Microsoft word - final mudworm report.doc
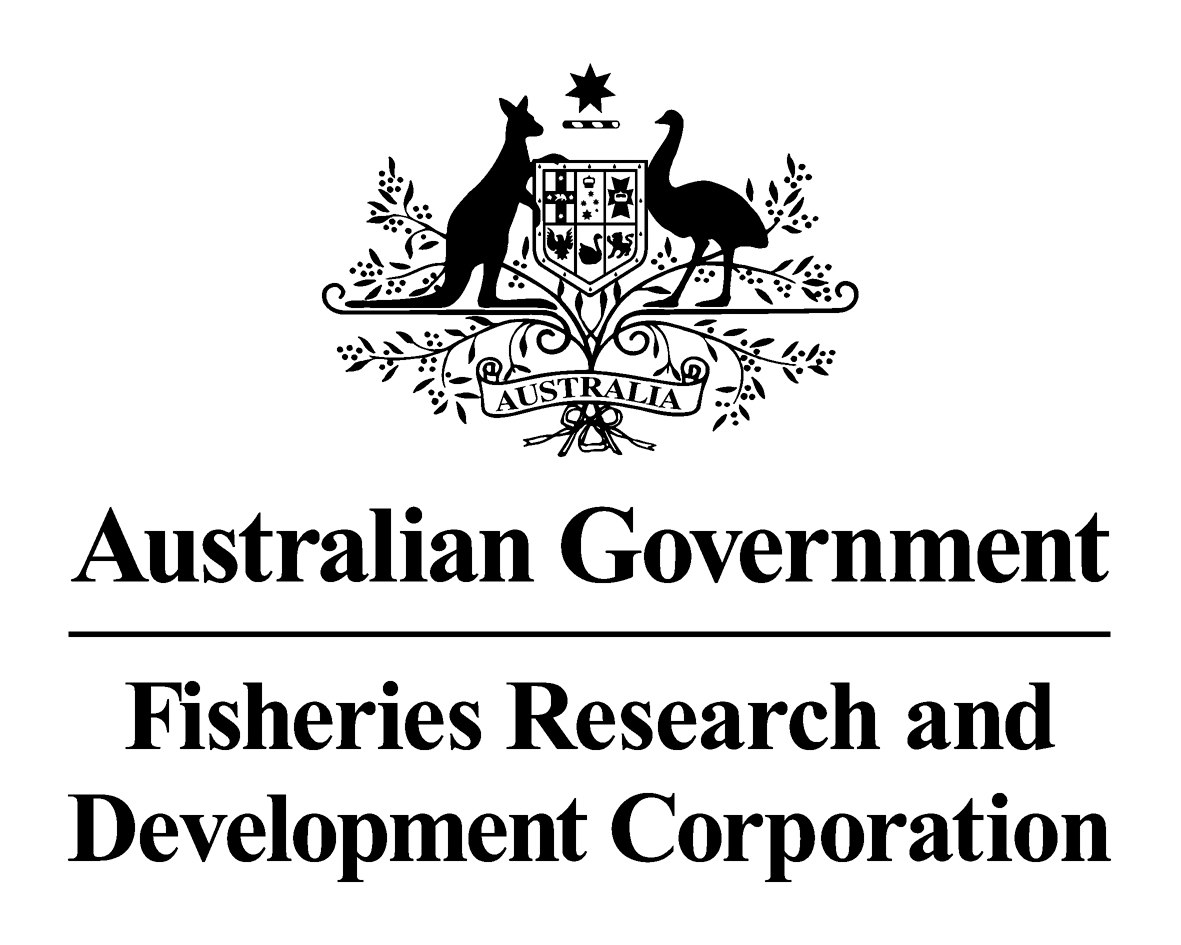
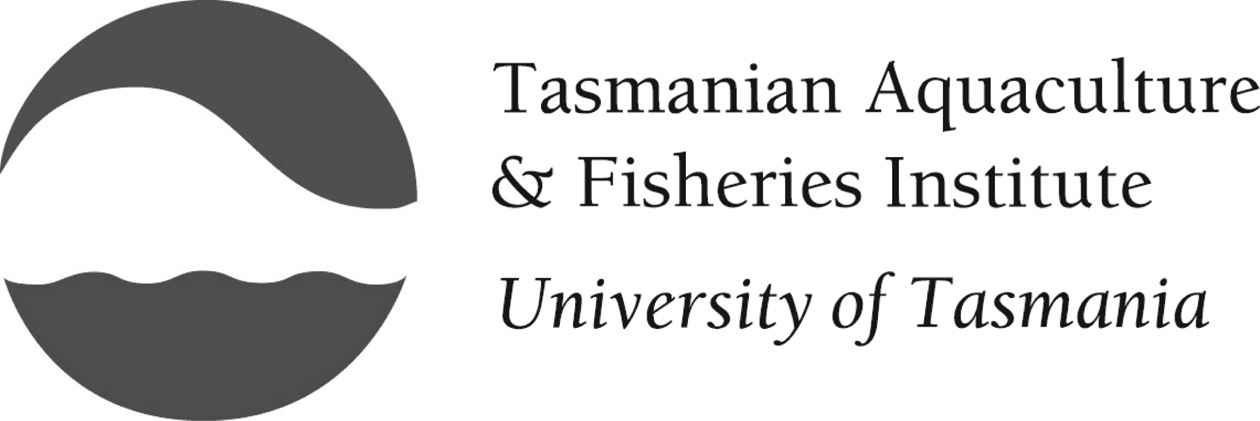
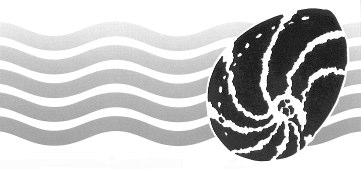
Mudworm control in abalone
DEVELOPMENT OF AN INTEGRATED
MANAGEMENT PROGRAM FOR THE CONTROL
OF SPIONID MUDWORMS IN CULTURED
Judith Handlinger, Mark Lleonart & Mark Powell
October 2004
FRDC Project No. 98/307
(Incorporating CRC for Aquaculture Project A.2.6.)
FRDC Final Report Page
Mudworm control in abalone
TABLE OF CONTENTS
SECTION 1 BACKGROUND
General background : spionid biology & impact………….….
The original Tasmanian abalone mortality episode………….
Previous & preliminary data on Tasmanian spionids…….….
SECTION 2 NEED …………………………………………………. 9
SECTION 3 OBJECTIVES ………………………………………. 9
SECTION 4 METHODS
Quantification by Boccardia knoxi chimney count ……….…
Quantification through expulsion & of spionids from shells.
Estimation of spionid kill efficacy ………………………….
Blister assessment ……………………………………………
4.11 Mud worm reproduction & settlement
4.11.1 Descriptive, larval rearing, reproductive histology……
4.11.2 Timing of spionid settlement……………………….…
4.12 Chemical treatment
4.12.1 Experimental animals & rationale…………………….
4.12.2 In vitro trials………………………………………
4.12.3 Spionid in situ toxicity trials …………………………
4.12.5 Selection of treatment agents…………………………
4.12.6 Follow up treatment experiments…………………….
4.13 Drying treatment
4.13.1 General experimental protocol…………………………
4.13.2 Stock history & method specifics…………………….
4.13.3 Drying & mortality ……………………………………
4.13.4 Drying & long term growth………………………….
FRDC Final Report Page ii
Mudworm control in abalone
4.14 Epidemiology/Risk factor methods
4.14.1 Experimental animals & locations…………………….
4.14.3 Fouling & spionid distribution……………………….
4.14.4 Spirorbid fouling effects……………………………….
4.14.5 Comparison with long term infected stock…………….
4.14.6 Rearing vessel comparison…………………………….
4.14.7 Position in water column………………………………
4.14.8 Abalone species comparison………………………….
4.15 Abalone health I: mortality, growth and condition)
4.15.1 Blister morphology & location……………………….
4.15.2 Measures of abalone condition……………………….
4.15.3 Experimental design: long term treatment…………….
4.16 Abalone health II: physiology and histology
Blister environment & microflora………………….
Ammonia excretion
Starvatopm comparison
SECTION 5 RESULTS & DISCUSSION
Reproductive biology and settlement …………………………
Chemical treatments
Follow up chemical treatment trial ………………….
Air drying treatment
Drying and abalone mortality………………………
Drying and long term growth……………………….
Effect of stock size…………………………………
Spionid settlement and fouling organisms………….
Long term spionid infection comparison………….
Position in water column……………………………
Abalone health I: mortality, growth & condition
Shell blistering & spionid counts………………….
Measures of abalone condition…………………….
Abalone health II: physiology & histology
FRDC Final Report Page iii
Mudworm control in abalone
SECTION 7 FURTHER DEVELOPMENT………………………. 107
SECTION 8 PLANNED OUTCOMES……………………………. 107
APPENDIX 1 (Intellectual property)………….…………………… 119
APPENDIX 3 (Raw data)…………………………………………… 120
FRDC Final Report Page iv
Mudworm control in abalone
NON TECHNICAL SUMMARY
1997/2001 Development of an integrated management program for the control of spionid mud
worms in cultured abalone
PRINCIPAL INVESTIGATOR: Dr Judith Handlinger
Tasmanian Aquaculture and Fisheries Institute
Fish Health Laboratory
Kings Meadows TAS 7249
Telephone: 03 63 365 289
Fax: 03 63 443 085
1. In general, to develop methods for the control of mud worms in farmed abalone, based on the
principles of sustainable aquaculture.
2. To thoroughly investigate the ecology and reproductive biology of spionid mud worms and their
interaction with abalone, through monitoring, field and laboratory experiments.
3. To gather long term data on the efficacy of the chemical treatment(s) (including antifoulants)
throughout the production cycle.
4. To develop a protocol of chemical treatment within the production cycle to optimise the efficiency
of chemical control in relation to abalone survival, growth and marketability, cost and responsible
chemical use.
5. To gather information on the epidemiology of mud worm infestation in relation to ecological and
hydrodynamic characteristics of sites, cage design and deployment and stock husbandry.
6. To use information collected to refine culture methods so as to minimise the level and
consequences of mud worm infestation, preferably without the use of chemical treatments.
FRDC Final Report Page 1
Mudworm control in abalone
NON TECHNICAL SUMMARY:
BACKGROUND AND NEED
Early sea based abalone grow out facilities in Tasmania were severely compromised by stock
mortality in the mid 1990's. Affected stock were found to have large shell blisters caused by marine
worms known as spionid polychaetes or mud worms. Two major species were identified and one of
these, Boccardia knoxi, was especially common at the farm with the greatest stock losses. As a result
of the mud worm infestations many marine farms abandoned attempts at commercial abalone culture
in the sea. The overall objective of this project was therefore to devise strategies by which mud worm
infection could be minimised.
To achieve this objective several research avenues were explored, including:
• Study of mud worm reproductive cycles and strategies with an emphasis on the Boccardia
knoxi species. By this means it was hoped that any seasonal variation in mud worm
reproduction could be identified and exploited to avoid initial infection.
• Ways by which mud worm infection might be treated. This involved testing many anti-
parasitic drugs commonly used in aquaculture and agriculture. Environmental treatments
such as fresh water soaking and air exposure were also tested as a preferred treatment option.
• Other aspects of the research involved examination of stock and environmental
characteristics that might contribute to mud worm infestation. These included stock size and
species, fouling organisms present on abalone, rearing cage design and depth in the water.
• Finally the effect of mud worm infection on abalone health was examined at regular
sampling periods. Mortality rates, growth, flesh weight and blister characteristics were
measured as were changes in abalone physiology. From this work we sought to define
indicators of abalone health that would be useful as diagnostic tools in abalone farming
AVOIDANCE STRATEGIES
Study of mud worm reproduction showed that the Boccardia knoxi species produced larvae
only in the spring months. Larvae were released into the plankton at approximately 0.5 mm and after
a few weeks settled on abalone and burrowed in the shell. Therefore a simple avoidance strategy for
this mud worm species was devised. Abalone placed in the sea in December or later remained free of
this mud worm species for approximately 9 months – allowing a good start to the grow out phase.
Another species of mud worm, Polydora hoplura, was found to settle on abalone in the
spring and summer and infestations could be substantially avoided by December to January stocking
of culture systems. Control of P. hoplura infestations older than 6-9 months is difficult, as local
settlement occurs in all months. Thus it is critical for farms husbandry to ensure stocks are monitored
and treated early.
Testing of mud worm treatments found that several chemicals and drugs were capable of
killing mud worms removed from the abalone shell. However, mud worms present in their shell
burrows were well protected from drug treatments given as a bath. Such treatments had a minimal
effect on mud worms in burrows or were harmful to abalone at doses high enough to kill the worms.
The best treatment for mud worm in abalone was found to be simple air-drying of stock, as has been
traditionally used by oyster growers to fight mud worms. Antifoulant paints supplied by the CRC for
biofouling were tested but as they lacked a suitable application method for abalone no long term
efficacy study of these was conducted. As air drying of abalone was found to be a superior treatment
option to chemical immersion, long term efficacy studies focused on this treatment.
Two to four hours out of water at about 15 to 20 °C was found to reduce mud worm numbers
by up to 90% or more. Treatment was especially effective if abalone had been infected for less than
six months. The older the infections, the longer the exposure time required to kill a significant
proportion of the mud worms. Appropriate conditions to ensure effective drying are considered to be
FRDC Final Report Page 2
Mudworm control in abalone
temperatures >15°C and humidity less than approximately 63%. These conditions are not uncommon
on sunny days in Tasmania outside the period late autumn to early spring. Where shells did not fully
dry treatment was ineffective. Lack of drying was associated with high humidity, larger stock and
severe shell fouling. The difficulty of treating heavily infected stock also emphasises the need to treat
early. Such treatment was considered safe for abalone but had potential to reduce the growth rate of
stock and should therefore be used as a second line of defence rather than the main management tool.
It is recommended that in mud worm susceptible areas abalone be transferred to leases after
December then checked for mud worm infection about a year later and treated then if necessary. A
single treatment would then give the best part of a year's relief from infection, generally allowing
enough time for abalone to reach market size.
Study of mud worm settlement patterns (to achieve objective 5) showed that the mud worm
larvae, especially those of B. knoxi, were attracted to certain fouling organisms sometimes present on
abalone shells. The main risk enhancers were tube building polychaete species such as spirorbids that
did not themselves burrow through the abalone shell but simply colonised the surface. Heavy fouling
with such tube building polychaetes could enhance mud worm settlement by 500%. Transfer of
abalone to sea leases post spring would avoid substantial natural spirorbid settlement.
Stock placed at < 35 mm remained substantially mud worm free even as they grew to
approximately 60 mm, whereas stock placed at about 50 mm became relatively heavily infested.
Thus if there is a requirement to transfer stocks to spionid susceptible sea based sites during
or soon before mud worm dispersal periods the use of smaller and relatively spirorbid free animals
would minimize risk. It would be prudent to place larger abalone after the completion of the spring
settlement period. Heavily fouled hatchery stock should be avoided by farms in mud worm
susceptible areas, but if they are to be used, they should also be placed after the spring settlement
period. While this would minimise the mud worm infection in the first summer, such animals would
provide some enhancement of P. hoplura infestation and would still attract larger numbers of B.
knoxi as well as further tube-building polychaetes in the subsequent spring. Unless animals are close
to harvest after their second summer in the sea, treatment of this infection could well be required, but
could be of limited effectiveness given the larger size and heavy fouling of these animals. Thus stock
growth rates and cost structures for sea based sites will determine which hatchery stock are
acceptable and whether individual farms use these sites for all or part of the grow-out phase.
REARING SYSTEM DESIGN
Rearing container design with respect to mesh area for water exchange had an effect of mud
worm settlement and the progression of the infestation. Cage design should take into account the
need for containment, adequate water exchange (especially in summer) and ease of removal of dead
abalone to maintain water quality, and suitability for air-drying in situ for practicality of treatment.
Restricted water flow restricted mud worm settlement from the spring larval dispersal, but favoured
local spread of P. hoplura at other times. Removal of fouling only after this period but prior to
summer has some advantages with regard to this. Lidded trays provide good drying exposure.
Abalone reared near the bottom (1 m clearance) were found to have greater infection with the P.
hoplura mud worm but not B. knoxi than stock reared higher in the water column (4 m). Thus mud
worm infestation can be minimised by taking into account these risk factors.
In relation to abalone health, mud worm infestation was shown to reduce the growth rate and
abalone with larger mud worm blisters tended to be smaller than less affected stock. Mud worm
affected abalone had less energy reserves than non infected stock. This was consistent with the
observed transfer of effort into shell healing.
Lack of energy reserves was indicated by histological changes to the right kidney and
digestive tubules, particularly pigment accumulation consistent with increased cell turnover; with
depletion of glycogen and protein tissue reserves; reduction in haemolymph glucose and with
FRDC Final Report Page 3
Mudworm control in abalone
increased oxygen consumption. Such changes were likely the result of mobilization and direction of
host resources to shell repair, at least in part, though more direct effects from surface tissue damage
and possibly reduced appetite have not been excluded. Low energy reserves may have been
manifested as reduced growth, and in some instances death. Spionid infestation was also associated
with reduced capacity to regulate potassium and in severely impacted abalone with significantly
lowered circulating haemocyte counts. Similar low levels of haemocyte counts were seen in "runted"
stocks and animals starved experimentally for 3 months, suggesting lack of haemocyte production
may contribute to this. Haemocyte counts are considered potentially useful as a general indicator of
health status.
The widespread mortality seen in the mid 1990's was not repeated in field studies from August
1998 to January 2001. Mud worm settlement levels during field studies from 1998-2001 were
generally very low compared to those observed in abalone surviving the original outbreaks of 1995
and 1996. This suggests mud worm infection of farmed abalone may not be a consistent problem for
farmers in the future but that monitoring of stocks will be required. The research has shown that mud
worm infestation can be managed by avoidance strategies in the first instance and treatment if
The major knowledge outputs are that mud worm infestations can be substantially avoided in
the first instance and treated if necessary. The major project outcome from this knowledge and
a new sense of perspective regarding the risk of severe infestation has been a rise in investor
confidence leading to renewed interest in sea based abalone farming. This has contributed to the
development of at least three new sea based abalone farms in Tasmania. Project findings have
been made available directly to industry and specific discussions held on risk minimisation with
some individual farms.
KEYWORDS: abalone, mud worm, spionid, aquaculture, parasitism, treatment.
FRDC Final Report Page 4
Mudworm control in abalone
The research was funded in the first year by the Cooperative Research Centre for
Aquaculture and for the remainder of the project by the FRDC Abalone Aquaculture
Subprogram. Within the abalone culture industry we wish to acknowledge the financial and
general support of the Tasmanian Abalone Growers Association and the contribution of all
abalone farms involved, particularly that of Huon Aquaculture Company P/L and Aquatas
P/L who provided the major study areas, staff and logistical support. Most of the laboratory
work was conducted at the Fish Health Unit's laboratories within the Tasmanian Department
of Primary Industries, Water and Environment (DPIWE) Mt. Pleasant Laboratories. The
University of Tasmania Aquaculture School also provided facilities for some experiments.
Statistical support was provided by DPIWE statistician Bruce McCorkell.
A considerable proportion of this work comprised the PhD studies of Mark Lleonart, whose
thesis has been submitted and accepted.
FRDC Final Report Page 5
Mudworm control in abalone
1.1 General Introduction
Abalone culture in Australia commenced in the mid 1980's with land based farms in
Tasmania and South Australia. The primary culture species were the blacklip abalone Haliotis
rubra Leach and the greenlip abalone H. laevigata Donovan. In the mid 1990's there were
five sea based experimental or pilot scale farms in southern Tasmania. By 1996 these farms
had experienced stock mortality levels in excess of 50% which were associated with blisters
to the inside of the abalone shell. Both greenlip and blacklip abalone were affected as were
hybrids of the two species. Preliminary investigation showed that two-spionid polychaete
"mud worm" species were present within the blisters of infested abalone. Mud worms are
noted pests of farmed bivalve molluscs and consequently considerable research exists on their
impact on this group (Blake and Evans 1972, Lauckner 1983).
The spionid species present in the shells of live and deceased abalone were identified
as Polydora hoplura Claparede and Boccardia knoxi Rainer. The former species was
previously recorded from Tasmanian farmed and wild oysters (Wilson et al., 1993) but B.
knoxi was only previously known from New Zealand (Rainer 1973, Read 1975). This species
was the dominant spionid at the culture facility with the greatest number of infested stock
and there was some concern that B. knoxi may have been an introduced and particularly
destructive mud worm.
By November 1997 when this study commenced, some marine farms in southern
Tasmania had abandoned abalone culture trials or relocated facilities and all farms had
discontinued transfers of new stock to their leases. It was apparent that a loss of confidence in
sea based abalone farming had occurred and strategies to minimize the economic impact of
spionid infestation would be required before further investment could occur.
1.2 General background: spionid biology and impacts
Mud worms belong to the family Spionidae, one of the largest families of marine
annelid worms in the class Polychaeta. The family is very common in all marine
environments and its members include free-living forms in sand and mud as well as species
that build permanent burrows in soft substrates (Fauchald 1977). Several genera of spionids,
including Boccardia and Polydora, are capable of boring in calcareous substrates including
the shells of molluscs. Boring is achieved by the production of acid secretions (Zottoli and
Carriker 1974, Almeida et al. 1996). The name "mud worm" may derive from the often-
muddy appearance of blisters formed in molluscs as a result of burrowing activity, though
large blisters in these abalone were often filled with clear fluid.
Because of the economic importance of oyster cultivation world-wide, there have
been many studies on spionid impacts (see reviews by Blake and Evans 1972, Skeel 1979,
Lauckner 1983, Handley 1997). In Australia, oyster culture industries have been severely
impacted in the past. Early mud worm infestations were investigated by Whitelegge (1890)
and from about 1870 in NSW were so severe that the industry changed from sub-tidal
dredging to the present intertidal culture system (Smith 1984, Nell and Smith 1988). By
about 1900 mud worm infestation in Queensland had devastated sub-tidal production in that
state (Smith 1982, Nell and Smith 1988). Potential treatment of spionid infestation in oysters
has been previously investigated (Whitelegge 1890, Korringa 1952, Mackenzie and Shearer
1959, Bailey-Brock and Ringwood 1982, Nel et al. 1996). The species most often associated
with damage to commercially important bivalve species are P. ciliata Johnston, P. hoplura
and P. websteri Hartman (Blake and Evans, 1972).
