock and key'' model for the stereochemical relationship between enzymes andtheir substrates; this model emerged as a result of a large body of experimentaldata on the stereospecificity of enzyme reactions. In the early twentieth century,experimental evidence for the formation of an enzyme
—substrate complex as areaction intermediate was reported. One of the earliest of these studies,reported by Brown in 1902, focused on the velocity of enzyme-catalyzedreactions. Brown made the insightful observation that unlike simple diffusion-limited chemical reactions, in enzyme-catalyzed reactions ‘‘it is quite conceiv-able . . that the time elapsing during molecular union and transformation maybe sufficiently prolonged to influence the general course of the action.'' Brownthen went on to summarize the available data that supported the concept offormation of an enzyme
—substrate complex:
There is reason to believe that during inversion of cane sugar by invertase thesugar combines with the enzyme previous to inversion. C. O'Sullivan andTompson . . have shown that the activity of invertase in the presence of canesugar survives a temperature which completely destroys it if cane sugar is notpresent, and regard this as indicating the existence of a combination of theenzyme and sugar molecules. Wurtz [1880] has shown that papain appears toform an insoluble compound with fibrin previous to hydrolysis. Moreover, themore recent conception of E. Fischer with regard to enzyme configuration andaction, also implies some form of combination of enzyme and reacting substrate.
Observations like these set the stage for the derivation of enzyme rate
equations, by mathematically modeling enzyme kinetics with the explicit
STUDIES OF ENZYME STRUCTURE
involvement of an intermediate enzyme
—substrate complex. In 1903 VictorHenri published the first successful mathematical model for describing enzymekinetics. In 1913, in a much more widely read paper, Michaelis and Mentenexpanded on the earlier work of Henri and rederived the enzyme rate equationthat today bears their names. The Michaelis
—Menten equation, or morecorrectly the Henri
—Michaelis
—Menten equation, is a cornerstone of much ofthe modern analysis of enzyme reaction mechanisms.
The question of how enzymes accelerate the rates of chemical reactions
puzzled scientists until the development of transition state theory in the firsthalf of the twentieth century. In 1948 the famous physical chemist LinusPauling suggested that enzymatic rate enhancement was achieved by stabiliz-ation of the transition state of the chemical reaction by interaction with theenzyme active site. This hypothesis, which was widely accepted, is supportedby the experimental observation that enzymes bind very tightly to moleculesdesigned to mimic the structure of the transition state of the catalyzed reaction.
In the 1950s and 1960s scientists reexamined the question of how enzymes
achieve substrate specificity in light of the need for transition state stabilizationby the enzyme active site. New hypotheses, such as the ‘‘induced fit'' model ofKoshland emerged at this time to help rationalize the competing needs ofsubstrate binding affinity and reaction rate enhancement by enzymes. Duringthis time period, scientists struggled to understand the observation thatmetabolic enzyme activities can be regulated by small molecules other than thesubstrates or direct products of an enzyme. Studies showed that indirectinteractions between distinct binding sites within an enzyme molecule couldoccur, even though these binding sites were quite distant from one another. In1965 Monod, Wyman, and Changeux developed the theory of allosterictransitions to explain these observations. Thanks in large part to this landmarkpaper, we now know that many enzymes, and nonenzymatic ligand bindingproteins, display allosteric regulation
STUDIES OF ENZYME STRUCTURE
One of the tenets of modern enzymology is that catalysis is intimately relatedto the molecular interactions that take place between a substrate molecule andcomponents of the enzyme molecule, the exact nature and sequence of theseinteractions defining per se the catalytic mechanism. Hence, the application ofphysical methods to elucidate the structures of enzymes has had a rich historyand continues to be of paramount importance today. Spectroscopic methods,x-ray crystallography, and more recently, multidimensional NMR methodshave all provided a wealth of structural insights on which theories of enzymemechanisms have been built. In the early part of the twentieth century, x-raycrystallography became the premier method for solving the structures of smallmolecules. In 1926 James Sumner published the first crystallization of anenzyme, urease (Figure 1.1). Sumner's paper was a landmark contribution, not
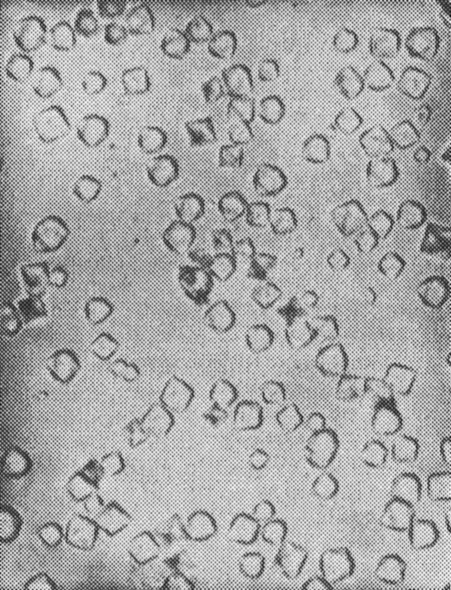
A BRIEF HISTORY OF ENZYMOLOGY
Figure 1.1
Photomicrograph of urease crystals (728; magnification), the first reported
crystals of an enzyme. [From J. B. Sumner, J. Biol. Chem. 69, 435—441 (1926), with
permission.]
only because it portended the successful application of x-ray diffraction forsolving enzyme structures, but also because a detailed analysis allowed Sumnerto show unequivocally that the crystals were composed of protein and thattheir dissolution in solvent led to enzymatic activity. These observations werevery important to the development of the science of enzymology because theyfirmly established the protein composition of enzymes, a view that had notbeen widely accepted by Sumner's contemporaries.