FRDC Final Report Page 6
Mudworm control in abalone
There is considerably less literature on the incidence and effects of spionids in
abalone. Mud worms have been reported from wild haliotid species by: Sinclair (1963),
Shepherd (1973), Clavier (1989), Horne (1996) and Grindley et al. (1998). In Japan, Kojima
and Imajima (1982) found that more than 10 Polydora per shell significantly decreased the
flesh weight of wild H. diversicolor Reeve. References to mud worm incidence in cultured
abalone have been fewer. Ruck and Cook (1999) note that Polydora is a potential problem in
H. midae and McCormick (1999 pers. comm.) described incidents of severe mud worm
infestation in H. discus hannai.
In addition to impacts on molluscs there have been many studies on the reproduction
of spionids (Wilson 1928, Hopkins 1958, Dorsett 1961, Blake 1969, Anger et al., 1986). A
dichotomy in larval feeding and dispersal modes have been described (Radashevsky 1994)
where spionids either obtain nutrition from yolk supplies (lecithotrophy) growing to a
relatively large size with reduced dispersal capabilities or feed in the plankton
(planktotrophy) and potentially disperse widely. These variations in spionid reproductive
strategies may in part explain how different spionids impact on molluscs to differing levels.
1.3 The original Tasmanian abalone mortality episode
During the period 1995-1997 various abalone culture facilities within Tasmania
contacted the State Government Fish Health Laboratory to report cumulative mortality levels
of up to 50% or more associated with mud worm blisters. Heavily blistered abalone were
examined from four sea based grow out farms in the south of the state and similarly infested
scallops from a further farm. Mortality at one of the southern sea farms was in excess of 95%,
involving the loss of more than 30 000 animals by late 1997 (O'Brien 2001, pers. comm.).
Hindrum (1996) reported approximate 40% mortality between November 1995 and April
1996 at one site. Infested abalone at the southern farms, mainly located in the
D'Entrecasteaux Channel, showed the presence of B. knoxi and P. hoplura but no attempt was
made at the time to quantify spionid numbers until late 1997. Stock from three of the southern
farms were made available for study. Two of the farms (Huon Aquaculture Company Pty Ltd,
and Aquatas Pty Ltd) subsequently became study sites for this research. A small number of
remnant stock from Tasmanian Tiger Abalone were made available for study. Mud worm
associated mortality was also reported from a sea-based farm outside the south of the state
and the same two-spionid species recovered from blisters. A further history of mud worm
associated mortality was reported in remnant 5-6 year old, long term infested stocks at an east
coast land based farm. Samples from this source revealed P. hoplura but not B. knoxi was
present in severe blisters. Samples obtained from a land-based farm in South Australia also
showed solely P. hoplura infestation in blistered older stock with a history of mortality.
Some mortality records for the period 1994 to April 1996 were available for Huon
Aquaculture Company stock (Appendix 3, 1A) and showed a high death rate during the
austral summer 1995/1996. Infested stock from this source examined in 1996 had extensive
blister damage that was associated with almost exclusively
B. knoxi infestation. The ventral surface of many shells was characterized by thick, soft,
blisters devoid of nacre and apparently failing to heal. By 1997 approximately 1000 shells of
dead and mud worm infested live stock remained and these were made available for
experiments on spionid treatment options (Sections 5.2 and 5.3). A subjective shell damage
assessment was made on a sample of these abalone (Appendix 3, 1B) using the method
described in Section 4.9. An estimate of spionid infestation made using the method developed
by late 1997 (Section 4.6) found a mean B. knoxi count of 36.5 (SD=19, n=40). Mean
percentage flesh weight of remnant heavily blistered stock from Tasmanian Tiger Abalone
was 52.6% (SD = 3.1%, n=10).
FRDC Final Report Page 7
Mudworm control in abalone
1.4 Previous and preliminary data on spionids in Tasmanian molluscs
In addition to abalone, mortality episodes involving spionid blisters in farmed
scallops and Pacific oysters Crassostrea gigas Thunberg were also reported to Tasmanian
aquaculture health authorities in the mid 1990's. Annual data on blister prevalence in farmed
oysters was collected by the Fish Health Unit as part of research and surveillance programs
(Appendix 3, 1C). These data indicate that blister rates for oysters may vary considerably
year to year. Additionally, the surveillance program showed the southern region of the state
where affected abalone farms were also located had consistently higher levels of oyster
blistering than other regions of the state (Unpublished DPIWE Fish Health Unit data). Wilson
et al. (1993) found 3 species of spionids in Tasmanian oysters. In order of prevalence these
were P. websteri, P. hoplura and B. chilensis Blake and Woodwick. Although B. knoxi was
not found in the survey by Wilson et al. (1993) its presence at that time cannot be ruled out
since most oysters surveyed were intertidal and B. knoxi appears to have a sub-tidal
distribution in New Zealand (Handley 1997).
Following the identification of B. knoxi in cultured abalone stock Tasmanian
aquaculture health authorities commenced sampling of wild abalone populations. Surveys
showed the presence of B. knoxi in wild stocks from the south, south-east, and east coasts but
not in a small number of samples from the north-west (DPIWE unpublished data). No mud
worm associated mortality from wild stocks has been reported. Re-examination of shells from
wild abalone collected by abalone fishery research staff at DPIWE Taroona Laboratories over
a number of years showed that shell damage in wild stocks was common (Appendix 3, 1D).
Most of the damage was attributed to spionid polychaetes but boring sponge damage was also
present in many shells. Anecdotal evidence from abalone divers and processors suggests that
there are populations of stunted abalone with shells considered too damaged to be used for the
jewelry trade. These are typically located in very sheltered area such as the lees of islands and
peninsulas. Analysis of samples from such areas present in the shell collection at the Taroona
Laboratories indicated they had higher rates of shell damage than larger abalone (Appendix 3,
1.5 Background: Project development
The Tasmanian abalone farming industry, through the growers association (TAGA)
sought support for research into mud worm problems as a result of the initial outbreaks
described above. In the first instance TAGA, in collaboration with the Tasmanian
Department of Primary Industries, Water and Environment (DPIWE) commenced a program
to identify mud worm species present and their distribution in farmed and wild stocks around
the state.The Cooperative Research Centre (CRC) for Aquaculture provided funding for a 12
month study into development of chemical control methods for spionids in farmed abalone.
This project (CRC project number A.2. 6) commenced in November 1997. The FRDC
funded component of the research (98/307) commenced November 1998 with the aim of
producing an integrated approach to spionid management. Thus the biology, including
reproduction of the mud worms was studied, as was the epidemiology of infestation and the
effects on abalone health.
Abalone culture is an expanding industry with commercial farming under way in all
the southern states. To date most farms are land based facilities but sea based culture offers
FRDC Final Report Page 8
Mudworm control in abalone
lower capital investment and provides a broader range of industry options. There are
currently sea based farms in Tasmania and Victoria. Although land based farms are not
immune from mud worm infestation the most serious problems have occurred in sea based
facilities in southern Tasmania. Advances in mud worm management strategies are essential
if sea based farming in Tasmania is to be viable and will benefit the abalone farming sector
1. In general, to develop methods for the control of mud worms in farmed abalone, based on
the principles of sustainable aquaculture.
2. To thoroughly investigate the ecology and reproductive biology of spionid mud worms
and their interaction with abalone, through monitoring, field and laboratory experiments.
3. To gather long term data on the efficacy of the chemical treatment(s) (including
antifoulants) throughout the production cycle.
Note: that air drying of abalone was found to be a superior treatment option to chemical
immersion so long term efficacy focused on this. Antifoulant paints supplied by the CRC for
biofouling were tested but lacked a suitable application method for abalone. Thus long term
testing was not conducted.
4. To develop a protocol of chemical treatment within the production cycle to optimise the
efficiency of chemical control in relation to abalone survival, growth and marketability, cost
and responsible chemical use.
Note: as above, air drying rather than a chemical treatment was used.
5. To gather information on the epidemiology of mud worm infestation in relation to
ecological and hydrodynamic characteristics of sites, cage design and deployment and stock
6. To use information collected to refine culture methods so as to minimise the level and
consequences of mud worm infestation, preferably without the use of chemical treatments.
FRDC Final Report Page 9
Mudworm control in abalone
4. METHODS
4.1 Experimental Animals
The majority of abalone used in the research were blacklip (H. rubra) stock
from a land based culture facility located on the east coast of Tasmania (farm 1).
Many abalone used in experiments were from a year class spawned in the summer of
1997/1998. These were approximately 20 mm shell length when first transfers to
study sites were made in spring 1998, growing to 40 mm by late 1999 when the last
intake of this age cohort was used. The subsequent year class (summer 1998/1999
spawning) was used in December 1999 and 2000. No evidence of any mud worm
species was seen in these year class stocks during the research.
Another important experimental group from this farm was a remnant
population approximately three years' old in mid 1998. These abalone had low
incidence (< 5% of population) along with a low severity (generally < 3 worms) of B.
knoxi infection. This group was used in some treatment experiments as described in
Sections 4 and 5 using the presence of characteristic B. knoxi chimneys to select for
infested animals. Further abalone from the group were used in some transfers to study
sites selecting B. knoxi negative animals on the basis of lack of chimneys and drying
them to kill any mud worms present (Section 5.3).
Where abalone other than blacklip stock were used in specific experiments, or
animals were obtained from culture facilities other than farm 1, this is noted in the
appropriate section of the report.
4.2 Study Sites
A field study site was located in North West Bay at Simmonds Point (DPIWE
lease number 154) and owned by Aquatas P/L, (Figures 4.1 and 4.2). The site is 17-
18 m deep, with sediments described as ranging from very fine dark brown sand to
muddy sands dark grey in colour. The current speed is 20 cm.s-1 and the tidal range
1.2 m (from D'Entrecasteaux Channel Marine Farming Development Plans for
Tasmania, February 1997). Aquatas was predominantly involved in salmon farming
during the course of the research but had investigated sea based abalone farming in
the early and mid 1990's. This previous history of mud worm infestation prompted
the use of the site for the current research. Abalone were originally housed in culture
vessels hung in 3-4 m of water from a barge on the lease but later moved to a long line
200 m north of the barge.
A second southern Tasmanian study site was located in the Huon River at
Hideaway Bay (DPIWE Farm Number 93) and belonged to the Huon Aquaculture
Company P/L (hereafter referred to as Huon Aquaculture). (Figures 2.1 and 2.2). The
environment is estuarine with a pH range of 7 - 8 and variable salinity. Averagemonthly temperature range is 11-19 °C. The study site was located in that part of the
lease (MF 93) where the depth ranges from 8-12 m and current speed ranges from 1-
20 cm.s-1. The sediments are fine sands (DPIWE Lease Monitoring Baseline data
provided by Huon Aquaculture Company P/L). During the research this was
predominantly a salmon farming company but with an interest in shellfish including
Pacific oysters and abalone. The site was chosen because of its past history of mud
worm infestation in abalone. Abalone were housed in culture vessels suspended at a
depth of 3-4 m from the collars of an empty salmon cage moored in 10-12m of water.
FRDC Final Report Page 10
Mudworm control in abalone
A third sea-based study site was located on the east coast of Tasmania (Figure
4.1). This is referred to as Site 3, or Site 3 (East Coast). Stock were reared on a
commercial scale at this site, suspended from long-lines in 20 m of water.
4.3 Holding Conditions
Field Trials
Various types of rearing vessels were used to contain abalone during the
research. When mud worm infestations were originally recorded, abalone at several
culture facilities were held in vessels constructed from 250 mm PVC pipe fitted with
6 mm mesh ends. These were referred to as "tubes" (Figure 4.3A) and measured 1500
mm in length. Monthly intakes of "clean" abalone were housed in tubes from August
1998 to November 1998. Most abalone were eventually removed from tubes and
housed in other types of rearing containers described below. This was because there
were insufficient tubes to house all the abalone required for the research and because
tubes were relatively expensive to manufacture and heavy to handle.
Nearly all intakes of abalone subsequent to summer 1998/99 were housed in
"basket type" culture vessels. These were modified polyethylene laundry baskets
fitted with oyster mesh and plastic inserts to increase mesh free substrate area (Figure
4.3B). The baskets were approximately cylindrical, 800 mm high with a diameter of400 mm. A third type of rearing vessel was the Aquatek Aquatray (Figure 4.3C)
measuring 900 mm on each side and 10 mm deep. Bases of these were solid with a 12
mm mesh lid and sides. Abalone maintained in the long term were transferred to these
from tubes and baskets in late summer 1999 and 2000 respectively. This was to group
abalone with similar levels of mud worm infection together in a smaller number of
rearing vessels to reduce maintenance time.
Abalone held at the southern study sites were generally treated in the same
way as when the original mud worm problems arose in the mid to late 1990's.
Feeding was weekly or bimonthly using Adam and Amos (Mt. Barker, S. Australia)
sea cage formulated abalone food.
Laboratory Holding Conditions
Abalone were moved from field sites to the Animal Health Laboratory (Mt.
Pleasant Laboratories, Department of Primary Industry Water and Environment,
Launceston, Tasmania) for analysis. Transportation was in water to minimise stress to
abalone and polychaetes. Abalone were held for no more than two weeks in a 650 l
recirculating system. This consisted of a fibre glass tank with a conical base and a 400
l sump containing 50 l of "bioballs" as a biological filter medium. The system was
aerated and temperature control maintained by use of a 250 W aquarium heater and/or
reverse cycle air conditioning. Water temperature was maintained at that of the studysites (±2 °C). Ammonia and nitrite measurements were made on occasion using
Aquasonic (Ingleburn, NSW) aquarium test kits and pH was measured using Sigma
(range 4.5-10) indicator paper. Salinity was measured using a Shibuya S-10
refractometer. Ten to twenty percent of tank volume was exchanged on a weekly
basis. Abalone samples were separated within the system by placement in mesh bags
or plastic aquaria with mesh lids through which air stones were fitted.
FRDC Final Report Page 11
Mudworm control in abalone
Figure 4.1 Map of Tasmania showing study sites and source farms
FRDC Final Report Page 12
Mudworm control in abalone
Figure 4.2 Map of D'Entrecasteaux Channel, southern Tasmania showing study
sites at Huon Aquaculture Company P/L and Aquatas P/L.
FRDC Final Report Page 13
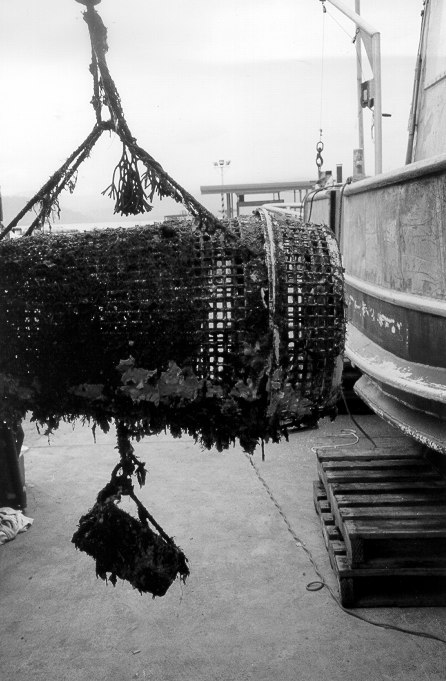
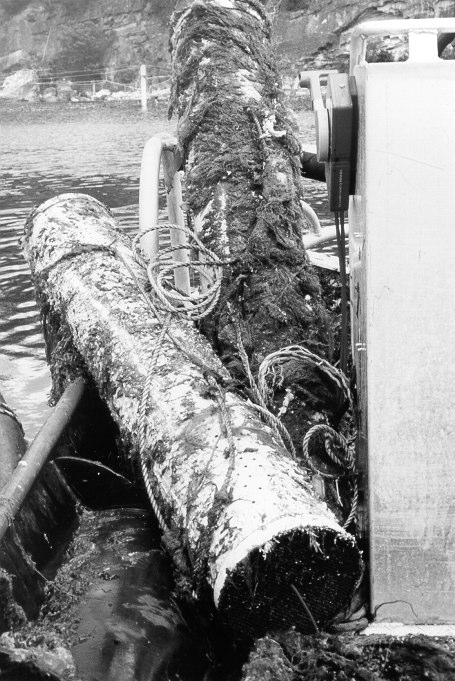
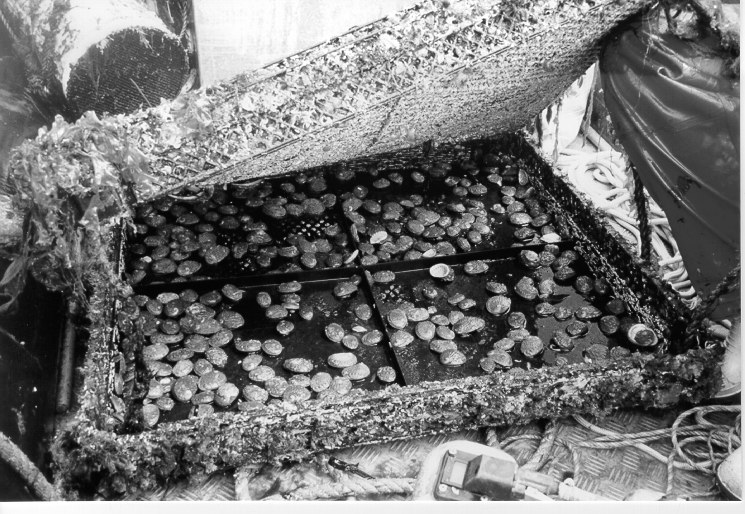
Mudworm control in abalone
A. Tube B. Basket
Figure 4.3 Abalone containment vessels
FRDC Final Report Page 14
Mudworm control in abalone
4.4 Speciation of polychaetes
Spionid species were identified on the basis of fifth setiger, prostomium, and
pygidium morphology, gill distribution, eyespots, colour and size. Descriptions of
species encountered in the research are given in Rainer 1973, Read 1975, Blake and
Kudenov 1978. Presumptive identifications of B. knoxi and P. hoplura were
confirmed by Dr. Rainer who first described B. knoxi (Rainer1973). Initial
identification of Boccardia proboscidea Hartman was made by Dr. Geoff Read. Non-
spionid polychaetes found on or within the shell were not speciated but were included
in total polychaete counts where appropriate. Calcareous tube building polychaetes,
such as spirorbids and Pomatoceros sp. (Figure 4.4), both usually grouped in the
family Serpulidae (Fauchald, 1977) were not included in polychaete counts.
4.5 Quantification by Boccardia knoxi chimney count
Preliminary investigation of mud worm infestation showed that B. knoxi had a
distinctive transparent tube or chimney at the burrow entrance (Figure 4.5). Such
chimneys were typically 2-10 mm in length and produced approximately a month
after B. knoxi settlement.
While other Tasmanian mud worm species at times produced a burrow entrance
structure, the B. knoxi chimney was considered distinctive enough to be a useful
diagnostic tool. Chimneys were found to survive the death of the worm by many
months in the field, and for at least eight months in a laboratory test (M. Lleonart
unpublished data). Remnant, heavily infested abalone from mortality episodes in 1997
were found to have a 90% occupancy rate of live B. knoxi on the basis of chimney
counts (mean B. knoxi count 36.5, SD=19, n=40 shells). This fell to 55% for shells of
abalone dead for several months, with a mean live B. knoxi count of 23 (SD=15.5,
n=40). Thus chimney counts allowed potential estimation of present and/or past B.
knoxi numbers. Severe shell fouling interfered with accurate assessment of chimneys.
Caceres- Martinez (1999) also found that spionid polychaetes survived the death of
the molluscan host.
4.6 Quantification through expulsion of spionids from shells
Mud worm infestation levels were quantified by the use of chemical vermifuges
to expel polychaetes from shell burrows or the surface of the shell. This was a mixture
of 100 PPM 0-dichlorobenzene and 500 PPM phenol in seawater and was based on
the methods of Mackenzie and Shearer (1959) and Handley (1997). Abalone were
shucked and shells placed in either 50 ml or 400 ml pots depending on size. Pots were
at least 60% full of vermifuge solution. When mud worm colonization was considered
relatively recent, shells were immersed in vermifuge in the morning and removed in
the late afternoon. Longer time periods tended to kill small polychaetes and post
larvae rendering identification difficult. Shells with obvious signs of mud worm
infestation were exposed to vermifuge solution overnight at a minimum temperatureof 15° C. After vermifuge exposure the contents of pots were drained through 90 µm
sieves and worms rinsed to petri dishes for examination with a dissecting microscope.
In addition, the surface of the abalone shell was examined under low power
microscopy to include any polychaetes only partly expelled from burrows. On
occasion mud worm blisters were dissected to check for the presence of polychaete
eggs and retained worms.
FRDC Final Report Page 15
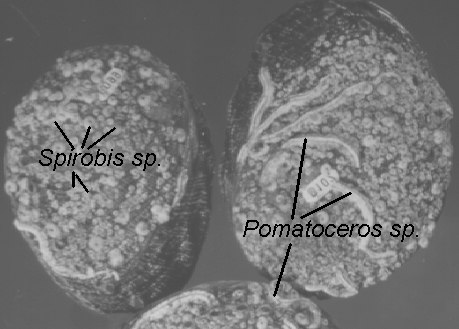
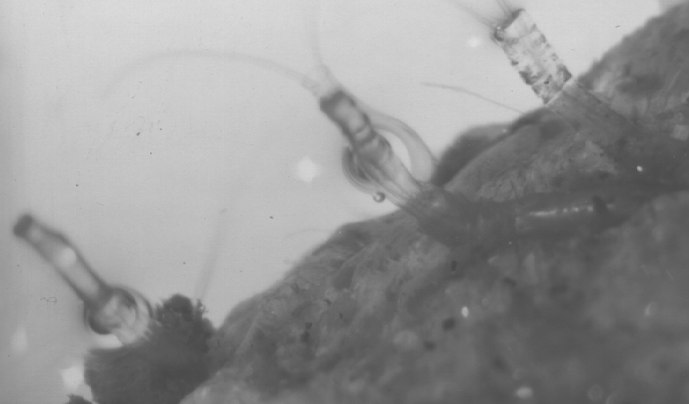
Mudworm control in abalone
Figure 4.4 Calcareous tube building polychaetes fouling abalone shells
Figure 4.5 Boccardia knoxi worms inside characteristic chimney tubes
FRDC Final Report Page 16
Mudworm control in abalone
4.7 Estimation of spionid kill efficacy
The distinctive B. knoxi chimney allowed an estimate of treatment efficacy by
comparison of chimney counts to worm numbers post treatment. This was termed the
estimated individual percentage (EI%) kill and calculated:
EI%Kill = No. chimneys - No. live B. knoxi • 100
Comparison of surviving worms of other species was calculated by comparison of
treatment and control group means. This is referred to as the group mean comparison
(GMC) percentage kill. Kill data for B. knoxi was calculated by the latter method
when excessive fouling rendered chimney counts difficult and when untreated control
group data indicated many B. knoxi burrows no longer contained live worms.
4.8 Growth
Length of abalone was measured to the nearest 0.1 mm using callipers and
weight measured after towelling dry to the nearest 0.1 g. Where abalone were tagged
this was done with soft plastic tags (Hallprint, South Australia) fixed to dried shells
with fast drying adhesive. This allowed mean individual growth to be calculated.
Specific Growth Rate (SGR) which gives a measure of growth independent on
abalone size was calculated as the difference between the natural logarithms of the
initial and final measures (in mm or g), divided by time and multiplied by 100.
4.9 Blister Assessment
The extent of mud worm blistering was assessed by two methods: a subjective
score and an estimate of percentage blister cover. The subjective score was based on
approximate area of blister damage, depth and degree of apparent shell healing.
Scores of 0,1,2 and 3 were possible and the system referred to as the subjective shell
damage rating (SSDR). Scores of "0" indicated an absence of shell blistering. Scores
of "1" indicated light damage, typically blistering to less than 10% of the shell area
with blisters characteristically flat and yellow or alternatively well healed with a thick
coating of shell nacre over them. An SSDR of "2" indicated damage to 10-25% of the
shell area with blister characteristics like that of rating "1" near the upper end of the
affected area range, or alternatively raised brown to black blisters over a lesser area of
the shell. Shells with raised brown to black blisters occupying at least 25-30% of shell
area often with deformity around the apex were assigned the maximum SSDR of "3"
This rating was based on shells observed in the original mortality episodes that lead to
the research. Examples of shell damage ratings are given in Figure 4.6.
Assessment of percentage blister coverage was made by tracing blisters and
shell perimeter on flexible plastic with a printed grid. Areas of blisters and total shell
were then calculated by counting squares and percentage blister coverage calculated.
Blister coverage was further assessed by classification into "active" and "healed"
blisters (Figure 4. 6C). Healed blisters were described as those blisters with a
substantial amount of shell nacre deposited over them and which in time could
become virtually indistinguishable from the normal shell. Active blisters included
those displaying the yellow colour of early conchiolin deposition through to brown
and black blisters as a result of the "mud" deposits showing through a relatively thin
FRDC Final Report Page 17
Mudworm control in abalone
surface layer. This distinction between blister types was intended to help quantify the
age of blisters and the host response to spionid infestation.
Figure 4.6A SSDR "1" B = Blisters, ST= soft tissue remnant
Figure 4.6B Three shells with SSDR of "2"
Figure 4.6C Three shells with SSDR of "3"A = Active blister, H = Healed Blister
FRDC Final Report Page 18
Mudworm control in abalone
4.10 General Statistical Methods
Statistical analysis was conducted using Genstat 5 software ( 1998 Lawes
Agricultural Trust, Rothamsted Experimental Station). For temporal change
experiments data were subjected to one or two way ANOVA with time, and time and
treatment regime as factors respectively. Assumptions of normality and homogeneity
of variance were checked by examination of the residual plots option in Genstat.
REML (Residual Maximum Likelihood technique) was used instead of ANOVA for
unbalanced data sets (where sample numbers varied between times). Where individual
data sets were transformed this is noted in the methods section of the appropriate
section. Separation of means was performed by the use of the LSD (Least Significant
Difference) function in Genstat.
Spionid count data when the values were low tended to be non- normally
distributed and the non-parametric Mann-Whitney U Test, suitable for such data,
(Sokal and Rohlf, 1995) was used. Non-parametric methods were also used for
analysis of subjective SSDR ordinal data.
The non-parametric or distribution free Kruskall-Wallis test (Zar, 1984) was
preferred to ANOVA when data sets were small and the nature of the underlying
distribution was unknown. Mean separation was performed by the method of Zar
(1984) relating to this test.
4.11 Spionid reproductive biology and settlement
4.11.1 Descriptive methods, including larval rearing and spionid reproductive
Mud worm egg strings were dissected from blisters in cultured abalone and
examined using Olympus dissecting and stage microscopes with camera adapters.
Where required, specimens were relaxed with 10% magnesium chloride.
Spionids were cultured by releasing larvae from capsules at approximately the 5-setiger stage and rearing in 1-l plastic aquaria. Water was filtered to 10 µm and
supplied with cultured microalgae (School of Aquaculture, University of Tasmania –
Launceston) including Isochyrsis galbana, Pavlova lutheri and Tetraselmis sp.
Ambient water temperature was 13-16 °C and larvae were captured on 90 µm sieves
each 3-4 d and relocated to clean aquaria. For reproductive studies presumptive adult
(>10 mm) B. knoxi were relaxed in 10% magnesium chloride, fixed in 10% seawater
formalin and histological sections prepared (section 4.12). Worm sections were
examined at a magnification factor of 100 for the presence of eggs. Eggs weremeasured with an eye piece graticule and the presence of mature eggs, up to 95 µm in
diameter (Read, 1975) recorded.
4.11.2 Timing of spionid settlement
To investigate the annual pattern of spionid settlement, non- mud worm infected
stock from farm 1 was transferred to the sea based study sites at Aquatas and the
Huon Aquaculture Company (section 4.2). This was done monthly: August-January
1998, June- December 1999 and July-December 2000 with 100 abalone in each of 2
replicates per study site. Generally 10 abalone were sampled per replicate the
following month with further samplings every 2-4 months subsequently. Additional
stock present at farms prior to August 1998 was also examined periodically. Abalone
FRDC Final Report Page 19
Mudworm control in abalone
were housed in tube or basket type rearing vessels and maintained as described in
Section 4.3. Abalone at Site 3 (East Coast) (Section 4.2) was surveyed April 1999 and
6 times between April and November 2000. Abalone were transferred to the site
January-February of the sampled year.
Samples were relocated to the Fish Health Laboratory, Launceston in water and
mud worms detached from shells using the chemical vermifuge method (Section 4.6).
Examination of shells exposed for a month showed that spionids present were < 3mm
in length and thus worms of this size present in shells with prior mud worm exposure
were also considered to provide evidence of recent larval settlement. Mud worm
blisters were investigated for the presence of adult worms and their reproductive state
assessed. Spionids were considered to have mature eggs within the body if these
ruptured easily and were released during examination. The presence of extruded egg
strings was recorded and they were examined for the presence of active larvae within
the capsules. As not all samples contained blisters within a given month the absence
of body egg and extruded shell egg/larvae data in results Tables 5.1 and 5.2 does not
confirm the absence of these stages of reproductive development.
4.12 Chemical treatments
4.12.1 Experimental animals and rationale
When these experiments commenced fewer than 1000 heavily infested abalone
remained from the original mid 1990's spionid outbreak. These were made available
for treatment research and continued to die during 1998 as experiments proceeded.
Consequently, initial experimental emphasis was on performance of screening trials to
identify promising treatments while experimental animals remained. Later it was
found that infested shells retained viable mudworms for months after death of abalone
and such "empty shells" were used in many experiments. As infested abalone shells
of any type became scarce further experiments were conducted in vitro using spionid
mudworms, primarily B. knoxi, extracted from infected Pacific oysters. Experiments
on toxicity of potential treatment agents to abalone were conducted on non-infected
healthy stock in the absence of infected stock. Follow up work for the most promising
treatment was conducted on a limited supply of lightly infested stock considered still
4.12.2 In vitro trials of toxicity to spionids
Spionids were sourced from known B. knoxi infected Pacific oysters resident at
the Huon Aquaculture Company. Shells containing presumptive B. knoxi were
determined by the presence of the distinctive transparent "chimney" (section 4.5). The
majority of spionids selected for use were B. knoxi but there was no attempt to
exclude worms of other species from closely related genera.
Mud worms were removed from shells by mechanical destruction with bone
forceps or by the use of chemical vermifuges (section 4.6). Where chemical extraction
was used harvested worms were placed in several changes of fresh seawater then left
for 1-2 d in aerated water. Worms were sorted using a dissecting microscope
selecting substantially intact specimens with normal colour.
During toxicity trials mud worms were housed in 200 ml specimen jars for
initial exposure to treatment chemicals and the duration of the recovery period. No
aeration was supplied and water changes were performed daily. Toxicity trial
recovery periods were up to 3 weeks and worms were not fed. Spionids were
FRDC Final Report Page 20
Mudworm control in abalone
examined grossly at water changes and microscopically once or twice per week.
Specimens were considered dead if no movement could be detected and
decomposition had begun. Generally, chemical treatments were tested at 3 or more
different concentrations with one specimen jar of 5-10 worms used for each
concentration. Exposure times were three hours unless otherwise stated. Ambienttemperature ranged from 15-18 °C.
4.12.3 Spionids in situ toxicity trials
Abalone used in these trials were remnant severely B. knoxi infested animals
from Huon Aquaculture. On going stock deaths indicated the health of experimental
animals was severely compromised. Stock was 40 - 80 mm in length and 4-5 years
old. In excess of 10 distinctive B. knoxi chimneys were present on stock selected for
experiments. Experiments used live infested abalone, infested "empty shells" or a
combination of both as indicated.
Potential treatments were generally tested at 3 different levels of chemical
concentration or exposure times with an untreated control. Ten abalone were used per
treatment level with individual tagging using "supa glue" (Selleys, Padstow, NSW)
and plastic tags (Hallprint, South Australia). During treatment abalone and "empty
shells" were removed to 5-l aquaria outside the recirculating system (section 4.3), and
returned to it after chemical exposure and thorough rinsing.
Aeration was supplied during experimental exposure and afterwards in the
holding tank. Exposure times were generally 3 h and water temperature was 15-18 °C.
Abalone and shells were maintained post treatment for approximately 1 week. This
allowed sufficient time for mud worms and abalone to recover from treatment if they
were capable of doing so. It also allowed sufficient time for dead mud worms to
decompose, assisting in the assessment of surviving worms. Abalone mortality was
recorded and dead animals removed, shucked and the shells replaced in the aquaria.
At the termination of the recovery period surviving mud worms were driven from
their shells by chemical vermifuges (section 4.6) and quantified.
Treatment efficacy was estimated by comparison of group means between
control and treatment groups (section 4.7). Alternatively, where appropriate the
individual kill rate for each shell was calculated by use of the EI%Kill (section 4.7).
4.12.4 Abalone Toxicity Trials
Live infected abalone were not always available for in situ efficacy testing of
potential mud worm treatments. Where such animals were available their health was
considered poor due to the severe extent of mud worm infestation. Thus healthy
abalone were used for toxicity work on promising chemical treatments. This stock
was sourced from farm 1 (section 4.1). Most of the abalone was 40-50 mm, 12-25 g
blacklip between 2 and 3 years old. A second population 18-20 mm, 0.7-1.1 g blacklip
of 10 to 11 months of age was also used on occasion. Toxicity trials were conducted
in the aquaria described previously. Generally 5-10 abalone were used for each
treatment level tested. A reserve population of at least 20 abalone in the recirculatingsystem served as a control. Immersion exposure times were 3 h at 14-16 ° C unless
otherwise stated. After experimental exposure abalone were returned to the
recirculating system until any mortality had apparently ceased. Where no mortality
occurred animals were maintained for 1-3 weeks with formulated commercial abalone
feed provided.
FRDC Final Report Page 21
Mudworm control in abalone
4.12.5 Selection of treatment agents
The experiments conducted on spionid mud worms in vitro, in situ in abalone
(including "empty shells) and toxicity trials conducted on abalone were considered
screening trials. Their intention was to test a wide range of treatment agents so that a
suitable treatment could be found while infected stock remained.
Freshwater exposure has a history of use in oyster culture for spionid treatment
and avoidance and has been assessed by Korringa (1952), Bailey-Brock and
Ringwood (1982) and Tonkin (1997). Death occurs through osmotic shock. As
abalone and mudworms (annelids) are closely related, chemotherapeutic agents
chosen sought to exploit the size differential between mud worms and host.
Potassium permanganate is effective against ectoparasites and external fungal
infections in fish (Cross and Needham, 1988). These authors cite concentration and
exposure times of 10 PPM for 30-60 min, 25 PPM for 15 min or 4 PPM permanently.
Kirby and Baker (1995) note that potassium permanganate has been used in the past
to expel earthworms (which like polychaetes are annelids) from grass sporting
surfaces. Accordingly mud worm toxicity trials in vitro and in situ, and abalone
toxicity experiments were conducted in the range between 2 and 50 mg.l-1.
Gentian violet is listed by the Merck Index as a vermicide. Toxicity trials on mud
worms in vitro were initially conducted at between 10 and 100 mg.l-1 to find the
effective range. Two representatives from the benzimidazole class of drugs were
tested, these being mebendazole and fenbendazole. Such drugs are commonly used in
terrestrial animal production against nematodes, flukes and tapeworms. Cross and
Needham (1988) indicate fenbendazole will kill nematodes in fish when added to
feed. The two drugs tested did not dissolve effectively in water and treatment
concentrations may be considered suspensions rather than solutions. Concentration
ranges of between 25 and 500 mg.l-1 were used for mud worm toxicity trials in vitro
and in situ, and abalone toxicity experiments.
Levamisole belongs to the imidazothiazole class of drugs used in various forms
in terrestrial animal production against nematodes in particular. Its use here against a
parasite of an aquatic animal is perhaps novel but was considered worth investigating.
This drug was tested in the range 0.32 to 640 mg.l-1 on mud worms and abalone.
Malachite green has been used to treat fungal and protozoan infection in fin
fish at 2 PPM for 30-60 minutes (Cross and Needham, 1988) and filamentous bacteria
in prawns at 10 mg.l-1 (Owens et al., 1988). It is widely used in aquarium fish
medications. Concentration ranges of 1, 5, 10 and 20 mg.l-1 were used for toxicity
trials on mud worms and/or abalone.
Trichlorofon is an organophosphate, a class of drugs used against nematodes and
tapeworms in terrestrial production animals. Neguvon, the active ingredient of which
is trichlorofon, used at 300 PPM for 15-60 min will kill salmon lice in farmed salmon
(Brandal and Egidius, 1979). Langdon (1990) states that trichlorofon is effective
against skin and gill flukes, Argulus sp. and copepods at 0.5 mg.l-1 in sea water. The
drug was used in the range 0.1 to 1000 mg.l-1 for in vitro mud worm toxicity trials and
10-500 mg.l-1 in abalone toxicity trials. Praziquantel concentration of 10 mg.l-1 for 3 h
is effective against monogenean flukes in fish (Cross and Needham 1988, Langdon
1990). Lester (1988) lists it as an effective agent against tapeworms when used in
food. A range of doses up to 100 mg.l-1 was tested against mud worms in vitro.
Hydrogen peroxide is an oxidising agent used in aquaculture for disinfection
purposes. Exposure ranges of 50 to 1000 PPM were used for toxicity trials with mud
worms in vitro and/or abalone.
FRDC Final Report Page 22
Mudworm control in abalone
Formalin is widely used in aquarium fish medications, often in combination
with malachite green. Cross and Needham (1988) list concentration rates of 167-250
PPM (60 min) as suitable for treating parasites of fin fish under certain conditions.
Concentrations of 50-200 mg.l-1 were used for toxicity trials against mud worms and
abalone.Ivermectin belongs to the macrolide endectocide class of drugs and is used in
terrestrial animal production against nematodes and arthropods. Wislocki et al. (1989)
present toxicity data for the related drug abamectin, stating 96 h LC50 values of
430,153 and 3.2 PPB for Eastern oysters (Crassostrea virginica), blue crabs
(Callinectes sapidus Rathbun) and rainbow trout (Oncorhynchus mykiss)
respectively. As with levamisole the use of ivermectin against an aquatic parasite is
some what novel. Ivermectin was tested against mud worms in vitro and/or abalone at
between 0.004 and 0.4 mg.l-1.
It was an aim of the screening process to test representatives of various drug
classes. Accordingly, Exelpet , "All-Wormer for Dogs" (Wyong, NSW, 2259) tablets
containing febantel, pyrantel embonate and praziquantel were tested. This
combination is intended to treat a variety of nematodes and tapeworms found in dogs.
Febantel and pyrantel embonate belong to the probenzimidazole and pyrimidine
groups of drugs respectively, which had not been previously tested. Praziquantel, also
present, had been tested previously and found ineffective. Interaction effects between
the three drugs would require investigation if early results were promising. The
concentration range was 25, 14.4 and 5 mg.l-1 respectively of each drug through to
five times these doses for mud worms in vitro. Abalone mortality was assessed at the
higher level only.
Metronidazole is effective against external protozoa (Cross and Needham, 1988)
at 25 mg.l-1 in fish. This and the related drug dimetronidazole were tested in the range
five to 200 mg.l-1 and 20 to 500 mg.l-1 respectively against spionids in vitro.
Methylene Blue is widely used in over the counter medicines used for the
aquarium fish trade. It is effective against protozoan and fungal infections in fish at 3
PPM (Cross and Needham, 1988) and against protozoans (ciliates) in prawns at 8
PPM (Owens et al., 1988). Mud worm toxicity experiments were conducted in situ at
1, 5 and 10 mg.l-1 and in vitro at 20 – 200 mg.l-1.
4.12.6 Follow up experiments performed on lightly infected stock
Further experiments using lightly infected stock considered still treatable, were
performed for treatment options showing most promise from the experiments
conducted in vitro, in situ with severely infected stock, and from abalone toxicity
trials. Experimental animals were chosen from a small pool of lightly infected,
B. knoxi positive, 40-50 mm abalone on the basis of visible chimney structures.
Animals were individually tagged as described previously.
A chemotherapeutic bath experiment consisted of four treatment groups,
including an untreated control, each with two replicates of 10 abalone. The treatmentswere 3.5 h immersions at 15 °C in one of the three following chemicals: gentian violet
5 mg.l-1, mebendazole 200 mg.l-1 or potassium permanganate 15 mg.l-1. The untreatedcontrol group was maintained in a recirculating system at 15 °C.
Following treatment abalone were maintained in the recirculating system for 1week at 15°C with out food and shells processed as described previously to quantify
polychaete infestation. Data were analysed using chi-square analysis. Chi-square
analysis was performed by comparing the number of presumed dead and live worms
FRDC Final Report Page 23
Mudworm control in abalone
recovered at the end of the trial. Dead worms were calculated as number of chimneys
minus number of live worms recovered for each shell.
4.13 Air drying treatment
4.13.1 General experimental protocol: air drying trials
Experimental animals were obtained from 1 of 4 commercial abalone culture
facilities. Where treatment experiments were conducted in the laboratory stock was
transported in water as were animals treated on site then assessed in the laboratory.
Treatment group abalone were removed from culture vessels and exposed for air
drying on a suitable substrate such as plastic sheet. Where replication was used
separate substrates were provided for each group. Temperature was measured at half-
hour intervals as was humidity using a wet and dry bulb hygrometer (Masons type,
Zeal, London). In some experiments abalone were individually tagged (section 4.8).
Untreated control animals were returned to water as soon as possible after tagging.
Replicates were kept in separate mesh bags or plastic aquaria.
Following treatment, air exposed and control abalone were maintained in therecirculating system (section 4.3) for one week at 15-16°C with out food. This
allowed time for dead mud worms to decompose clarifying assessment of surviving
mud worms. Abalone were subsequently shucked and shells placed individually in a
mixture of 500 PPM phenol and 100 PPM 0-dichlorobenzene (vermifuge solution) in
seawater over night to expel worms from shells (section 4.6). All spionid mud worms
were speciated but other polychaetes were grouped as "others" and may contribute to
total polychaete data in some experiments. Fouling polychaetes such as Pomatoceros
sp. and Spirorbids were excluded from counts. Treatment efficacy data was calculated
by the estimated individual percentage kill (EI%Kill), (section 4.7) where possible
and otherwise by group mean comparison (GMC).
4.13.2 Stock history and method specifics
Trial 1. Treatment of remnant severely infested abalone
Blacklip abalone present at Huon Aquaculture since 1994 or 1995 and severely
B. knoxi infested were assigned to treatment groups of 3, 5 and 8 h air exposure.
Abalone were 50-70 mm in length with SSDR scores of 2-3 (section 4.9) and blister
coverage to > 25 % of shell area. Ten shells were assigned to each air exposure time,
the majority of which were live abalone. Five shells including one live abalone were
used for an untreated control.
Abalone were placed in plastic aquaria and located in light shade outside at the
Fish Health Laboratory. Air temperature inside aquaria ranged from 21-24° C during
exposure which was staggered so that all aquaria were returned to water at the same
time. The trial was conducted in late November 1997.
Trial 2. Treatment of stock infested with B. knoxi < eight months, four hours at 24 °C
Blacklip abalone approximately 3 years old and positive for B. knoxi chimneys
were selected from available stocks at farm 1. Forty animals were chosen a sample of
which measured 44.6 mm (SD=3.6 mm, n=15). The infection level was considered
FRDC Final Report Page 24
Mudworm control in abalone
low with generally 1-3 chimneys per abalone. The infestation period was estimated at
approximately eight months.