Sumner's crystallization of urease opened a floodgate and was quickly
followed by reports of numerous other enzyme crystals. Within 20 years ofSumner's first paper more than 130 enzyme crystals had been documented. Itwas not, however, until the late 1950s that protein structures began to besolved through x-ray crystallography. In 1957 Kendrew became the first todeduce from x-ray diffraction the entire three-dimensional structure of aprotein, myoglobin. Soon after, the crystal structures of many proteins,including enzymes, were solved by these methods. Today, the structural
insights gained from x-ray crystallography and multidimensional NMR studiesare commonly used to elucidate the mechanistic details of enzyme catalysis,and to design new ligands (substrate and inhibitor molecules) to bind atspecific sites within the enzyme molecule.
The deduction of three-dimensional structures from x-ray diffraction or
NMR methods depends on knowledge of the arrangement of amino acidsalong the polypeptide chain of the protein; this arrangement is known as theamino acid sequence. To determine the amino acid sequence of a protein, thecomponent amino acids must be hydrolyzed in a sequential fashion from thepolypeptide chain and identified by chemical or chromatographic analysis.
Edman and coworkers developed a method for the sequential hydrolysis ofamino acids from the N-terminus of a polypeptide chain. In 1957 Sangerreported the first complete amino acid sequence of a protein, the hormoneinsulin, utilizing the chemistry developed by Edman. In 1963 the first aminoacid sequence of an enzyme, ribonuclease, was reported.
1.5 ENZYMOLOGY TODAY
Fundamental questions still remain regarding the detailed mechanisms ofenzyme activity and its relationship to enzyme structure. The two mostpowerful tools that have been brought to bear on these questions in moderntimes are the continued development and use of biophysical probes of proteinstructure, and the application of molecular biological methods to enzymology.
X-ray crystallography continues to be used routinely to solve the structures ofenzymes and of enzyme—ligand complexes. In addition, new NMR methodsand magnetization transfer methods make possible the assessment of thethree-dimensional structures of small enzymes in solution, and the structure ofligands bound to enzymes, respectively.
The application of Laue diffraction with synchrotron radiation sources
holds the promise of allowing scientists to determine the structures of reactionintermediates during enzyme turnover, hence to develop detailed pictures of theindividual steps in enzyme catalysis. Other biophysical methods, such asoptical (e.g., circular dichroism, UV—visible, fluorescence) and vibrational (e.g.,infrared, Raman) spectroscopies, have likewise been applied to questions ofenzyme structure and reactivity in solution. Technical advances in many ofthese spectroscopic methods have made them extremely powerful and access-ible tools for the enzymologist. Furthermore, the tools of molecular biologyhave allowed scientists to clone and express enzymes in foreign host organismswith great efficiency. Enzymes that had never before been isolated have beenidentified and characterized by molecular cloning. Overexpression of enzymesin prokaryotic hosts has allowed the purification and characterization ofenzymes that are available only in minute amounts from their natural sources.
This has been a tremendous advance for protein science in general.
The tools of molecular biology also allow investigators to manipulate the
A BRIEF HISTORY OF ENZYMOLOGY
amino acid sequence of an enzyme at will. The use of site-directed mutagenesis(in which one amino acid residue is substituted for another) and deletionalmutagenesis (in which sections of the polypeptide chain of a protein areeliminated) have allowed enzymologists to pinpoint the chemical groups thatparticipate in ligand binding and in specific chemical steps during enzymecatalysis.
The study of enzymes remains of great importance to the scientific commu-
nity and to society in general. We continue to utilize enzymes in manyindustrial applications. Moreover enzymes are still in use in their traditionalroles in food and beverage manufacturing. In modern times, the role ofenzymes in consumer products and in chemical manufacturing has expandedgreatly. Enzymes are used today in such varied applications as stereospecificchemical synthesis, laundry detergents, and cleaning kits for contact lenses.
Perhaps one of the most exciting fields of modern enzymology is the
application of enzyme inhibitors as drugs in human and veterinary medicine.
Many of the drugs that are commonly used today function by inhibitingspecific enzymes that are associated with the disease process. Aspirin, forexample, one of the most widely used drugs in the world, elicits its anti-inflammatory efficacy by acting as an inhibitor of the enzyme prostaglandinsynthase. As illustrated in Table 1.1, enzymes take part in a wide range ofhuman pathophysiologies, and many specific enzyme inhibitors have beendeveloped to combat their activities, thus acting as therapeutic agents. Severalof the inhibitors listed in Table 1.1 are the result of the combined use ofbiophysical methods for assessing enzyme structure and classical pharmacol-ogy in what is commonly referred to as rational or structure-based drug design.