Twenty abalone were assigned to each of treatment and control groups andtagged. The treatment group was exposed to air for 4 h at 24 ± 1 °C using reverse
cycle air conditioning. The humidity was measured by later recreation of the
conditions at 46%.
Trial 3. Treatment of B. knoxi infected stock at different temperatures
A total of 105 B. knoxi positive blacklip abalone, mean length 47.1 mm (SD =
3.9 mm, n = 40) were obtained from farm 1. This group was considered lightly
infected with typically 1-3 chimneys seen by stereo microscopy. Larval settlement
was believed to have occurred about 8 months previously. Abalone were individually
Twenty-one abalone in each of 4 treatment groups were exposed to air for 4 h.
Air temperatures were: 15, 18, 21 and 24 °C and were controlled using reverse cycle
air conditioning or column heating. Humidity values were: 60, 62, 53 and 71% in
ascending temperature order. An untreated control group was maintained in therecirculating system at 15°C.
Trial 4. Treatment of B. knoxi infected abalone at different exposure times
Eighty B. knoxi positive abalone were selected from the same source as Trials 2
and 3 above and tagged. There were 5 treatment groups, including an untreated
control each consisting of 2 replicates of 8 abalone. The treatment air exposure timeswere 1, 2, 3 and 4 h. Air temperature was 21 ± 0.5 °C and humidity was 60%. The
experiment was conducted in the laboratory using reverse cycle air conditioning. Theuntreated control group was maintained in the recirculating system at 15°C. In this
trial the recovery time was 3 rather than 7 d before processing.
Trial 5. Air drying of old, heavily, polychaete infested greenlip abalone
A group of 60- 85 mm greenlip abalone was obtained from Tasmanian Tiger
Abalone, Dunalley (section 4.2). The stock was 5-6 years old and had become spionid
infected at least 3 years previously. Previously the abalone were cultured in a sea
based grow out system but had since been transferred to a land based system with
filtered water. The animals were survivors of a group with mortality linked to mud
worm infestation commencing 3-4 years previously. The health of the stock was
considered poor with some of the animals apparently under weight for their shell
length. The shells were eroded on the dorsal surface and had blistering to 20-30% of
the ventral shell area.
From the pool of stock 20 B. knoxi positive abalone were chosen for the
experiment and tagged. There were two experimental groups: an untreated control and
a group treated by air exposure. Ten animals were assigned to each group with 2replicates of 5 per group. The treatment conditions were 4 h air exposure at 21 ± 0.5
°C. Treatment was performed in the laboratory using reverse cycle air conditioning
and humidity was 60 %. The untreated control group was maintained in the holdingsystem at 15 °C.
FRDC Final Report Page 25
Mudworm control in abalone
Trial 6. Air drying of recently infected stock under field conditions
This experiment was performed at Huon Aquaculture on December 9 1998.
Stock were blacklip abalone approximately 3 years old when placed on the farm inAugust 1998. Length was 46.5 mm ± 2.9 mm (mean ± SD, n=40). Settlement of
B. knoxi and P. hoplura spionids was known to have occurred in the interval between
placement and treatment. The exposure time was 3.5 h and ambient temperature andhumidity were 16-18°C and 49-62% respectively during the time period 12:30 h to
16:00 h. Abalone were removed from each of 2 replicate culture vessels and placed
on plastic sheet in direct but weak sun light for 5 min and then in shade for the
remaining time. Thirty abalone were assigned to control and treatment groups from
each replicate. Stock was not inspected to exclude not infected abalone. The control
group was returned to water as soon as possible after selection.
From the pool of stock, 20 treated and 20 control abalone (in 2 replicates of 10)
were taken to the laboratory for efficacy analysis. The remainder stayed at the site to
provide data on long-term treatment benefits (section 5.5).
Trial 7. Air drying of heavily fouled, P. hoplura infected stock under field conditions
This trial was performed at Aquatas on January 20 1999. Experimental animals
had been transferred to the farm in December 1997 at approximately 2 years of age.
Stock at treatment were 30-50 mm in length and had acquired various degrees of
fouling with Pomatoceros sp., Spirorbis sp. and Pacific oysters. Previous examination
of the stock indicated P. hoplura was the most common spionid present.
Exposure time was 4 h between 10:30 h and 14:30 h. Treatment group animals
were placed in direct sunlight for the first 10 min of air exposure and in shade onplastic sheet for the remaining time. Air temperature ranged between 17.5 and 22 °C
and air humidity between 43 and 65%. Twenty animals were assigned at random to
control and treatment groups without inspection to exclude any abalone not infected.
The control group was returned to water as soon as possible after selection.
Trial 8. Two and half hour drying of recently B. knoxi infected stock
Blacklip abalone, mean length 42.3 mm (SE =0.6 mm, n=80), were collected
from Huon Aquaculture and treated on site 12 November 1999. Stock had been on site
for 12-14 months but the infection rate from the preceding spring 1998 B. knoxi
settlement period was considered low. Stock was treated towards the end of the
presumptive 1999 B. knoxi settlement period. The maximum recent B. knoxi infection
period was approximately 3 months.
Forty animals were assigned at random to control and treatment groups with out
inspection to exclude not infected stock. The treatment group was placed on plastic
sheet outside in the shade. The day was sunny and air temperature ranged from 16 to17 °C during the 2.5 h exposure. Humidity was 50 to 54%.
Trial 9. Drying of 14 month mud worm infested blacklip abalone
Blacklip abalone, mean length 52.6 mm (SE = 1.0 mm, n=20), were collected
from Huon Aquaculture and treated outside at the laboratory 25 October 1999. Stock
was approximately 4 years old and had been on site for 14 months, acquiring shell
fouling in addition to mud worm infestation.
FRDC Final Report Page 26
Mudworm control in abalone
Ten abalone from each of 2 rearing vessels were assigned to a treatment or
control group giving 2 replicates of 5 animals per treatment. Control group animals
were tagged and returned to water as soon as possible. Treatment group abalone wereexposed to air for 3.5 h. Air temperature ranged from 16 to 20 °C (but was generallyin the range 16 – 17 °C), humidity ranged from 48 to 59%. Post treatment abalone
mortality data was not collected in this trial
Trial 10. Repeat drying of abalone previously treated one year earlier
Blacklip previously treated in December 1998 were re-treated December 13 1999.
Stock were 60.9 mm (SE = 0.8, n = 19) and drawn from the same population as in
Trial 6 above. Abalone were dried at Huon Aquaculture for 4 h. Temperature rangedfrom 15-18°C and humidity from 46 and 58% after an early reading of 80%.
4.13.3 Drying and mortality
In a preliminary drying mortality trial 140 blacklip abalone 15-20 mm were
obtained from farm 1 (section 4.2). There were 6 exposure times (1-6 h) and an
untreated control with 20 animals in each treatment. Abalone were dried using reversecycle air conditioning at 21°C ± 0.5°C. Post treatment mortality was recorded for 17 d
while animals were held in small plastic aquaria within the recirculating system
(section 4.3).
A second drying mortality experiment used 156 blacklip abalone (41.5 ± 4.1 mm,mean ± SD) obtained from Huon Aquaculture. Mud worm infection was minimal in
the group and the animals had been tagged previously. After 5 d to acclimatize,
without feeding, abalone were randomly assigned to 12 baskets with 13 abalone per
basket. Experimental treatment consisted of 3 exposure times: 5, 8 and 11 h and an
untreated control. There were three replicates for each treatment. Cages were 200 mm
by 200 mm and 250 mm high with 6 mm mesh bases and plastic sides. Cages were
semi-randomized in the recirculating holding tank in block design.
Abalone were dried in their cages outside on a cool sunny day April 5 2000.
The temperature varied between 15 and 20 °C and humidity between 40 and 73% (and
was less than 65% for all except the initial reading). Following air exposure abalone
in cages were returned to water and fed to slight excess for 54 days. Water
temperature was measure daily and ammonia and nitrite were measured 2-3 times per
week using appropriate aquarium test kits (Australian Pet Supplies, Smithfield NSW).
Growth comparisons between treatments were made by one way ANOVA on the
calculation of SGR length and weight (section 4.8) for individual animals. A further
treatment with an exposure time of 15 h was run concurrently but with an abbreviated
recovery period. Two replicates of 15 abalone were exposed over night using reversecycle air conditioning. Temperature range was 17-21 °C and humidity was 65%.
Abalone were then returned to water and housed as described above for 2 weeks.
4.13.4 Drying and long term growth
Two hundred blacklip abalone, mean length 39.3 mm (SD = 4.7 mm) and
weight 8.9 g (SD = 3.3 g) were obtained from an east coast land based farm (referred
to hence as farm 2). All the animals were tagged and 100 (in 2 replicates of 50)
assigned to each of an untreated control and drying treatment group. Air dried abalonewere placed in shade on a sunny day for 4 h; the temperature range was 16-20.5°C
FRDC Final Report Page 27
Mudworm control in abalone
and the humidity 57-65% except for a short interval at 69%. Replicates were dried on
separate sheets of plastic in the same general location and assigned to separate "hides"
in a commercial scale grow-out tank. Control animals were returned to water
immediately after tagging with each replicate placed in a separate hide. As many
tagged experimental abalone as possible were recaptured and re-measured 168 d
A similar experiment was conducted 6 October 1999 at Tasmanian TigerAbalone, Dunalley (section 4.2) using greenlip × blacklip hybrids. A total of 200
abalone, mean length 30.4 mm (SD = 5.6 mm) and mean weight 3.8 g (SD = 1.7 g),
were tagged for treatment with replication as above. Air exposure time was 3 h with atemperature range of 15-17°C and a humidity range of 60-84%. Abalone were placed
in direct sunlight for up to 10 min at a time to ensure thorough drying. Treatment and
control groups were returned to a commercial scale grow-out tank with 50 treated and
50 untreated animals assigned to each of 2 replicate hides. After a 214 d growth
period experimental abalone were recaptured and re-measured.
Experiment 3 commenced at Huon Aquaculture 15 February 2000. The abalone
used had been present on the farm for 6 months and were 2 years old. Mean lengthand weight (± SD, n=146) were 37.4 ± 4.1 mm and 7.3 ± 2.2 g respectively with
approximately equal numbers in a treated group and a control. Air exposure was 2.5 hwith a temperature and humidity range of 20-21 °C and 56-64% respectively. The ongrowing period was 210 d during which abalone were grown in Aquatech Trays
(section 4.3) with a similar number of treated and control animals in each of 2 trays.
The null hypothesis in long term growth trials was that there would be no difference
in growth between air dried and control stocks. Statistical analysis for the three
growth comparison trials consisted of Specific Growth Rate comparisons for weight
and length between treatments.
4.14 Epidemiology/Risk factor methods
4.14.1 Experimental animals and location
Abalone used in the experiments were H. rubra free of spionid infestation and
sourced from a land based farm outside the study area in southern Tasmania.
Experimental work was conducted at Huon Aquaculture and Aquatas in southern
Tasmania using the husbandry methods described previously (section 4.3).
4.14.2 Influence of size experiment
Three abalone size cohorts of approximately 6 months, 18 months and 3 years ofage were selected. Samples of stock were 15.0 ± 0.4 mm (n=23), 34.3 ± 0.5 mm(n=100) and 50.9 ± 0.7 mm (n=20), ( X ± SE). These size groups hence forth referred
to as "small", "medium" and ‘large" were considered to represent the extremes and
middle of the range at which sea based grow out farms are likely to purchase hatchery
Fifty stock of each size class was placed in 4 culture vessels. Two such cages
were hung within 2 m of each other at each of the two study sites. Experiments began
in the Austral winter and were concluded in the late summer exposing abalone to
presumptive spring/summer spionid settlement periods.
Mud worm infestation levels were quantified by exposure to vermifuge solution
(section 4.17.2). Preliminary investigation showed low rates of mud worm settlement
FRDC Final Report Page 28
Mudworm control in abalone
so abalone were shucked and shells exposed to vermifuge solution in replicates of 5.
Counts of characteristic B. knoxi chimneys, expelled B. knoxi worms and P. hoplura
worms were performed. Shell damage was assessed by the % blister and SSDR
methods as described above. Basket replicates for each study site were assessed for
each size class and measured variable by Mann-Whitney U Test. Where replicate
variables were not significantly different (P>0.05) they were combined for further
assessment. Size class data were compared by Kruskal-Wallis test for each of:
B. knoxi chimneys, B. knoxi worm counts, P. hoplura worm counts, SSDR, and %
blister coverage. Mean separations for significant Kruskal-Wallis tests were
performed by the method of Zar (1984).
4.14.3 Fouling organism survey method
A survey of B. knoxi chimney location was conducted on 80 abalone 20- 40 mm
in length and infested for less than 6 months. Shells were examined with low power
stereo microscopy. Preliminary examination suggested the use of common chimney
location categories. All chimneys were assigned to such a category, these being: on
spirobids, on other shell fouling organism, within the apical groove of the shell and
elsewhere on the shell.
Nine abalone fouled with the red bryozoan were collected during October 1998
from Huon Aquaculture. These animals were remnant stock from a cohort that had
suffered considerable mud worm mortality in 1996 and 1997. The abalone had a mean
length of 56 mm (SE=3 mm) and were at least 4 years old at the time of collection.
B. knoxi chimney counts were compared to those of 10 animals from the same intaketime and culture vessel with out the bryozoan (length 64 ± 2 mm, X ± SE). Chimney
counts of bryozoan and non-bryozoan fouled stock were compared using Mann-
Whitney U Test.
4.14.4 Method for assessment of spirorbid fouling effect
Four hundred abalone, a sample of which measured 34.3 ± 0.7 mm ( X ± SE,
n=200) were selected on the basis they had little obvious spirorbid fouling.
Subsequent microscopic examination showed mean spirorbid infestation per abalonein these "clean" stock was 7.8 ± 0.7, n=100). Relatively heavily spirorbid infestedstock from the same size, age and tank cohort had a mean count of 62.0 ± 4.0 (n=32).
One hundred "clean" stock were placed in each of 4 rearing vessels, two at each
study site. Additionally, 23 and 11 spirorbid infested stock were assigned to each
basket at Huon Aquaculture and Aquatas respectively. The exposure period was the
same as that for the size effect experiment described above. Some nominally clean
stock were removed before completion to provide data for other studies on timing of
mud worm settlement. Counts were performed as described above in replicates of 5
shells for expelled B. knoxi and P. hoplura worms and B. knoxi chimney counts were
performed on individual shells. Shells were assessed for mud worm shell damage by
Subjective Shell Damage Rating (SSDR) described above (section 4.9). Basket
replicates in the spirorbid fouling experiment were compared using the Kolmogorov-
Smirnov Two-sample test for data collected in replicates of 5. The Mann-Whitney U
Test was used for data collected from individual shells. Where replicates were not
significantly different (P>0.05) they were combined and further tests as appropriate
conducted between nominally clean and spirorbid fouled stock for each of: B. knoxi
chimneys counts, B. knoxi and P. hoplura worm counts and SSDR. Where basket
FRDC Final Report Page 29
Mudworm control in abalone
replicates could not be combined the 2 statistical tests described were performed
separately as appropriate between "clean" and fouled stock. Tables in the results
section indicate whether basket replicates have been combined or not.
4.14.5 Comparison with long term spionid affected stock
Further stocks transferred to Huon aquaculture in August and September 1998
and reared for in excess of 2 years were available for comparative purposes. Samples
of animals transferred in August had mean length 46.9 mm (SE=0.3, n=100) and in
September 18.8 mm (SE=0.1, n=150). The former stock had some spirobid fouling
(generally < 20 per abalone) whereas the latter animals were essentially spirorbid free.
4.14.6 Rearing vessel comparison
This comparison used similar abalone stocks being held in different rearing
vessels in the winter/spring of 1999 at the 2 study sites. Experimental animals had
been placed on the study sites August 1998, promptly becoming infected. By the
winter/spring of 1999 abalone were between 50 and 65 mm in length and
approximately 4 years old.
Abalone rearing vessels were of two designs. "Tube type" vessels were in
widespread usage when stock mortality was originally recorded in 1995-1996. These
were 1500 mm lengths of 250 mm diameter PVC pipe fitted with 6 mm mesh ends.
"Basket type" culture vessels were modified polyethylene laundry baskets fitted with
6 mm oyster mesh over existing mesh sides and plastic inserts to increase mesh free
substrate area. The "baskets" were approximately cylindrical, 800 mm high with a
diameter of 400 mm and had considerably more meshed area than "tubes".
Spionid counts were performed before and after the known settlement period
for the pest species B. knoxi. At Huon Aquaculture a pair of "basket" reared stock
were compared to a pair of "tube" reared stock. Additionally, another pair of basket
reared stock with known lesser levels of spionid infestation were available
At Aquatas one pair of "basket type" and a single "tube type" rearing vessel
stocked with similarly infested abalone were available for comparison purposes.
Polychaete counts before and after the B. knoxi settlement season and between rearing
container types were compared using the Mann-Whitney U Test.
4.14.7 Position in water column
One hundred abalone were placed in duplicate at each of 3, 6 and 9 m from the
surface in 10 m of water at Huon Aquaculture. The 40-45 mm animals were
transferred in August 2000 and exposed to spionid settlement until January 2001.
Following exposure to chemical vermifuges spionids and B. knoxi chimneys were
recorded for replicates of 10 abalone. A SSDR was assigned to each shell as an
indicator of spionid impact.
4.14.8 Species comparison
One hundred H. laevigata 26.7 ± 3.2 mm and 100 H. rubra 27.3 ± 2.8 mm( X ± SD, n=100) were placed in each of two "basket type" rearing vessels at Huon
Aquaculture. Stock were transferred in August 2000 and assessed January 2001,
allowing exposure to the presumptive 2000 B. knoxi settlement period. Abalone were
FRDC Final Report Page 30
Mudworm control in abalone
shucked and exposed to chemical vermifuge solutions in replicates of ten to expel
mud worms. Spionid counts between abalone species were compared by Mann-
Whitney U-test.
4.15 Abalone health I: mortality, growth and condition
4.15.1 Blister morphology & location
The size and severity of mud worm blisters was assessed by the blister tracing
and subjective shell damage rating methods as described previously. The location of
blisters was quantified by assigning blisters to a type locality map as depicted in
Figure 4.7. Six hundred blistered shells were assessed and separate location records
kept for those shells infected by a single species of spionid.
The volumes of a sample of the largest mud worm blisters encountered were
measured by allowing shells to dry, puncturing the blister and then submerging shells
in water. The water was then removed from the blister with a 21 gauge needle and 1-5
ml syringe as appropriate and the volume recorded. For comparison purposes a
sample of the largest blisters formed in Pacific oysters by B. knoxi at Huon
Aquaculture were assessed. Histological examination of blisters was performed on
seawater formalin fixed, decalcified shells.
FRDC Final Report Page 31
Mudworm control in abalone
Figure 4.7 Common mud worm blister locations in abalone
FRDC Final Report Page 32
Mudworm control in abalone
4.15.2 Measures of abalone condition
The condition, or relative "fleshiness" of abalone was assessed by calculating
the percentage flesh weight. This is simply the shucked flesh weight divided by the
whole live weight and multiplied by 100. An advantageous of this method was that
after measurement the flesh could be fixed in formalin for histology or frozen for later
chemical testing. Dry weight indices were calculated by separating meat and shellsand drying in an incubator at 70° C for 48 h until constant weight was achieved.
The dry weight index CILENGTH was calculated as:
CILENGTH = Dry flesh weight (g) × 100
Shell length (mm)
The dry weight index CIWEIGHT as recommended by Lucas and Beninger (1985) was
calculated as: CIWEIGHT = Dry flesh weight (g) × 10
Dry shell weight (g)
The dry to wet flesh weight ratio as also recommended by Lucas and Beninger (1985)
for use in aquaculture studies was also calculated. The various indices were compared
using non infested abalone to find the most suitable measure for long-term health
4.15.3 Experimental design: growth, mortality, long term treatment efficacy
Placement of non mud worm infected stock at two sea lease sites with a history
of severe mud worm infestation was intended to recreate the conditions which led to
stock mortality in the mid to late 1990's. To this end the same hatchery and feed
sources were used as were the initial containment vessels. Regular sampling to
determine spionid infestation levels was performed and the consequences of this in
terms of stock mortality and health indicators such as growth, condition and shell
blistering was quantified.
By allowing a stock cohort to become mud worm infested and then treating a
portion of that stock to eliminate or reduce spionid infestation, the affects of such
infestation could be compared for otherwise matched groups. The null hypothesis was
that differential spionid infestation levels would have no affect on abalone health
measures including mortality rate, growth, shell blistering and condition indices. This
experiment was conducted using August 1998 intake abalone at Huon Aquaculture
and stock assigned to this experiment are referred to as "cohort 1" in the results and
To control mud worm infestation levels in one group of stock relative to the
other air drying of half the animals was conducted in December 1998 and 1999.
Details of the drying conditions and efficacy are given in section 4.13.2 (Trials 6 and
10). December was chosen as the drying period as initial data suggested that larval
settlement of B. knoxi, the major focus of the research, had concluded by then (section
5.1). For the experiment comparing health of matched infested and non-infested stock
to succeed, long-term suppression of spionid infestation in one of the groups would be
necessary. This was an experiment in itself, with the null hypothesis that annual post
B. knoxi settlement season air drying treatment would severely reduce spionid
infestation by this species.
FRDC Final Report Page 33
Mudworm control in abalone
The recommendation from section 5.1 that B. knoxi infestation could be avoided
by placement of stock post November was tested by comparison of stocks placed in
September 1999 and December 1999 at both southern study sites. The null hypothesis
was that there would be no difference in B. knoxi counts and shell blistering between
the two intake time cohorts.
Long-term treatment trial data (Huon Aquaculture August 1998 cohort 1) were
analysed using ANOVA or REML in situations where sample sizes varied giving an
unbalanced non-orthogonal design. Thus spionid count data was analysed using two-
way ANOVA, after log transformation of data and blister coverage data was analysed
using REML. Percentage flesh weight data was arcsine transformed using then
analysed using 2 way ANOVA. The non-parametric Mann-Whitney U Test was used
to analyse SSDR data. Linear Regression data were analysed using the simple linear
regression option in Genstat. Residual plots were examined to check for major
departures from the assumptions of normal distribution and uniform variance.
4.16 Abalone health II: physiology and histology
4.16.1 Clinical Pathology
Haemolymph samples were drawn from a foot incision as described for
haemocyte counts or from the cephalic sinus (Jorgensen et al. 1984). This site is
located 2-3 mm deep on the midline between the mouth and the anterior portion of the
foot. Samples were taken by use of a 1 ml syringe and 27 gauge needle. At least 0.3
ml was drawn from the appropriate sample site and centrifuged at 3000 rpm for 3
minutes to remove haemocytes. Samples not analysed immediately were frozen at -20°C for subsequent analysis.
Measurement of protein, glucose and all ions except copper was performed on a
Roche Cobas-MIRA automatic analyser. Samples were diluted 1:4 for sodium,
potassium, chloride and calcium and 1:40 for magnesium. Sodium and potassium
were measured using ion-selective electrodes. Chloride was assayed
spectrophotometrically using the thiocyanate method (Cobas-MIRA, 1987). Calcium
and magnesium ion concentration was determined spectrophotometrically using the
arsenazo method (Cobas-MIRA, 1987). Glucose and protein were undiluted and
determined spectrophotometrically using the hexokinase and biuret methods
respectively (Cobas-MIRA, 1987). Copper concentration was determined by adding
0.1 ml sample to 1 ml of 20% trichloroacetic acid and reading at 324.8 nm on a
Background data on normal levels for non-spionid infested abalone was
established by sampling groups of 5-10 presumptive healthy animals acquired in the
period 1998-2000. Such animals were growing well at the time and had no obvious
health problems. The abalone were obtained from farm 1 or Site 3 on the east coast.
These were compared to mud worm infected stocks from Huon Aquaculture, Aquatas
and Tasmanian Tiger Abalone. Samples assigned to the mud worm affected group
were collected 1998-2000 and comprised moderately to severely infested animals.
The August 1998 intakes of abalone to Huon Aquaculture and Aquatas were
sampled at regular intervals until mid- late 2000. These groups were subsequently
shown to be the most severely mud worm infested (section 5.5) and thus of most
interest for clinical pathology investigations.
Statistical methods for comparison of data varied with the size and nature of data
sets. The non-parametric Mann-Whitney U Test was used to compare haemolymph
ions, copper, protein and glucose drawn from cephalic sinus haemolymph between
FRDC Final Report Page 34
Mudworm control in abalone
presumptive normal and mud worm infested stock. As a larger data set was available
for haemolymph drawn from the foot, distributions approached the normal
distribution and the more powerful t-test was used. Similarly, the distribution free
Kruskal-Wallis test was used to assess Na+/K+ ratio data from the relatively small data
set of starved animals (section 5.6.1) whereas one way ANOVA was used to assess
the larger temporal variation data sets from the two study sites.
4.16.2 Haemocyte counts
Haemocyte counts were carried out to help define the physiology with regard
to stock stunting. In order to interpret these findings, additional tests on the effect of
temperature and copper exposure on haemocyte counts were carried out as an
indication of haemocyte sensitivity and the practicality of haemocyte counts as a
general health indicator in abalone.
Total haemocyte counts were performed with a haemocytometer (Assistent,
Germany) on samples taken from an incision in the foot, as many animals were too
small for reliable collection from haemolymph sinuses. A small cube of flesh was
removed with a scalpel blade, severing haemocyte channels, and approximately 0.2
ml of haemolymph/tissue fluid transferred from the wound to the haemocytometerwith a syringe. Counts were performed immediately at ×200 magnification by light
microscopy to avoid aggregation of haemocytes. Five of 25 small squares were
counted on each side of the haemocytometer (total volume 0.2 mm2 area x 0.1 mm
depth) and a mean calculated for each sample (Count data were converted to cells.ml-1
by multiplying by 50 000).
A normal range was established by collecting data from presumptive healthy,
mud worm free abalone in the range 30-65 mm. Samples generally consisted of at
least 3 abalone acquired from land-based farm 1, sea-based Site 3 (East Coast) or
from Huon Aquaculture within 3 months of transfer and in the absence of shell
blistering. Simple linear regression analysis of size (length) versus count data was
performed using Genstat.
From 1999 regular samples were taken from the August 1998 intakes at Huon
Aquaculture and Aquatas as these were the most heavily mud worm infested groups.
Overall mean counts from the 2 sites were compared using Mann-Whitney U-test.
Abalone from 3 age classes were collected from farm 1, September 9 2000 to
examine variations in haemocyte counts between stunted and fast growing stock of
the same age and from the same tank. Three year old animals were assigned to 1 of 2
size classes. These being "runts" (mean shell length 36.9 mm, SD=2.5, n=10) and
larger animals (mean shell length 53.3 mm, SD=1.9, n=10). Ten, 2 year old animals
were assigned to each of three relative size classes these being "runts", medium and
large stock. Mean length of 2 year old abalone size classes were 27.4 mm (SD=2.6),
44.0 mm (SD=2.7) and 54.6 mm (SD=2.9). A sample of larger abalone only, less than
6 months old, were also collected: mean length (26.1 mm, SD=1.7, n=10).
Further data from one and two year old stock was collected from farm 1 February
9 2001. Stocks were selected to fit 1of 3 relative size classes these being "runts",
medium and large stock. Ten animals were bled for each age and size class. Mean
length of 2 year old abalone size classes were 34.9 mm (SD=4.0), 45.1 mm (SD=2.9)
and 58.2 mm (SD=3.9) and of one year abalone: 15.5 mm (SD=1.6), 24.6 mm
(SD=1.4) and 30.0 mm (SD=1.6). Data were tested using ANOVA for differences due
to size and age class.
For experiments using temperature stress to assess the suitability of haemocyte
counts for evaluation of short term environmental stress, thirty abalone of mean length
FRDC Final Report Page 35
Mudworm control in abalone
60.4 mm (SD=4.0) were obtained from farm 1. These animals had been exposed, onsite, to relatively warm water (22-23 °C) for 4-8 weeks and were removed to water at16 ± 0.5°C on February 9 2001 (recirculation system- Fish Health Laboratory).
Haemocyte counts were made 4, 7 and 14 days later and were compared using
A further investigation commenced March 21 2001 where abalone wereremoved from tanks at 14-15°C to tanks at either 16.5 ± 0.5°C or 21 ± 0.5°C for a
period of 6 d. This experiment was conducted in 40 l aquaria with complete water
changes daily. Mean length of abalone was 53.9 mm (SD=4.4, n=50) and the animals
were not fed during the trial. Twenty abalone were assigned to each temperature
treatment in 2 replicates of 10 animals. Haemocyte counts at higher and lower
temperature were compared using Mann-Whitney U Test.
For examination of the short-term effects of the known toxicant copper
(Mance 1987) on abalone haemocyte counts, abalone, mean length 51.3 mm (SD =
4.1, n=18) were assigned to either an untreated control group or 0.2 mg.l-1 coppersulphate exposure for 9 d at 15.5 ± 0.5 °C. Animals were housed in 40 l aquaria
without feeding. Haemocyte counts of copper exposed and control groups were
compared using Mann-Whitney U test.
Abalone tissues were fixed in 10% seawater formalin. Routine processing was
performed using a Shandon Hypercenter XP for dehydration through ethanol to
xylene then paraffin embedding on a Tissue tek work centre and sectioning on aMicrom HM 340 microtone at 4 µm. Routine Harris's Haemotoxylin and Eosin (H &
E) staining was performed using a Leica Jung Autostainer XL for automatic staining.
Sections were mounted in DPX and examined and photographed through a light
Tissues routinely examined included right kidney, digestive gland, intestine, gills
and foot. Some comparisons were made with Martius Scarlet Blue (MSB) (Ellis,
1992) stained sections to examine foot muscle depletion in spionid infested abalone.
Sections of severely mud worm infested abalone were stained using the Periodic Acid
Schiff reaction (PAS) (Ellis, 1992) to check for fungi and tissue glycogen levels.
Unaffected control animal material was also PAS stained for comparison.
Remnant severely mud worm infested abalone stocked at southern sea farms in
the mid 1990's were sampled for histological examination in the period 1998-99.
These changes were compared to changes that occurred when mud worm free stock
cohorts were transferred to the same areas in August 1998 and September-November
1998. A subjective score was developed to assess the extent of brown pigment
granules some times present in the right kidney and digestive tubules of abalone
sampled. A zero score indicated no pigment and a score of 3 indicated maximum
pigment levels seen in worst affected animals.
4.16.4 Blister environment and microflora
The pH of a sample of 10 large blisters was measured by drawing at least 0.2 ml
of blister fluid with a syringe and needle and wetting the tip of pH test strip (range 4.5
-10.0, Sigma). Blisters tested came from remnant stock surviving the initial reported
outbreaks in the mid 1990's until sampling in 1998-99.
FRDC Final Report Page 36
Mudworm control in abalone
Similarly, samples of blister fluid from some of the most mud worm damaged
shells available were cultured for bacteria on TCBS, Johnson's Marine Agar andBlood Agar plates and incubated at 20 °C (AHL Fish Health Methods Manual, 2001).
Abalone shells and adjacent tissue were processed for histology and stained using
Periodic Acid Schiff reaction (PAS) (Ellis, 1992) to test for the presence of fungi.
Shells were decalcified using RDO rapid bone decalcifier (Phoenix Scientific, Vic.,
4.16.5 Tissue chemistry
Foot tissue was frozen and stored at –20°C and soluble protein content
measured using a Sigma Diagnostics protein assay kit (Procedure No. P5656) based
on the Lowry method. Sample preparation involved placement of 0.05-0.15 g frozen
tissue in cold SEI buffer (250 mM sucrose, 5 mM EDTA in 0.1 M imidazole buffer).
Tissue was then homogenized with a teflon rod while kept on ice. Homogenate was
centrifuged for 7 min at 3500 rpm and the supernatant discarded. The remaining
pellets were resuspended in 1 ml SEID solution (consisting of SEI plus 0.1 g sodium
deoxycholate per 100 ml SEI) re-homogenized and left on ice for 15 min. The
homogenate was then centrifuged for 6 minutes at 5000 rpm and a 0.2 ml sample of
supernatant diluted to 1 ml as per protein test kit method. Absorbency was measured
at 700 nm with a light spectrophotometer and the protein concentration determined
from a standard curve. Data were calculated as mg protein per gram tissue as
expressed at % protein wet weight foot tissue.
Statistical analysis of tissue protein data were performed using the
nonparametric Kruskal-Wallis test in conjunction with non-parametric Tukey-type
multiple comparisons to separate means (Zar, 1984).
Respirometry trials to assess the effect of mud worm infestation on metabolic
rate, were performed at University of Tasmania, Launceston in March 1999 and May
2000. In the first trial chronically mud worm infested stock (SSDR 1-3) were sourced
from Huon Aquaculture and compared to mud worm free stock of similar age from
farm 1. Mean shell lengths were 57.8 mm (SD = 2.7, n=8) and 58.1 mm (SD=7.4,
n=4) respectively. Abalone selected for the second experiment were part of the long
term treatment trial at Huon Aquaculture. Five mud worm infested animals withspionid counts of 62.8 ± 24.4 and blister coverage of 18.2% ± 9.8% ( X ± SD) were
compared to 5 lesser infested stock (previously air dried December 1998 and 1999)with spionid counts of 18.4 ± 5.8 and blister coverage 12.9% ± 9.0 ( X ± SD). Meanlengths of the untreated and less infested groups were 61.9 ± 3.6 mm and 64.9 ± 5.1
mm respectively.
Abalone were held for 1-2 weeks without food, after selection and transport and
were transferred to respirometry chambers attached to petri dish substrates to
minimize handling. One experimental animal was assigned to each plastic 1.5 l
respirometry chamber for data runs consisted of 5 abalone plus 1 blank. One ml water
samples were taken at regular intervals over a period of 2-4 h or until the water
oxygen tension dropped to approximately 100 mmHg partial pressure of oxygen.
Oxygen consumption was measured using an E101 oxygen electrode in conjunctionwith a Radiometer BMS Mk 2 blood gas analyzer maintained within 0.5 °C of
ambient respirometry chamber water, and connected to a PHM 71 Acid-Base
FRDC Final Report Page 37
Mudworm control in abalone
Analyzer (Radiometer, Copenhagen). The oxygen meter was calibrated using 2%
sodium sulphite (zero PO2) and air saturated seawater set to a PO2 of 159 mmHg.
Sample readings were taken after 3 min equilibration.
Trial 1 was conducted at 20 °C and trial 2 at 16 °C. Oxygen consumption was
measured as reduction in mmHg PO2, converted to µmol O2 (Cameron 1986) and
expressed as µmol O2.g-1soft body weight.h-1. Statistical comparison was made using
Mann-Whitney U Test as data sets were small and underlying distributions unknown.
4.16.7 Ammonia excretion
Ammonia excretion rates were compared using the long term spionid treatment
cohort (August 1998 intake 1- section 4.15.3) at Huon Aquaculture, sampled in
October 2000. Ten heavily mud worm infested animals were compared to 10 less
infested animals air dried 10 months previously.
Mean length and weight of abalone were 63.6 ± 4.4 mm and 38.3 ± 7.1 g
respectively. Mean blister coverage was 28.6% (SD= 8.8) for highly infested stock
compared to 10.9% (SD=7.9) for the less infested group. These differences, which
could not be assessed until the completion of ammonia excretion measurements were
significant by Mann-Whitney U Test (U=9.0, P<0.01). Abalone were placed in 2 l
aquaria, with aeration at 13 °C for 6 h and 50 ml samples taken and frozen at –20 °C.
Ammonia was measured using the salicylate-hypochlorite assay (Verdouw et al.,
1978) against a range of standards read at 650 nm on a light spectrophotometer.
Ammonia excretion was expressed as µmole NH4-N.g-1.h-1 and statistical comparison
made by Mann-Whitney U Test.
4.16.8 Starvation comparison
One cohort of abalone with severe mud worm infestation began to die after
approximately 18 months exposure (section 5.5.1). As underfeeding was also
considered a factor in this outcome a 3 month starvation trial was conducted in an
attempt to differentiate between the effects of mud worm infestation and starvation.
A pool of 40 abalone, mean length 44.6 ± 4.2 mm, mean weight 12.7 ± 4.2 g was
obtained from farm 1 in early February 2001. Abalone were tagged, their length and
weight recorded and housed in plastic aquaria within the Fish Health Unit
recirculating system. At the beginning of the time sequence and at 4 week intervals
thereafter for 12 weeks 5 animals were sampled and haemocyte counts and Na+/K+
ratio determined. Material was also fixed for histology.
FRDC Final Report Page 38
Mudworm control in abalone
5. RESULTS & DISCUSSION
5.1 Reproductive biology and settlement of spionids
Five species of spionids were found in experimentally exposed abalone, these
being B. knoxi, B. chilensis, B. proboscidea, Polydora hoplura and P. armata. Of
these P. hoplura and B. knoxi were overwhelmingly the most common and were
therefore described in detail.
Most mature specimens of B. knoxi were 10-20 mm in length with the
occasional specimen up to 35 mm recorded. The mean of a sample of fixed specimens
was 16.3 mm (SD=16.1 mm, n=15) for a mean setiger count of 98.8 (SD=95.5, n=13).
Specimens of P. hoplura were generally 20-30 mm in length with larger specimens of
50 mm (>200 setigers) recorded. Specimens confirmed to line drawings and technical
descriptions of these species by Blake and Kudenov (1978), Rainer (1973) and Read
(1975).B. knoxi egg strings had a mean of 21.8 capsules per string (SD=8.6, n=9) and
capsules contained a mean of 32.8 larvae (SD=8.7,n=46) for a mean brood yield of
715 larvae. Egg strings often exhibited a greenish tinge and all eggs developed into
larvae, there was no evidence of nurse eggs. Asetigerous embryos were approximately240 µm long, growing to approximately 350 µm at 5 setigers when they were capable
of surviving outside the capsule. Larvae of this size were strongly phototaxic and their
translucent bodies had a green tinge. Larvae released from egg capsules at a mean sizeof 369 µm and cultured reached 670 µm 5 d later and 900-1200 µm for 16-17 setigers
about 2 weeks after release. By this time larger larvae were commencing
metamorphosis with the beginning of crawling behaviour, and showed the presence of
fifth setiger spines and palps. A small number of larvae were maintained for a further
week after this but failed to grow any larger.
Histology of adult B. knoxi showed that parapodial cavities were densely packedwith oocytes of 90-110 µm diameter in May. Sections of worms sampled June to
August showed essentially empty body cavities – corresponding with extrusion of
eggs from the body to the shell burrow. Immature oocytes could be seen developing in
the ovary by November/December and by January to March had been released once
more into the parapodial cavities to mature.
Egg strings of P. hoplura tended to be deep yellow in colour and were often 20-
30 mm in length for > 30 capsules. Nurse eggs were always observed and capsulesgenerally contained 40-50 such eggs and 4-6 larvae of about 500 µm early in thedevelopment sequence. Larvae as large as 1900 µm were observed within capsulesnear the exhaustion of nurse egg supplies. Small worms 2000-2500 µm were
sometimes observed excavating burrows adjoining the maternal burrow having never
apparently left the host shell. No direct evidence of planktotrophic larval development
of P. hoplura was observed in infested abalone.
B. proboscidea was seen sufficiently frequently to allow some assessment of its
reproductive cycle. Egg strings, with distinctive oval capsules characteristic of the
species (Woodwick 1977, Blake and Kudenov 1981) were seen once in each of
December 1998 and September 1999. Lecithotrophic larvae 1.4 mm long were
observed within capsules October 1999. Adults with well developed eggs within their
bodies were seen between September 1998 and January 1999.
FRDC Final Report Page 39
Mudworm control in abalone
Timing of settlement
Settlement of B. knoxi larvae was confined to the months of the Austral spring
in the period 1998-2000 at both study sites (Tables 5.1 and 5.2). Mature body eggs
were observed between April and August and extruded eggs in June and September.
Viable unhatched larvae were present in most August samples at both study sites with
the earliest observation June 1999 and the latest January 2001 (Tables 5.1 and 5.2).
Post-larvae of P. hoplura and adults with mature body eggs were observed in
every calendar month at one time or another during the study period (Tables 5.1 and
5.2). The majority of post larval worms were considered to be the lecithotrophic
offspring of worms already present in blisters. At times post larval P. hoplura were
found inhabiting otherwise spionid free shells and it was considered possible such
specimens had undergone planktonic development. Most of such specimens were
found in the spring and summer (Tables 5.1 and 5.2).
Settlement intensity was low compared to data for infestations during 1995-
1997. Abalone transferred to study site 1 in September 1998 had acquired a mean
spionid infestation of 1.2 (SE=0.2, n=64) worms per shell by January 2001.
The findings above relate to the specific project objective No. 2 on
investigation of spionid reproductive biology. Field studies between 1998 and early
2001 and the laboratory rearing trial ensured this objective was achieved. Knowledge
of spionid settlement patterns contributed to mud worm control strategies as given in
objectives 1 and 6 (page 9) and discussed below.
Of the 5 mud worm species found infesting stock, only B. knoxi and P. hoplura
were present in sufficient numbers to be considered pest species. Of the 3 minor
species P. armata has been previously recorded in H. rubra from Victoria and in
H. roei from South Australia (Blake and Kudenov 1978). B. chilensis is quite
common in Tasmanian oysters, but neither B. proboscidea nor P. armata were
previously known from this source (Wilson et al. 1993). P. hoplura had previously
been seen in Tasmanian abalone and oysters (Wilson et al. 1993) and the species has
often been associated with damage to commercially important bivalve species world-
wide (Blake and Evans, 1972).
The B. knoxi species appears to be somewhat larger in Tasmanian abalone than
described in New Zealand (Rainer 1973, Read 1975). There was no evidence of
brooded B. knoxi production as reported by Read (1975). Rather, the lack of nurse
eggs, release of larvae from capsules at the 4-5 setiger stage and relatively large clutch
size indicate planktonic larval production as described by Handley (1997, 2000). The
estimated clutch size of > 700 larvae for Tasmanian B. knoxi is similar to that of other
spionids with planktotrophic development including P. websteri (500-550, Blake1969), B. chilensis (460±160, Skeel 1979), P. ciliata (225-400, Wilson 1928; 200-
1100 Dorsett 1961) and P. haswelli (1200-2400, Skeel 1979).
By contrast, P. hoplura, although physically larger than B. knoxi, was less fecund
consistent with the observed lecithotrophic development. Maximum fecundity would
be less than 150-180 larvae, consistent with estimates for the species by Skeel (1979)
Radashevsky (1994), and Gromadzki (1994). There was no direct evidence of
planktonic larval production within P. hoplura blisters in cultured abalone. However,
the presence of P. hoplura larvae in otherwise spionid free stock at some distance
FRDC Final Report Page 40
Mudworm control in abalone
from the nearest known source of infection may suggest a swimming ability not
normally associated with large lecithotrophic larvae.
It would not be without precedent if P. hoplura were capable of producing two
types of larvae to maximize its reproductive potential. Wilson (1928) noted that if
larval P. hoplura within capsules containing brood eggs were removed and fed on
micro-algae they developed normally and were indistinguishable from larvae allowed
to complete lecithotrophic development. As noted previously B. knoxi apparently
produces both brooded and planktonic larvae in New Zealand.
The ability of P. hoplura to produce lecithotrophic larvae at most times of the
year once established in the abalone may render control difficult. Fortunately the
presence of P. hoplura post larvae on clean stock was mainly confined to spring and
summer (Tables 5.1 and 5.2). Likewise, B. knoxi settlement data, and other
observations of the reproductive cycle (including histology) strongly suggest that if
stock are placed post November they will remain substantially spionid free until the
following spring. Current sea based abalone grow-out operations generally place seed
stock at 20-30 mm and on grow to at least 60 mm. Placement of seed stock in
susceptible areas December to February should allow approximately a years growth
before the impact of any spionid settlement is felt. This may be sufficient to allow
grow out to marketable size. Where on growing to larger sizes is required treatment
options (sections 5.2 and 5.3) might be required during the second year. Infestation
rates for B. knoxi were low compared to available previous data suggesting
recruitment rates may vary considerably year to year.