This approach uses the structural information obtained from x-ray crystallog-raphy or NMR spectroscopy to determine the topology of the enzyme activesite. Next, model building is performed to design molecules that would fit wellinto this active site pocket. These molecules are then synthesized and tested asinhibitors. Several iterations of this procedure often lead to extremely potentinhibitors of the target enzyme.
The list in Table 1.1 will continue to grow as our understanding of disease
state physiology increases. There remain thousands of enzymes involved inhuman physiology that have yet to be isolated or characterized. As more andmore disease-related enzymes are discovered and characterized, new inhibitorswill need to be designed to arrest the actions of these catalysts, in thecontinuing effort to fulfill unmet human medical needs.
We have seen in this chapter that the science of enzymology has a long andrich history. From phenomenological observations, enzymology has grown toa quantitative molecular science. For the rest of this book we shall viewenzymes from a chemical prospective, attempting to understand the actions of
Table 1.1
Examples of enzyme inhibitors as potential drugs
Carbonic anhydrase
Viral DNA polymerase
Aspirin, ibuprofen,
Inflammation, pain, fever
Prostaglandin synthase
-Lactam antibiotics
Bacterial infections
Organ transplantation
Hypertension, congestive
Bacterial resistance
Organ transplantation
Congestive heart failure
cAMP phosphodiesterase
Benign prostate hyperplasia
Organ transplantation,
FK-506 binding protein
autoimmune disease
Thymidilate synthase
Bacterial infection
Lung elastin degradation
in cystic fibrosis
Neutrophil elastase
HMG CoA reductase
Dihydrofolate reductase
Parkinson's disease
Urinary tract infections
Metabolism of antineoplastic
Purine nucleoside phosphorylase
Brain monoamine oxidase
Diabetic retinopathy
Hypertension, congestive
heart failure, analgesia
Bacterial infection, malaria
Dihydropteorate synthase
Hormone-dependent tumors
Bacterial infection
Dihydrofolate reductase
Rhinovirus coat protein
HIV reverse transcriptase
Adapted and expanded from M. A. Navia and M. A. Murcko, Curr. Opin. Struct. Biol.
2, 202—210 (1992).
A BRIEF HISTORY OF ENZYMOLOGY
these proteins in the common language of chemical and physical forces. Whilethe vital importance of enzymes in biology cannot be overstated, the under-standing of their structures and functions remains a problem of chemistry.
REFERENCES AND FURTHER READING
Rather than providing an exhaustive list of primary references for thishistorical chapter, I refer the reader to a few modern texts that have done anexcellent jobof presenting a more detailed and comprehensive treatment of thehistory of enzymology. Not only do these books provide good descriptions ofthe history of science and the men and women who made that history, but theyare also quite entertaining and inspiring reading — enjoy them!
Friedmann, H. C., Ed. (1981) Enzymes, Hutchinson Ross, Stroudsburg, PA. [This book
is part of the series ‘‘Benchmark Papers in Biochemistry.'' In it, Friedmann hascompiled reprints of many of the most influential publications in enzymology fromthe eighteenth through twentieth centuries, along with insightful commentaries onthese papers and their importance in the development of the science.]
Judson, H. F. (1980) T he Eighth Day of Creation, Simon & Schuster, New York. [This
extremely entertaining book chronicles the history of molecular biology, includingprotein science and enzymology, in the twentieth century.]
Kornberg, A. (1989) For the L ove of Enzymes. T he Odyssey of a Biochemist, Harvard
University Press, Cambridge, MA. [An autobiographical look at the career of aNobel Prize—winning biochemist.]
Werth, B. (1994) T he Billion Dollar Molecule, Simon & Schuster, New York. [An
interesting, if biased, look at the modern science of structure-based drug design.]
Source: ftp://h74.net32.bmstu.ru/books/Medicine/Enzymes-2ed/chp1.pdf
Master of Laws (LL.M) Objectives: The program aims to provide broad education in law and academic disciplines. It aims to develop in students a wide appreciation of relevant subjects, their importance and rigorous academic challenges of current law, and to train them to be excellent law practitioners. The student will be engaged in private practice, or work for government or law agencies. Their training in the Program gives them the preparation they need to solve the challenging problems they encounter.
PASS CR (MICRO Type II) CAPE SEAL and PASS QB Rejuvenating Seal for Residential Roads SECTION 700 - PASS CR Scrub Seal The work shall consist of furnishing all necessary labor, materials and equipment for the transporting, application of the polymer modified asphaltic emulsion PASS or equal, ¼" by No. 10 premium aggregate to conform to the Provisions of Section 37-2, of the Standard Specifications, Plans and these Special Provisions. The work shall be done in the following order: preparing the pavement surface; applying the emulsion; scrubbing the applied emulsion with an emulsion broom; applying premium aggregate; rolling the ¼" by No. 10 premium aggregate; and sweeping up excess aggregate and more fully described below.