FRDC Final Report Page 41
Mudworm control in abalone
Table 5.1.Presence-Absence of B. knoxi and P. hoplura life history stages at
Extruded egg strings
Settled post-larvae
+ = B. knoxi; • = P. hoplura; •# = suspected planktonic P. hoplura post larvae
Extruded egg strings
Settled post-larvae
Extruded egg strings
Settled post-larvae
Table 5.2 Presence-Absence of B. knoxi and P. hoplura life history stages at Huon
Extruded egg strings
Settled post-larvae
+ = B. knoxi; • = P. hoplura; •# = suspected planktonic P. hoplura post larvae
Extruded egg strings
Settled post-larvae
Extruded egg strings
Settled post-larvae
+** = record consists of 2 worms
FRDC Final Report Page 42
Mudworm control in abalone
5.2 Chemical treatments
5.2.1 Screening trials
Major findings for the considerable body of screening trial data are described
below, more detail is presented in Appendix 3, section 5, as indicated. Fresh water
immersion of spionids in vitro proved 100% fatal after 10 min exposure. Exposure of
spionids in situ proved ineffective for 1 h immersion, rising to 40% GMC (Group
Mean Comparison) kill at 2 h, at the cost of 80% abalone mortality (Appendix 3,5A).
Potassium permanganate exposure appeared to have some potential against
spionids in vitro at 15-20 mg.l-1, and 100% mortality was seen 7 d post exposure at 50
mg.l-1. Potential for abalone mortality was seen at 20 mg.l-1 and above with 80%
mortality seen 16 d post treatment at 20 mg.l-1, 4 h immersion. Three-hour treatment
of spionids in situ at 25 mg.l-1 KMnO4 resulted in 60% abalone mortality and 11%
GMC spionid kill (Appendix 3, 5B).
Gentian violet exposure was found to be 100% lethal to mud worms in vitro at
10 mg.l-1 4 d post treatment. Some abalone mortality was seen at 10 and 20 mg.l-1 but
not at 5 mg.l-1, 17 d post treatment. Four hour exposure of mudworms in situ to 7.5
mg.l-1 gentian violet resulted in 23% GMC kill (Appendix 3, 5C).
Spionid mortality in vitro approached 100% 9-10 d post treatment with
mebendazole and fenbendazole in the range 50-500 mg.l-1. No abalone mortality was
seen following 50 mg.l-1 exposure to mebendazole for 9 h and 200 mg.l-1 exposure for
3 h. Lethality to spionids in situ was estimated at 53% by GMC kill. Similarly, no
fenbendazole toxicity to abalone was seen at 250 mg.l-1, and GMC kill was 41% for
spionids tested in situ empty shells (refer to Appendices 5D and 5E)
Levamisole immersion of spionids in situ resulted in GMC kills of 42 and 66%
respectively at 64 and 640 mg.l-1, resulting in abalone mortality of 50 and 100%.
Follow up abalone toxicity trials using healthy 20 mm stock showed no mortality 8 d
post exposure to 64 mg.l-1 levamisole, rising to 33% at 320 mg.l-1 and 100% mortality
at 500 mg.l-1. Larger healthy stock (40-50 mm) showed no mortality at 320 mg.l-1 18
d post exposure (Appendix 3, 5F).
Malachite green exposure to mud worms in vitro was 100% lethal at 5 and 10
mg.l-1 but ineffective at 1 mg.l-1. Concurrent exposure to spionids in situ (empty
shells) gave GMC kill data of 38 and 60% at 5 and 10 mg.l-1 respectively. Toxicity
trials on healthy abalone (20 mm) showed 10% mortality at 5 mg.l-1 and approached
100% at 10 mg.l-1 (Appendix 3, 5G).
Spionids in vitro showed limited mortality 8 d post exposure to trichlorofon at
500 and 1000 mg.l-1. Healthy 40-50 mm abalone were not killed by exposures of up to
500 mg.l-1 11 d post treatment (Appendix 3, 5H). Similarly, praziquantel at up to 100
mg.l-1 produced limited mortality to spionids in vitro 20 d post treatment (Appendix 3,
5I). Hydrogen peroxide in the range 200-1000 PPM was lethal to 60% of spionids in
vitro. No abalone mortality was seen at in healthy stock at 200 PPM (Appendix 3, 5J).
Formalin produced 30% mortality at 100 PPM for spionids in vitro, dropping to
22% (GMC kill) for spionids in situ empty shells. At 200 PPM in vitro spionid
mortality exceeded 80%, dropping to 49% for concurrent estimate in situ. Follow up
trials on healthy abalone showed no mortality a week post treatment at 100 PPM but
90% mortality at 200 PPM (Appendix 3, 5K).
Mud worm toxicity in situ was zero at 0.004 and 0.04 mg.l-1 ivermectin rising to
57% (GMC kill) at 0.4 mg.l-1 with mortality of 85% for infested abalone at the same
concentration. Follow up work in the range 0.1-0.3 mg.l-1 showed ivermectin was
FRDC Final Report Page 43
Mudworm control in abalone
ineffective against spionids in vitro and 100% lethal to healthy 20 mm abalone above
0.2 mg.l-1 (Appendix 3, 5L).
A commercial product, Exelpet , "All-Wormer for Dogs" containing febantel,
pyrantel embonate and praziquantel (at 125, 72 and 25 mg.l-1 respectively in solution)
was ineffective against mud worm in vitro and non lethal to healthy abalone at twice
the concentration (Appendix 3, 5M).
Metronidazole at 200 mg.l-1 was non lethal to spionids in vitro and similarly
dimetronidazole produced limited mortality at 500 mg.l-1(Appendix 3, 5N).
Methylene blue was found non toxic to spionids in situ empty shells and healthy
abalone at up to 10 mg.l-1. Further trials on spionids in vitro showed no mortality at up
to 200 mg.l-1 (Appendix 3, 5O).
5.2.2 Follow up chemical treatment trial on lightly spionid infested abalone
Gentian violet at 5 mg.l-1, mebendazole at 200 mg.l-1 and potassium
permanganate at 15 mg.l-1 were chosen on the basis of screening trial data for follow
up bath trials on spionids in situ lightly infested abalone. Spionid killing efficacy data
were of marginal statistical significance (P=0.057, Pearson Chi-square value 7.5, 3
df). Mebendazole was the most effective of the bath treatments with a mean B. knoxi
estimated individual percentage kill of 32.5% (Table 5.3). Gentian violet treatment
resulted in the death of 1 in 20 experimental animals.
Table 5.3 Treatment of lightly infected abalone by one of three chemical baths
(means ± SD).
mebendazole KMnO4
Mean B. knoxi chimneys 1.5 ± 0.9
Mean surviving B. knoxi 1.4 ± 0.7
Mean EI% B. knoxi Kill
Abalone Mortality (%)
n=20 each treatment
The testing of chemical treatments was commenced as part of the preceding CRC
funded project and continued as part of objectives 3 and 4 in this FRDC project. As
indicated by the data above (and Appendix 3, section 5), short term chemical
treatments were not highly promising and once this was apparent greater research
effort was directed towards an alternative treatment (section 5.3).
Sixteen potential chemotherapeutic agents and fresh water immersion were
tested. Available literature on effective concentrations for bath treatment of fish and
shellfish parasites was used as a starting point. It was concluded that trichlorofon,
praziquantel, hydrogen peroxide, febantel, pyrantel embonate, metronidazole,
dimetronidazole and methylene blue were not effective against spionid polychaetes at
doses which were high relative to available literature.
Immersion in freshwater, potassium permanganate, gentian violet, levamisole,
malachite green, formalin and ivermectin was highly effective against mudworms in
vitro. However, the protection conferred by the burrows of spionids in situ rendered
effective concentrations unsafe for abalone. The benzimidazoles (mebendazole and
FRDC Final Report Page 44
Mudworm control in abalone
fenbendazole) showed the largest differential between the level toxic to mud worms in
vitro (100% mortality at 50 mg.l-1) and level toxic to stock (0% mortality at 200 mg.l-1
or greater). However, as for other chemotherapeutic agents, the protection conferred
by spionid burrows rendered the drugs largely ineffective for bath treatment. This may
be compounded by their poor solubility in water.
The benzimidazole class of drugs may have some potential for
microencapsulation as treatment for various polychaetes, (including families other
than the Spionidae such as Serpulids and Sabellids), with infest abalone. This
approach has been explored previously by Shields et al. (1997) using copper sulphate
as the effective agent to treat a sabellid polychaete. More recently Overweter (2000)
used microencapsulated albendazole with success against the calcareous tube building
polychaete Galeolaria sp. Further work in this area was not considered a high priority
because the alternative approach of air drying set out in the next section, appeared
more promising.
5.3 Air drying treatment
5.3.1 Treatment efficacy of air drying.
Trial 1. Treatment of remnant severely infested abalone by shell drying.
Results for both efficacy and safely in severely affected abalone are presented
in Table 5.4. ANOVA (arcsine transformed data) of estimated % kill data was highly
significant (F=19.2, df 3,34 P<0.001) with a mean 90% kill rate seen for the 8 h
exposure. Abalone mortality occurred at 5 and 8 h exposure times.
Table 5.4 Treatment of severe B. knoxi infestation by drying: 3-8 h, 24 °C
Air Exposure Time (Hours)
B. knoxi chimneys ( X ± SE) 53.8 ± 7.8
Surviving B. knoxi ( X ± SE) 49.4 ± 4.6
EI% Kill ( X ± SE)
Abalone mortality
n=10 infested shells all treatments except control where n=5
EI%Kill means with shared superscripts are not significantly different (P>0.05)
Trial 2. Treatment of stock recently infested with B. knoxi (< 6-8 months) by drying
for four hours at 24 ° C
Treatment caused considerable reductions in total and mean recovered B.
knoxi and the estimated percentage kill was 96.8% (Table 5.5). The treatment effect
was statistically significant (Pearson Chi-square value 31.97, 1 df P<0.001). Of the
20 treated abalone live B. knoxi were recovered from 2 shells. One of these has 10 B.
knoxi chimneys from which 3 live worms were recovered. Most of the shells from
each group had small shell blisters (< 5% shell coverage) in the apex region. There
was no abalone mortality.
FRDC Final Report Page 45
Mudworm control in abalone
Table 5.5 Treatment of B. knoxi infestation by drying: 4 h, 24 °C
Mean ± SE Abalone
Recovered Recovered
n=20 each group, Pearson Chi-square value 31.97, 1 df P<0.001
Trial 3. Treatment of B. knoxi infected stock by drying at variable temperatures
Comparison by Chi-square analysis of dead and live worms after 4 hours of
drying at different temperatures indicated a significant treatment effect (Pearson chi-square value 48.26, 4 df, P<0.001). The most effective treatment at 21°C reduced the
mean B. knoxi count to less than 10% of the control count (Table 5.6). Estimates of
percentage worm kills were approximately 70% or greater for all treatment except thatat 24°C. The shells of stock exposed at 24 °C did not appear dry at the conclusion of
the exposure time. This was associated with the high humidity (71%) and did not
result in a treatment effect (Table 5.6). There was no abalone mortality during
treatment or in the week post air exposure. Shell blistering was minimal and generally
less than 5% when present.
Table 5.6 Treatment of B. knoxi infestation at variable temperatures
Temperature Total
Chimneys Recovered Recovered
n=21 all treatments, Pearson chi-square value 48.26, 4 df, P<0.001
Trial 4. Treatment of B. knoxi infected abalone by drying, variable exposure times
Chi-square analysis showed a significant treatment effect (Pearson Chi-square
value 30.27, 4 df P<0.001). Trends for recovered worm and estimated kill data
showed a potential treatment effect at 2 h and increasing with exposure time (Table
5.7). Only one B. knoxi in total survived the most effective 4 h exposure. The
relatively high value (33.3%) of the control EI%K indicates derelict B. knoxi burrows
were common. Mud worm blisters were small when present, covering less than 5% of
shell area. There was no abalone mortality during desiccation or in the 3 d post air
FRDC Final Report Page 46
Mudworm control in abalone
Table 5.7 Treatment of B. knoxi infestation at 21°C and variable exposure times
Chimneys Recovered Recovered
n=16 all treatments, Pearson Chi-square value 30.27, 4 df P<0.001
Trial 5. Air drying of old, heavily polychaete infested greenlip abalone
Despite the exposure time, temperature and humidity conditions (4 hours, 21 ±
0.5 °C, 60% humidity), the shells did not appear dry at the conclusion of treatment.
There was no statistically significant reduction in numbers of B. knoxi (Pearson Chi-
square value 1.27, df 1, P=0.260) and clearly no reduction in total polychaetes (Table
5.8). Control EI%K data indicated derelict B. knoxi burrows were common. There was
1 abalone mortality in the treated group during the recovery period. Polychaete worms
other than mud worms were very common, with 400 recorded in one treated shell.
The majority of the shells were seriously blistered in the range 10-50% blister
coverage, frequently with large walled off chambers.
Table 5.8 Efficacy of air drying for old heavily polychaete infested greenlip
B. knoxi chimneys ( X ± SE)
Surviving B. knoxi ( X ± SE)
EI% B. knoxi Kill ( X ± SE)
total worms ( X ± SE)
Abalone Mortality
n= 10 each group, Pearson Chi-square value 1.27, df 1, P=0.260 for B. knoxi.
Trial 6. Air drying of recently infected stock under field conditions
Drying treatment resulted in a mean reduction of B. knoxi from 3.3 to 0.4 per
shell under field conditions (16-18ºC, 49-62% humidity) (Table 5.9). The EI% Kill
value was very high at 87.5%. Analysis of surviving B. knoxi data by Chi square
analysis was significant (Pearson Chi-square value 64.2, df 1, P<0.001). Small
numbers of P. hoplura mud worms were present in the control but not in the treatment
group. This treatment effect was also statistically significant (Mann-Whitney U Test,
U = 120.0, P <0.01). There was no abalone mortality. Mean blister coverage was
4.3% (SD = 7.2%, n = 20)
FRDC Final Report Page 47
Mudworm control in abalone
Table 5.9 Field trial treatment of recent (< 3 month) mud worm infestation
EI%K B. knoxi
P. hoplura
n=20 for both groups, Pearson Chi-square value 64.2, df 1, P<0.001 for B. knoxi
Column means with shared superscripts are not significantly different (P>0.05)
Trial 7. Air drying of heavily fouled, P. hoplura infected stock under field conditions
Due to excess surface fouling the shells of many treated abalone did not appear
completely dry at the conclusion of air exposure (17.5-22ºC, 43-65% humidity).
Analysis of counts data by Mann-Whitney U Test showed the reductions in B. knoxi
and large P. hoplura between control and treatment groups (Table 5.10) were not
statistically significant (P>0.05). However, the reductions in small P. hoplura and
non-spionid polychaetes were statistically significant (U = 53.0, P=0.03 and U =14.5,
P<0.01 respectively). Non-spionid polychaetes were common and many appeared to
live on the surface of the shell rather than within it.
Abalone mortality data could not be assessed reliably in this trial because after
transport to the laboratory for mud worm quantification one control animal and
several in the treatment group died or were moribund. It was believed this was due to
transport stress and poor water quality in the holding tank. Additionally 10 of 20
abalone in the control group escaped from their holding cage after treatment but
before transport. Of the remainder, one abalone was excluded from statistical analysis
of count data as it contained > 100 P. hoplura (mostly post larvae), a level more than
twenty times the mean for the rest of the group. Mean blister coverage for the control
group was 20.4% (SD = 22.6, n=10).
Table 5.10 Field trial treatment of heavily fouled stock with 13 month exposure
to mud worm settlement
Small P .hoplura
Large P. hoplura spionid polychaetes
Control 1.1A ± 0.2
Treated 0.5A ± 0.02 0.3 B ± 0.02
n=20 treatment group. n=9 control group .
Column means with shared superscripts are not significantly different (P>0.05)
Trial 8. Two and half hour drying of recently B. knoxi infected stock
Although 40 abalone were assigned to each treatment group subsequent
analysis showed that less than half the animals had any B. knoxi infestation on the
basis of chimney counts. Table 5.11 displays data for the B. knoxi positive abalone
only. The 2.5 h air exposure(16-17ºC, 50-54% humidity) was highly significant in
reducing infestation (Pearson Chi-square value 30.22 df 1, P<0.001). Shell blistering
was rare and generally less than 5% coverage when present. There was no mortality in
either group (total n=80) in the week following treatment.
FRDC Final Report Page 48
Mudworm control in abalone
Table 5.11 Short air exposure treatment of three month B. knoxi infected stock.
Treatment
Mean ± SE 2.00 ± 1.61
Mean ± SE 1.38 ± 0.65
n=11 and 13 for control and treated groups respectively
Contingency table analysis: control group 23:0 (live worms: dead worms) and treatment group 3:15
(live worms: dead worms) Pearson Chi-square value 30.22 df 1, P<0.001.
Trial 9 Drying of 14 month mud worm infested blacklip abalone
Mud worm counts were reduced in air dried abalone compared to the control
animals for all worm categories. However, none of the reductions (Table 5.12) were
statistically significant (P>0.05, Mann–Whitney U Test) except for the category "total
small mud worms" (U = 30.0, P = 0.03).
Table 5.12 Treatment of 14 month mud worm infested abalone
Worm count ( X ± SE)
Small B. knoxi
Large B. knoxi
Total B. knoxi
Small P. hoplura
Large P. hoplura
Total P. hoplura
Total small mud worms
Non-spionid mud worms
Total polychaetes
n = 10 both groups
Row means with shared superscripts are not significantly different (P>0.05)
Trial 10. Repeat drying of abalone previously treated one year earlier
Drying resulted in lower counts for all mud worm categories (Table 5.13). These
were statistically significant by Mann-Whitney U Test for: total polychaetes (P=0.01),
large P. hoplura (P=0.01), small P. hoplura (P=0.03) and total P. hoplura (P=0.01).
Reductions were not statistically significant for large and total B. knoxi respectively
(P=0.10,and P=0.06). Insufficient small B. knoxi were present for a sensible test.
Mean shell blister coverage was 9.6% (SD=10.0%, n=19). One of the control group
animals was lost from the experiment before transport to the laboratory for
FRDC Final Report Page 49
Mudworm control in abalone
Table 5.13 Summer 1999 re-treatment of previously treated stock
Worm count ( X ± SE)
Small B. knoxi
Large B. knoxi
Total B. knoxi
Small P. hoplura
Large P. hoplura
Total P. hoplura
Non-spionid mud worms
Total polychaetes
n = 9 control group, n=10 treated group
Row means with shared superscripts are not significantly different (P>0.05)
Table 5.14 summarises the results of treatment trials reported above. The
efficacy of treatment column classes treatment as effective only if reductions in
worms were statistically significant
Table 5.14. Summary of trial effectiveness
Trial Infection
% Shell Treatment Efficacy of treatment
Blisters Time (h)
Effective for B. knoxi
Effective for B. knoxi
Effective for B. knoxi 15-21 °C
Effective for B. knoxi 2-4 hours
Ineffective for B. knoxi and non-spionids
Effective for B. knoxi and P. hoplura
Effective: B. knoxi, non-spionids, small P. hoplura
Ineffective: large P. hoplura
Effective for small B. knoxi
Effective for small mud worms only
Effective: for P. hoplura all sizes
Ineffective: for large B. knoxi
Effective treatment defined as statistically significant spionid reduction (P<0.05)
5.3.2 Drying and abalone mortality
Mortality of 15-20 mm stock dried at 21°C was minimal with no deaths for
control, 1, 2, 3 and 5 h air exposure treatments (Table 5.15). In the second experiment
there was no mortality in the 8 week observation period following air exposure for 11
h (Table 5.16). Growth was poor in all treatment groups including the non driedcontrol (0.89 mm ± 0.76 mm, 1.69 g ± 1.21 g; X ± SD, n=146). There was no
significant difference between SGR of treatments for length (F = 1.40, df 3,140, P=
0.246, ANOVA) or for weight (F =1.39, df 3,140, P = 0.249, ANOVA).
Tank conditions were not ideal during the recovery period. Temperatureextremes of 10-20 °C were recorded with a mean daily reading of 15.0°C (SD =2.1°C). Un-ionised ammonia readings of 0.25 mg.l-1 were recorded which might have
contributed to suppressed growth. One dead abalone was found to have a discoloured
FRDC Final Report Page 50
Mudworm control in abalone
lesion on the foot believed to have been a knife wound. There were a few escapes
from the experimental cages and/or holding tank. Feed consumption was
approximately 2% body weight.d-1. Of 30 abalone air dried for 15 h on 4-5 April
2000, most appeared moribund at completion of drying but subsequently recovered
with only 1 mortality in the 2 week recovery period.
Table 5.15. Mortality data: 1- 6 h exposure, 15-20 mm abalone
Cumulative mortality
Table 5. 16. Mortality data: 5-11 h exposure, 42 mm abalone
Cumulative mortality
5.3.3 Drying and long term growth
Forty-one tagged blacklip abalone (11 control, 30 air dried were recovered from
the grow out tank at farm 2. A mortality episode over the summer 1999/2000 reduced
abalone numbers generally and affected recovery of experimental abalone. There were
also 5 deaths in the air dried group in the week following treatment. There was no
significant difference between the groups in SGR for length (t=-0.69, df 39, p=0.497 t
-Test) nor for SGR weight (t=-0.43, df 39, p=0.669 t -Test). Growth in length (pooledtreatments) was 13.1 ± 3.4 mm and weight increment was 15.0 ± 4.9 g ( X ± SD, n =
41 both data sets).
At Tasmanian Tiger Abalone 62 of the hybrid experimental abalone (42 control,
20 air dried) were recovered 214 d after commencement of the trial. As at Marine
Shellfish Hatcheries an unrelated mortality episode at the farm significantly reduced
stock numbers in some tanks, including the tank housing the trial stock. There was no
significant difference in SGR of air dried and control stock for length (t=-0.77, df 59,
p=0.447 t-test) nor for SGR weight (t=-0.25, df 60, p=0.804 Unpaired t-test). Growthin length (pooled treatments) was 9.9 ± 2.5 mm (X ± SD) and growth in weight was5.3 ± 2.6 g (X ± SD).
At Huon Aquaculture 78 abalone (42 control, 35 dried) were recovered 210 d
post drying. Treatment group animals appeared stressed immediately after air
exposure, with limited clinging and turn over ability. However, there were no dead
animals in the weeks immediately following drying treatment. After 210 d there were
5 dead abalone in the control treatment and 3 in the air dried group. Mean length and
weight of control animals increased 4.0 mm (SD= 2.0, n=42) and 3.4 g (SD=1.5,
n=42) respectively. Mean length and weight increment for dried abalone were 3.2 mm
(SD=1.5, n=35) and 2.6 g (SD=1.8, n=35). This was a reduction of approximately 20
and 24 % for length and weight respectively compared to the control group.
Comparison of SGR by unpaired t–test found significant differences for length
(t=2.02, df 76, p=0.047) and weight (t=4.55, df = 75, p<0.001).
FRDC Final Report Page 51
Mudworm control in abalone
The results presented above relate to the original project objective No. 4 on
protocols for treatment within the production cycle. As noted previously the treatment
focus was shifted from chemical to air drying treatment when the former proved to be
ineffective. For this treatment, the objectives relating to abalone survival, growth,
cost, marketability have been met. The integration of the treatment regime into an
overall method to minimise mud worm infestation in line with objectives 1 and 6
(page 9) is outlined below.
The body of experimental work demonstrates that treatment of small B. knoxi
(and small P. hoplura) can be highly effective. In fact all treatment trials except No. 5
showed a reduction in numbers for all classes of worms between control and air dried
groups. These reductions were not necessarily statistically significant as summarised
in Table 5.14. Larger mud worms (> 5 mm and typically 10-25 mm) may be
susceptible to drying treatment depending on a host of factors. These appeared to
include: infestation time, blister severity, stock size and stock fouling. Increasing
infection period allowed mud worms time to become established in burrows deep
within the shell. Where larger chambers occurred within blisters survival appeared
especially likely. Non-spionid polychaetes were highly susceptible to drying treatment
in most trials where they were present. This may be because they tend to occur on the
surface of the shell rather than within it.
Appropriate conditions to ensure effective drying are considered to betemperatures >15°C and humidity less than approximately 63%. These conditions are
not uncommon on sunny days in Tasmania outside the period late autumn to early
spring. Where shells did not fully dry (Trials 5, 7 and one treatment in Trial 3)
treatment was ineffective. Lack of drying was associated with high humidity, larger
stock and severe shell fouling. The effect of humidity is clearly demonstrated bycomparison of results for B. knoxi survival dried at 24°C in trials 2 and 3. The
treatment was highly effective in the former at low humidity and ineffective in the
latter at higher humidity.
Previous work on treating mud worms in shellfish has focused on oysters.
Environmental treatments have included: fresh water soaking (Bailey-Brock and
Ringwood 1982, Nel et al., 1996 and Tonkin 1997), heated seawater (Bailey-Brock
and Ringwood 1982, Nel et al., 1996) and soaking in hyper saline water (Mackenzie
and Shearer 1959, Bailey-Brock and Ringwood 1982, Tonkin, 1997). The latter
method generally requires heating to dissolve sufficient salts. Leighton (1998)
successfully used elevated temperature to treat sabellid polychaete infection in two
tropical abalone species but this approach was unsuitable for the more commercially
valuable temperate species H. rufescens. Quantified studies on the efficacy of air
drying appear to be lacking although there are references to its use in oyster growing.
Nell and Smith (1988) note that 10-14 d out of water is a traditional treatment for mud
worms in Sydney rock oysters. This appears to be based on the work of Whitelegge
(1890). Fortuitously, desiccation is effective as a treatment for infected abalone at
much lower exposure times. In relation to this, observed blister morphology
differences between abalone and mud worm infested bivalve molluscs in Tasmania
are of interest. In bivalves B. knoxi is observed to inhabit very large water filled
blisters whereas in abalone blisters rarely have a significant volume. These
differences are quantified in section 5.5.3 (shell blistering section). Thus, blisters
typical of B. knoxi and to a lesser extent P. hoplura in abalone may provide relatively
poor protection against desiccation strategies.
FRDC Final Report Page 52
Mudworm control in abalone
Air drying treatment trials showed that mud worm infestation rates for lightly
infested abalone could be considerably reduced by air drying for 2-4 h. Post drying
recovery times of a week or so failed to show any abalone mortality in lightly infected
stocks. Longer air exposure and recovery times were used in follow up experiments
with the intention of defining a safe period for treatment air exposure. Abalone proved
surprisingly tolerant of air drying. There was no mortality of 15-20 mm stock dried
for up to 3 h and of 40 mm animals dried for 11 h, providing ample scope for effective
spionid treatment. This contrasts with poor survival for smaller abalone (5-10 mm) airdried in shade at 24°C (Whang and Chung 1977). Wells and Baldwin (1995) found
that larger abalone of two New Zealand species were "less susceptible to anaerobic
stress than smaller animals". The larger abalone conserved relatively more adenylate
energy charge and had lower concentrations of the glycolytic end products lactate and
tauropine. It was considered beyond the scope of the present research to establish the
point at which air drying abalone results in significant deaths of animals.
There was no difference in growth of control and air dried (5, 8, 11 h) abalone
54 d post treatment. However, growth was poor in the control group and temperature
fluctuation was a problem. Ammonia levels were potentially high enough to suppress
growth according to data of Harris et al. (1998). Thus the effect on growth of air
drying may have been masked by other factors. Certainly it would not be unexpected
if air drying resulted in a short term growth decrease. Edwards et al. (2000) has shown
that removal of abalone from the substrate with a knife is enough to depress the
growth rate over a two month period.
The series of three experiments on medium-long term post drying growth effects
produced conflicting results. Two trials showed no growth depression but the third
trial demonstrated growth depression of 20-25%. It may be significant that stock in
the trial showing reduced performance were growing slowly before the trial began and
untreated control animals continued to perform relatively poorly. The recorded growth
depression was similar to that reported by Shepherd and Hearn (1983) for wild
juvenile abalone handled in hot weather.
Given the potential for air drying treatment to suppress growth of stock this
management option should be used prudently with the emphasis on avoidance of
infestation. Where treatment is required, stress to abalone will likely be minimised by
early intervention, reduced drying time, reduced air temperature and reduced
differential between sea and air temperatures. Additionally, Watanabe et al. (1994)
found that starved abalone survived air exposure at considerably greater levels than
fed animals.
The use of air exposure as a mud worm treatment is considered a very
favourable outcome. The treatment is environmentally benign, uncontroversial
compared to potential chemotherapeutic treatments, and requires no withholding
period. Compared to any potential chemical treatments it minimises handling and thus
labour costs since it is difficult to conceive of a treatment protocol that does not
require removal of abalone cages from the water as an initial step. Additionally, there
are no consumable input costs associated with the treatment. Thus a potential
treatment option exists should farmed stock become infected. In susceptible areas the
treatment option should be secondary to and compliment avoidance strategies (section
5.1). Treatment if required should be exercised earlier rather than later to maximise
mud worm kill rates. Summer would appear the most appropriate time for treatment
as spionid settlement is largely completed and weather conditions are at their most
suitable for effective drying. Thus stock placed post November-January to avoid
spionid infestation can be treated if required approximately a year later if required and
FRDC Final Report Page 53
Mudworm control in abalone
should then remain mud worm free for the best part of another year before probable
5.4 Epidemiology/Risk factors
5.4.1 Effect of stock size
At Aquatas mud worm settlement as measured by the presence of
B. knoxi chimneys, B. knoxi worms and P. hoplura worms increased generally with
increasing stock size class (Table 5.17). The large size class was significantly
different (P<0.05, Kruskall-Wallis test) to the other size classes. Similarly, at Aquatas
the largest stock size acquired significantly more mud worm settlement by all
measures than medium and small stock size categories (P<0.05, Kruskall-Wallis
Test). Table 5.18 displays this data with replicates separate as P. hoplura data could
not be grouped in the medium size class (P<0.01 U = 0.0, Mann-Whitney U Test) and
because the small size class category was deleted from replicate 2 due to poor
recovery of animals.
Settlement of B. knoxi on experimental stock at both sites was considered low
with a maximum of approximately 8-12 chimneys per replicate of 5 large size class
abalone. Survival of B. knoxi worms until assessment in February – March was even
lower at about half chimney count levels (Tables 5.17 and 5.18).
Shell damage attributable to mud worms was significantly higher (P<0.05,
Kruskall-Wallis test) in the larger stock size class at both sites as measured by both
percentage blister cover and the SSDR methods (Table 5.19).
Table 5.17. Mud worm settlement on three size classes of abalone at Aquatas.
Means with standard error and n in parentheses.
B. knoxi chimneys
P. hoplura
Row means with shared superscripts are not significantly different (P>0.05) means are replicates of 5
Table 5.18. Mud worm settlement on three size classes of abalone at Huon
Aquaculture. Means with standard error and n in parentheses.
B. knoxi chimneys
P. hoplura
B. knoxi chimneys
P. hoplura
Row means with shared superscripts are not significantly different (P>0.05)
means are replicates of 5 abalone
FRDC Final Report Page 54
Mudworm control in abalone
Table 5.19. Shell damage assessment following spionid infestation for 3 size
classes of abalone (means with SE and n values in parentheses)
Row means with shared superscripts are not significantly different (P>0.05)
5.4.2 Spionid settlement and fouling organisms
Examination of recently formed B. knoxi chimneys showed that settlement did
not occur evenly over the shell surface but rather favoured certain sites. Although the
number of spirorbids and the surface area they occupied on abalone shells was
relatively small in the examined stock, 38% of B. knoxi chimneys occurred on or in
these fouling polychaetes (Table 5.20). Dead spirorbids were especially favoured with
the chimney often projecting from the tube opening. The most commonly settled part
of the abalone shell itself was the groove running around the base of the shell apex
(34% of chimneys). Chimneys were also found in shell irregularities such as shell
fractures, growth ridges and within respiratory pores, especially closed ones.
Observation in the period 1998-2000 showed spirorbid settlement occurred in the
spring and early summer in the south of the state.
Table 5.20 Distribution of B. knoxi chimneys on abalone shells
Location
Other fouling structure
Other shell location
As suspected bryozoan coated abalone had significantly fewer B. knoxi chimneysthan other abalone (P<0.01 U = 4.5, Mann-Whitney U test), 1.3 ± 0.5 compared to14.2 ± 3.1 respectively ( X ± SE). Bryozoans living on shucked abalone shells in a
recirculating holding system remained alive for over 6 months but did not appear to
spread nor did they colonise other nearby shells.
The quantitative spirorbid fouling experiment showed settlement of
B. knoxi was considerably higher on spirorbid fouled stock than on "clean" stock.
This was true for chimney count (P≤ 0.01, Mann - Whitney U Test) and surviving
worm data (P < 0.05, Kolmogorov –Smirnov Two-sample Test) where levels were 6-
10 times higher on spirorbid fouled stock at both study sites (Tables 5.21 and 5.22).
Note that basket replicates were not combined for Huon Aquaculture, as there were
significant differences (P<0.05, Mann-Whitney U Test) between B. knoxi chimney
data, B. knoxi worm data and SSDR for the "clean" group.
Settlement of P. hoplura was not as high as that of B. knoxi and there was no
significant difference (P>0.05, Kolmogorov-Smirnov Two-sample test) between
FRDC Final Report Page 55
Mudworm control in abalone
P. hoplura settlement on fouled and nominally clean abalone at Aquatas and the
second of the Huon Aquaculture replicates.
The differences in mud worm settlement (chiefly B. knoxi) between spirorbid
fouled and "clean" stock, led to higher levels of shell damage in the fouled stock as
described by the SSDR. There was an approximate 10 fold increase in mean SSDR for
fouled stock at Aquatas (P<0.01, U=125.5 Mann -Whitney U Test) and a 2-6 foldincrease at Huon Aquaculture (P ≤ 0.02, Mann -Whitney U Test, both replicates)
compared to nominally clean stocks (Table 5.23).
Table 5.21 Spionid settlement indicators for "clean" and spirorbid fouled
abalone stock at Aquatas. Means* with SE and n in parentheses
B. knoxi chimneys
P. hoplura
* means are replicates of 5 for B. knoxi and P. hoplura worm data
Row means with shared superscripts are not significantly different (P>0.05)
Table 5.22 Spionid settlement indicators for "clean" and spirorbid fouled
abalone stock at Huon Aquaculture. Means* with SE and n in parentheses
B. knoxi chimneys
P. hoplura
B. knoxi chimneys
P. hoplura
* means are replicates 5 for B. knoxi and P. hoplura worm data
Row means with shared superscripts are not significantly different (P>0.05)
Table 5.23 Subjective shell damage ratings for clean and spirorbid fouled
abalone at 2 study sites Means with SE and n in parentheses
Huon Aquaculture, Rep. 1
Huon Aquaculture, Rep. 2
Row means with shared superscripts are not significantly different (P>0.05)
The differences in shell damage between spirorbid fouled and "clean" stock
prompted the examination of growth between the 2 groups. Comparison of "clean"
and spirorbid fouled stock growth rates was performed separately for each replicate
(Table 5.24). Nominally clean stock grew significantly faster in replicate 1 for both
SGR length (t=2.49 df 41, P<0.01, one tailed t-test) and weight (t=2.73 df 23, p<0.01,
one tailed t-test) and for replicate 2, SGR weight (t = 1.80 df 36, P=0.04) but not for
SGR length replicate 2 (t = 1.21 df 40, P=0.12). As the value of n was low for a
reliable t-test in replicate 1 SGR weight comparison this was repeated as a Mann-
Whitney U Test (U = 12, P=0.01). Mean growth depression as a result of increased
shell blistering in spirorbid fouled stock was 28% (SD=8%, n=2) for length and 39%
(SD=19%, n=2) for weight.
FRDC Final Report Page 56
Mudworm control in abalone
Table 5.24 Comparison of growth (SGR) between "clean" and spirorbid
fouled stock. Means ± SD, n in parenthesis.
SGR length "clean"
0.054A ± 0.020 (24)
0.032A ± 0.016 (28)
0.036B ± 0.027 (19)
0.025A ± 0.015 (10)
SGR weight "clean"
0.226A ± 0.062 (5)
0.133A ± 0.049 (28)
0.108B ± 0.091 (20)
0.100B ± 0.055 (10)
Data pairs by replicate with shared superscripts are not significantly different
5.4.3 Long term spionid infestation and growth comparison
Abalone transferred August 1998 grew from 46.9 mm (SE=0.3, n=100) to 61.8 mm
(SE=1.1, n=23) and had acquired a mean spionid count of 54.1 (SE=5.9, n=23) by
October 2000. By contrast, smaller abalone transferred September 1998 grew from
18.8 mm (SE=0.1, n=150) to 57.6 mm (SE=0.62, n=94) by January 2001 acquiring a
considerably smaller mean spionid count of 1.2 (SE=0.2, n=64). Mean percentage
shell blistering was 28.7 (SE=2.9, n=23) and 1.9 (SE=0.5, n=64) in August and
September intake groups respectively.
5.4.4 Rearing vessel comparison
Comparison of worm numbers infesting Huon Aquaculture basket reared stock
sampled before and after the B. knoxi settlement season showed significant increases
for B. knoxi chimneys (P<0.01 U=0.0, Mann-Whitney U Test) and B. knoxi worms
(P<0.01 U=6.5), (Table 5.25). Count data for P. hoplura also increased significantly
between the sample times (P<0.01 U=7.0). By contrast, worm numbers infesting tube
reared stock at the site showed no significant increase for B. knoxi chimneys (P>0.05
U=39.5) or worms (P>0.05 U=39), (Table 5.26). Count data for P. hoplura increased
significantly between the two sample times (P<0.01 U=8.5).
Before the beginning of the B. knoxi settlement season at Huon Aquaculture
there was no significant difference between numbers of B. knoxi chimneys (P>0.05
U=30), B. knoxi worms (P>0.05 U=42.5), or P. hoplura (P>0.05 U=36) on abalone in
tubes and baskets (Tables 5.25 and 5.26). By completion of the B. knoxi settlement
period (sampled December for baskets and January for tubes) there was a significant
difference for B. knoxi chimneys and worms (P<0.01, U=0.5, 9.5 respectively)
between baskets and tubes. In the baskets the number of B. knoxi as indicated by
chimney counts and recovered worms increased approximately 5-6 fold during the
exposure period, where as in the tubes there was no increase in B. knoxi numbers.
There was no significant difference for P. hoplura (P>0.05 U=36.0, 41.0) between
baskets and tubes after the settlement season. Increase in numbers of this species were
greater than 10 fold in both baskets and tubes.
In the less infested basket reared set at Huon Aquaculture the trend was the
same as for the more infested basket reared stock at the site. There were significant
increases in counts of: B. knoxi chimneys, B. knoxi worms and P. hoplura worms
(P<0.01 for all; U=2.0, 5.5, 7.0 respectively), (Table 5.27)
As for data from the tubes at Huon Aquaculture there was no significant
increase in B. knoxi chimneys (P>0.05 U=45.5) or worms (P>0.05 U=49.5) for tube
FRDC Final Report Page 57
Mudworm control in abalone
reared stock at Aquatas (Table 5.28). There was a significant increase in P. hoplura
(P<0.01 U=16.5) as for tube reared stock at Huon Aquaculture.
Stock in the basket type rearing vessels at Aquatas had suffered heavy mortality
by early summer 2000 when they were resampled. Only B. knoxi chimney count data
was considered reliable as previously spionids numbers had been seen to decline
several months following the death of the host. Chimney structures were known to
survive the death of the worm by several months (section 4.5) and counts increasedfrom 1.7 ± 1.9 (mean ± SD, n=10) in September to 3.1 ± 0.4 (mean ± SD, n=39) in
January/February. This result was borderline statistically significant (P=0.05
Table 5.25 Mud worm progression for Huon Aquaculture abalone reared in
baskets: June 1999 - December 1999. Means ± SE
Time
B. knoxi Chimneys
P. hoplura
Column means with shared superscripts are not significantly different (P>0.05)
n= 10 both data sets
Table 5.26 Mud worm progression for Huon Aquaculture abalone reared in
tubes: August 1999 - January 2000. Means ± SE
Time
P. hoplura
Aug 99 5.0A± 1.0
Jan 00 4.6 A±1.2
Column means with shared superscripts are not significantly different (P>0.05)
n= 9 Aug 99, n = 10 January 2000 samples
Table 5.27 Mud worm progression for Huon Aquaculture less infested baskets:
June 1999- December 1999. Means ± SE
Time
P. hoplura
Column means with shared superscripts are not significantly different (P>0.05)
n= 9 Jun 99, n = 10 Dec 99 sample
FRDC Final Report Page 58
Mudworm control in abalone
Table 5.28 Mud worm progression tube reared stock at Aquatas:
August 1999-January 2000. Means ± SE
Time
P. hoplura
Aug 99 1.4A ± 0.4
Jan 00 1.7A ± 0.8
Column means with shared superscripts are not significantly
different (P>0.05) n=10 both groups
5.4.5 Position in water column
There was no significant difference between the 6 and 9 m depth abalone for
B. knoxi chimney and worm counts (P>0.05, Mann-Whitney U-test). Counts of
P. hoplura, total spionids and subjective shell damage rating (SSDR) were
significantly different (P<0.05, U =117, 124 and 129 respectively, Mann-Whitney U-
test) between the 6 and 9 m treatments (Table 5.29). Spionid settlement was very low
overall but approximately 4 times higher for P. hoplura at 9 m (representing a
minimum 1 m off the sea floor) compared to 6 m. The 3 m depth baskets were lost
before completion and Table 5.29 provides an estimate based on the sum of 1 month
samplings during the same time period used for determining settlement timing
(section 5.1).
Table 5.29 Comparison of spionid settlement and impact at two positions
in the water column. Means ± SE
Depth
P. hoplura
0.35 A ± 0.21 0.45 A ± 0.21
1.15 B ± 0.30 1.05 B ± 0.22
Means in columns with shared superscripts are not significantly different (P>0.05)
n=10 samples for all means, each consisting of a replicate of 10 abalone
* data presented as mean of replicate of 10 abalone for comparison purposes no statistical variation
data available.
5.4.6 Comparison of abalone species
Spionid settlement was very low with a maximum mean of 0.2 (SE = 0.09, n=20)
P. hoplura per replicate of 10 abalone for H. laevigata. This was not significantlydifferent (P>0.05, U=170 Mann-Whitney U-test) to data for H. rubra (0.05 ± 0.05,
X ± SE, n=20). No B. knoxi settlement was detected in either group.
Data presented above and discussed below relate specifically to project objective
No. 5 on epidemiology of mud worm infestation and in part to objective 3 on
interactions between mud worm reproductive behaviour and abalone. These findings
contribute to the minimisation of mud worm risk as per objectives 1 and 6 (page 9).
FRDC Final Report Page 59
Mudworm control in abalone
Examination of B. knoxi chimney locations revealed that settlement was not
random on the surface of the abalone shell but favoured certain areas. The apical
groove was a favoured site and this was consistent with the observation that B. knoxi
shell blisters were common in the ventral shell apex region. Fouling organisms with
calcareous shells such as spirorbids and to a lesser extend Pomatoceros sp. and
Pacific oysters were also targeted by settling B. knoxi larvae. It is unclear whether
larvae target these areas because they are relatively easy to penetrate or because they
provide shelter like the apex groove and other "shell irregularities". Zottoli and
Carriker (1974) noted that P. websteri larvae settle in crevices present in oyster shells.
Certainly the tubes of dead spirorbids would appear to provide an instant shelter for
settling B. knoxi larvae.
The presence of B. knoxi has previously been recorded in some of these fouling
organisms in New Zealand. Read (1975) noted the presence of the species in "serpulid
tube masses" and Handley (1997) recorded the presence of B. knoxi in Pomatoceros
sp and Pacific oysters.
The higher rate of settlement on the spirorbid fouled, relative to the "clean" group
of experimental animals has implications for abalone culture in B. knoxi susceptible
areas. Although the extent of fouling in the spirorbid positive group was high for
animals in land based systems during the study period it was not remarkably for
abalone transferred to sea based study sites for a period of time. As spirorbid
settlement was observed mainly in the spring months, post spring abalone transfer
should result in relief from the spionid enhancement effect associated with spirorbids.
Simultaneous transfer of 3 different sized stock groups resulted in significantly
higher spionid infestation of the largest group (mean length 51 mm) following a single
spring/summer spionid settlement season. Interestingly, abalone transferred at less
than 20 mm attracted little spionid settlement initially and subsequently for two years
while growing to in excess of 50 mm. By contrast, abalone initially stocked at 47 mm
and spirorbid positive acquired greater than 50 spionids per animal after two years in
the sea. The shell location settlement data and this size/growth data suggest that
spionid settlement is not simply passive and could be influenced by spionid
The significance of stock size on mud worm settlement has rarely been
mentioned in cultured mollusc mud worm studies. One exception is Handley (1997)
who suggested that small oyster stock were not attractive for settlement as a possible
explanation for their failure to become infected with B. knoxi. Studies of wild abalone
and mud worm have also shown increasing infestation with increasing size (Kojima
and Imajima 1982, Clavier 1989). The former authors found a minimum shell length
of 29 mm before H. diversicolor became infested with Polydora sp. The incidence
and severity of infection increased with increased abalone shell length.
The pattern of mud worm infestation over a B. knoxi settlement period differed
between rearing container types at both study sites. Infestation levels of the mud
worm species P. hoplura increased in both tube and basket type rearing vessels by a
factor of approximately 10. In contrast, B. knoxi chimney and/or worm counts
increased significantly in basket type but not in tube type rearing vessels at both sites.
Dissection of spionid blisters showed that P. hoplura produced brooded
(lecithotrophic) larvae from nurse eggs (section 5.1). Such larvae leave the maternal
burrow at a relatively large size and may omit a planktonic swimming stage (refer to
reviews by Woodwick 1977, Skeel 1979, Radashevsky 1994). On several occasions
P. hoplura larvae were observed to form new burrows adjacent to the maternal
burrow. It is plausible therefore that the increase in P. hoplura numbers in all rearing
container types was substantially driven by reproduction of worms present in infested
FRDC Final Report Page 60
Mudworm control in abalone
stocks. In contrast to the strategy of P. hoplura, B. knoxi in Tasmania appears to
produce only planktonic larvae as previously reported by Handley (1997, 2000) in
New Zealand. Such larvae spend 2-3 weeks in the plankton after release fromcapsules at a relatively small size ( 500 µm). Therefore, by the time such larvae areready to settle (1500-2000 µm) they may have been moved considerable distances by
currents and wave action.
The smaller mesh area on tube type rearing containers may reduce the probability
of B. knoxi larvae from the outside gaining access to the stock, relative to the more
open basket type culture vessels. Thus B. knoxi numbers increased in the more easily
accessed stocks and remained static in the more sheltered stocks. Escape of hatching
planktonic larvae from rearing containers may be explained as an active process since
such larvae were observed to be strongly phototaxic as previously noted by Handley
(1997). They would therefore be expected to swarm towards the light emitting mesh
areas of the tube type rearing containers and swim out and up towards the surface.
Thus mud worm settlement on abalone may depend on the interactions between
reproductive strategy of the polychaete species and the effective water exchange of
the containment vessel. Whether restriction of water flow in abalone rearing
containers as a means of risk reduction for B. knoxi infection is a worthwhile strategy
is unclear. There must be a point where restriction of water flow is detrimental to
abalone health. However, with respect to cleaning of rearing vessels or transfer of
stock from fouled to clean containers it may be worth considering the timing of such
events in relation to B. knoxi settlement.
Spionid settlement data showed P. hoplura infestation was greatest 9 m from
the surface, or more importantly 1 m from the bottom. Greater sediment can be
expected near the bottom and this has been considered in the past to have a role in
spionid infestation of oysters. Nell and Smith (1988) state that some oyster growers in
NSW spray mud from oysters as this is considered to reduce the risk of mud worm
infestation. Following experiments on intertidal oyster culture and mud worms,
Handley and Bergquist (1997) recommended that oysters be grown at least 50 cm
above the bottom to optimize spionid avoidance. Likewise Caceres-Martinez et al.
(1998) found placement of oysters away from the bottom may reduce the prevalence
of Polydora sp. Pregenzer (1983) found that spionid infestation (which included
P. hoplura) of mussels was greatest on the bottom and in silty areas.
At some culture facilities empty shells and feral Pacific oysters may be found on
the bottom and could serve as hosts for P. hoplura. The limited dispersal ability of
P. hoplura may therefore increase the risk of colonisation in near bottom reared
stocks at some locations. By contrast, B. knoxi with a long planktonic larval phase has
greater scope for dispersion. As in the present study, Handley (1997) found no
difference in B. knoxi settlement rates in oysters at two depth treatments.
Differences in respiratory pore size and shell sculpturing did not result in
differential spionid settlement between H. rubra and H. laevigata. This was possibly
due to the very low rate of spionid settlement generally in the experiment.
In summary, Larger stock ( 50 mm) attracted greater mud worm settlement and
subsequent shell damage than stock of 35 mm or smaller. Likewise spirorbid fouling
was found to enhance spionid settlement, especially that of B. knoxi. Thus if there is a
requirement to transfer stocks to spionid susceptible sea based sites during or soon
before mud worm dispersal periods the use of smaller and relatively spirorbid free
animals would minimize risk. It would be prudent to place larger abalone after the
completion of the spring settlement period. Stock growth rates and cost structures for
sea based sites will determine whether individual farms use these sites for all or part
of the grow-out phase. The settlement intensity of B. knoxi was seen to vary
FRDC Final Report Page 61
Mudworm control in abalone
considerably depending on the design of the abalone containment vessel. Settlement
of P. hoplura but not B. knoxi was greater on abalone reared near the bottom than
higher in the water column. Thus the design of rearing vessels, especially with respect
to water flow and their placement in the water column has implications for farming of
abalone in mud worm susceptible areas.
5.5 Abalone health I: mortality, growth & condition
Generally, there was little mortality that could be attributed to spionid mud
worm infestation during field studies 1998-2001. Approximately 9000 abalone were
transferred to the study sites for experimental work detailed in sections 5.1-5.4 and the
present section. Of these only a few hundred animals became heavily mud worm
infested. The two most heavily infested cohorts provided a contrast in mortality data.
At Aquatas the heavily infested August 1998 intake group experienced
mortality of 48 of 61 (79%) remaining abalone between November 1999 and June
2000. Whereas, in the similarly infested, same intake time cohort at Huon
Aquaculture there were 3 (from initial pool of 200) abalone deaths between May 1999
and Feb 2000 featuring severe blistering. In the minimally mud worm infected spring
1998 intake at Huon Aquaculture there was a 7% mortality rate over the length of the
5.5.2 Growth
Growth was generally poor by commercial abalone culture standards as shown in
Table 5.30. The August 1998 intake at Aquatas that experienced considerable
mortality showed a mean reduction in weight over the course of the study. Growth
data is presented in more detail below for those groups where there was a significant
degree of mud worm infestation.
August 1998 Cohort 1 Huon Aquaculture (long term treatment comparison)
Growth comparison between mud worm infested stock and the matched group
treated by drying to reduce infestation levels is shown in Figure 5.1. At the time of
treatment (December 1998) there was no significant difference in length of the 2
groups but by July 1999 the air dried group were significantly longer (P<0.01, t=-
2.79, df 152, two-tailed t-test). Based on group mean comparisons treated stock grew
at 57 microns.day -1 compared to 44 microns.day -1 for untreated stock. This is a
growth depression of approximately 20% attributable to the level of mud worm
infection present in the time period . As seen in Figure 5.1 growth of both treated and
untreated abalone was poor in this intake cohort after July 1999. Between July 1999
and October 2000 both groups grew only another 3 mm corresponding with a
reduction in feeding frequency from approximately weekly to twice monthly.
August 1998 Cohort 2 Huon Aquaculture.
This group was reared in "tube type" vessels throughout the length of the study.
As seen later, this group was subject to very high levels of mud worm infestation
including shell blistering and grew from approximately 46 to 67 mm in over 2 years
(Table 5.30).
FRDC Final Report Page 62
Mudworm control in abalone
Table 5.30 Size and growth data for abalone at three farm sites, mean ± SD (n)
Huon Aquaculture Company
Initial length (mm)
46.2 ± 3.1 (100)
18.8 ± 1.4 (150)
42.0 ± 6.1 (100)
28.2 ± 3.2 (100)
31.5 ± 4.3 (172)
Initial weight (g)
15.4 ± 2.8 (100)
14.7 ± 2.9 (100)
10.7 ± 4.5 (100)
Final length (mm)
36.0 ± 10.1 (23)
46.7 ± 13.0 (49)
25.0 ± 11.4 (88)
Growth: length (µ.d-1)
Growth:weight (mg.d-1)
SGR length
Huon Aquaculture August 1998 intakes: (1) = control group from long term mud worm treatment experiment, (2) = stock reared in "tube" type containers
Site 3 (East Coast)
Initial length (mm)
46.9 ± 2.7 (200)
39.1 ± 4.3 (100)
26.1 ± 3.6 (100)
Initial weight (g)
15.7 ± 2.8 (200)
9.5 ± 3.7 (10) *
Final length (mm)
Growth: length (µ.d-1)
Growth:weight (mg.d-1)
SGR length
* = First Site 3 weight data taken June 6 2000, later than first length data.
FRDC Final Report Page 63
Mudworm control in abalone
Length (m
Figure 5.1 Length comparison for mud worm treated and untreated abalone.
Huon Aquaculture August 1998 intake (1). Means ± SE.
Growth of other abalone intake time cohorts
The spring 1998 cohort at Huon Aquaculture Company was stocked at
approximately 20 mm or less and approached market size ( 60 mm) about 2 years
later in early January 2001 (Table 5.30). Specific growth rates (Table 5.30) were
similar for the spring 1998, December 1999 and April 2000 cohorts at Huon
Poor growth of stock at Aquatas generally was linked to increasingly
infrequent feeding in the second half of the project. Stock sampled at Site 3 (East
Coast) grew faster than stocks at the other study sites (Table 5.30). All 3 sites used
the same sources for spat and feed. Aquatech trays were used to grow the stock at
Site 3 and for some intake cohorts at Aquatas and Huon Aquaculture.
5.5.3 Shell blistering and spionid counts
Shell blistering was most common in the apex area of the shell, with blisters in
the vicinity of the respiratory pores the second most common. (Table 5.31). Blisters
caused by B. knoxi worms were located overwhelmingly in the shell apex area (63%)
contrasting with P. hoplura blisters that tended to be more evenly spread around the
host shell (Table 5.31).
FRDC Final Report Page 64
Mudworm control in abalone
Table 5.31 Blister location by spionid species
Blister location
Mixed spionids B. knoxi
P. hoplura
Respiratory pores
Early mud worm blisters were flat and yellow in colour, a result of initial
conchiolin deposition (Blake and Evans, 1972). Actively growing B. knoxi blisters
tended to be amber to light brown in colour and were frequently located in the shell
apex as indicated by Table 5.31. Eventually such blisters usually developed a thick
coat of nacre over them contrasting with the soft, non-healed blisters associated with
the initial mortality reports.
There was considerably variability of blister location with P. hoplura infestation
including damage to the leading edge, respiratory pores, apex and other areas. With
time P. hoplura blisters became dark brown/ black in appearance unless substantial
shell nacre was deposited over them. Older blisters of both spionid species were
raised up to 5 mm from the base of the shell. Long-term infested abalone usually had
a mixture of spionid species and corresponding damage to the shell. A range of shell
blistering is presented in Figure 4.6 (methods section).
The volumes of 10 representative large blisters from the apex area of the shell
were measured. These blisters, caused by B. knoxi and had volume range of 0.1 to 0.6
ml. By contrast the volume of a sample of large B. knoxi blisters measured in Pacific
oysters was an order of magnitude greater, with one oyster recording a volume of 10
ml. Histological cross sections of shell, including blisters, mud worms and blister
detritus are shown in Figures 5.2 and 5.3.
August 1998 cohorts
In cohort 1 at Huon Aquaculture shell blistering was detected September 1998,
one month after transferto the site (Figure 5.4). "Active" category blisters (section
4.9) dominated samples for the first few months but by 1999 blisters assigned to the
"healed" category were common, indicating nacre was being deposited. By October
2000 shell blistering in the untreated control stock had reached approximately 30% of
shell area (Figure 5.4). This was comparable blister coverage to that associated with
the initial abalone mortality episodes of the mid 1990's (introduction). Comparison of
% total blister coverage in treated stock (air dried in December 1998 and 1999) and
control untreated abalone is shown in Figure 5.5. REML analysis with time and
treatment as factors was highly significant (P<0.001) for both factors. Untreated stock
were significantly more blistered than treated stock from the same time sample in
March and October 2000 (Figure 5.5).
FRDC Final Report Page 65
Mudworm control in abalone
Figure 5.2 Normal abalone shell structure, O = outside of shell,
Bar = 100 µm
Figure 5.3 Cross-section of abalone shell honey combed with spionid burrows.
SL = disrupted shell layers, BU = spionid burrows, S = spionid in cross-section.
Bar = 500 µm
FRDC Final Report Page 66
Mudworm control in abalone
Figure 5.4 Progression and nature of shell blistering Huon Aquaculture August
Intake cohort 1 (untreated control abalone) means ± SE
blister cover
Figure 5.5 Mud worm blister comparison for treated and untreated abalone
Huon aquaculture August 1998 intake (means ± SE). * denotes significantly
different data Pairs (P<0.05).
FRDC Final Report Page 67
Mudworm control in abalone
Subjective blister data showed the maximum SSDR value of "3" was recorded
in samples from untreated stock in 2000 but not in previous years . The treated
abalone recorded no Grade 3 SSDR scores and contrasting with the untreated stock
recorded some zero SSDR scores during 2000. Mann-Whitney U Test analysis of
SSDR data for October 2000 (final sample) showed a significant difference between
the treated and untreated stock (P<0.01, U=196).
Spionids were present in abalone shells within a month of transfer and mean
counts were approximately 50 per shell by May 2000 (Figure 5.6). This level was
previously associated with stock mortality (background, section 1.3). However, a
dominance of B. knoxi worms were previously recorded in the shells of dead and
dying abalone at the site, contrasting with this study where P. hoplura dominated
numerically (Figure 5.6). Air drying treatment (December 1998 and 1999)
significantly reduced mud worm counts over time (Figure 5.6). Two-way ANOVA
performed on log transformed data with time and treatment as factors was highly
significant (P<0.001 for both factors and for both spionid species). Counts of B. knoxi
in untreated stock were approximately five times higher than in air dried stock over
the duration of the study. This difference was statistically significant at every time
sample (P<0.05). Similarly, counts of P. hoplura were significantly (P<0.05) higher
in untreated stock at all sample times except June1999. Spionids of both species
increased markedly in number in untreated abalone post June 1999 after the second
spring/summer mud worm reproductive period. Treatment efficacy data for the 2
drying periods are reported in section 5.3.1 (Trials 6 and 10).
In the second August 1998 intake cohort at Huon Aquaculture low level shell
blistering was also detected a month after transfer to the site. Blisters categorised as
"active" dominated during the first spring until autumn the following year (1999).
Mean total blister coverage reached approximately 35% by January 2000 and
remained at this level until final sampling in November 2000 (Table 5.32). There was
a significant inverse relationship between % total blister and both final length (Figure
5.7) and whole weight of abalone (df 1 47, P<0.001 for both). Full regression
analysis summaries including estimates of parameters and significance of r values are
shown in Appendices 5P and 5Q.
The pattern of spionid infestation in cohort 2 at Huon Aquaculture was similar
to that of August 1998 cohort 1 untreated control stock until early 2000. By
November 2000, however, total spionid numbers had increased in cohort 2 from
approximately 50 to 160 worms per abalone (Table 5.32). Approximately one third of
P. hoplura were juveniles no more than 5 mm long and considered less than 3 months
in age. Count data showed that P. hoplura was more common than B. knoxi in both
August 1998 cohorts at this site, but more so in relative and absolute terms in cohort 2
compared to cohort 1 (Table 5.32). This was attributed to an interaction between
spionid reproductive strategy and rearing container type (section 5.4.4).
FRDC Final Report Page 68
Mudworm control in abalone
Polydora hoplura
post 2 nd treatment
post 1 st treatment
Boccardia knoxi
post 2 nd treatment
Figure 5.6 Mud worm counts for Huon Aquaculture August 1998 intake (cohort
1) treated and untreated abalone (means ± SE, n values equivalent for each data
pair unless indicated Sample time data pairs marked with an * are not
significantly different (P>0.05).
FRDC Final Report Page 69
Mudworm control in abalone
Table 7.2 Final % blister coverage and spionid count data for abalone at three farm sites, mean ± SE (n)
Huon Aquaculture Company
0.1 ± 0.1 (10)
P. hoplura
163.5 ± 10.0 (49)
5.2* ± 1.7 (10)
165.6 ± 8.3 (49)
Huon Aquaculture August 1998 intakes: (1) = control group from long term mud worm treatment experiment, (2) = stock reared in "tube" type containers
* most worms newly settled < 3 mm
Site 3 (East Coast)
P. hoplura
FRDC Final Report Page 70
Figure 5.7 Total blister coverage versus length for Huon Aquaculture August
1998 (intake 2) abalone
Figure 5.8 Progression and nature of shell blistering Aquatas August 1998
intake. Means ± SE, n=10 unless otherwise specified. * = sample of "dead" shells
FRDC Final Report Page 71
At the Aquatas site, blistering of the August 1998 intake abalone increased
steadily with little nacre present on blisters in the first year. Maximum blister
coverage exceeded 25% in March and April 2000 as stock mortality was recorded
(Figure 5.8). Maximum recorded blister levels were not significantly different
(U=116, P>0.05, Mann-Whitney U-test) between similarly reared August 1998 intakecohorts at Aquatas (30.3% ± 9.0, 14) and Huon Aquaculture (28.7% ± 2.4, 23), ( X ±
SE, n). Final samples from Aquatas had a low proportion of healed blisters (<50%,
Figure 5.8) compared to the comparable Huon Aquaculture samples (Figure 5.4). The
subjective shell damage rating system indicated a rapid decline in the proportion of
zero rated (blister free) shells in the first 6 months after transfer. Rating 2 (moderately
blistered) shells dominated by the late spring 1999 and a small proportion of severe
blister ratings (SSDR = 3) occurred in shell samples post November 1999.
Spionid counts were significantly lower (U=0.0, P<0.01 Mann-Whitney U-test)for the last Aquatas sample in June 2000 (6.5 ± 1.3, n=10) compared to a similar(May 2000) Huon Aquaculture sample (56.4 ± 8.6, n=5), ( X ± SE). Spionid counts
were generally <10 per sample for this intake at Aquatas (Figure 5.9), although as
previously indicated maximum blister levels were similar to more infested stock at
Huon Aquaculture.
Data for other intake times
Spionid infestation and subsequent shell blistering was minimal in stocks
transferred to Huon Aquaculture and Aquatas in the spring months of 1998, 1999 and
December 1999 (Table 5.32). After exposure to 3 successive spring/summer spionid
dispersal periods the Huon Aquaculture spring 1998 intake had <2 spionids per
abalone by January 2001 (Table 5.32). Significantly less, (U=0.0, P<0.01 Mann
Whitney U-test) than the lesser infested of the 2 August 1998 intakes (Table 5.32).
Mean blister coverage was similarly low at <2% by January 2001. This group grew
from 18 to 58 mm during the study period (Table 5.30) but spionid infestation did not
increase significantly with size. Placement of abalone at study sites in December 1999
to avoid presumptive B. knoxi settlement (section 5.1) was successful at Huon
Aquaculture with no worm of this species recorded by January 2001. Similarly no
B. knoxi was recorded at Aquatas up to and including April 2000, following which
time the baskets were lost (Table 7.2).
Stock present at Site 3 on the east coast of Tasmania were sampled 7 times
(n=10 per sample) between April 2000 and January 2001. Both
B. knoxi and P. hoplura were present in the cumulative total of 4 adult spionids and
10 recently settled larvae. Only 1 mud worm blister, covering 10% of the shell was
FRDC Final Report Page 72
P. hopluraB. knoxi
Figure 5.9 Temporal change in spionid count and species mix in August 1998
abalone intake at Aquatas. Means + SE, n=10 unless otherwise shown.
Figure 5.10 Percentage flesh weight comparison: Treated and untreated abalone,
August 1998 intakes at Huon Aquaculture (means ± SE, n values shown.) Data
pairs denoted with an * are significantly different (P<0.05).
FRDC Final Report Page 73
5.5.4 Measures of abalone condition
Preliminary assessment of various condition indices was made to ascertain the
most suitable for long-term health monitoring. Regression analysis showed there was
a highly significant relationship between weight and CILENGTH (df 1 41, P<0.001) with
the condition index increasing with weight of stock. Further, the dry weight index
CILENGTH increased as a function of shell length (df 1 41, P<0.001).Thus, as CILENGTH
increased with stock size it was unsuitable for long term-term studies where growth
was expected. This was also true of the relationship between percentage dry flesh
weight data and length (df 1 41, P=0.006) and weight (df 1 41 P=0.039).
By contrast, there was no significant relationship between length and percentage
wet flesh weight (df 1 41, P=0.401) and weight and percentage flesh weight (df 1 41
P=0.361), indicating % flesh weight was independent of these measures of animal
size. In both regressions the residual variance exceeded the variance of the response
variance (percentage flesh weight) and no r-squared value could be calculated.
Regression analysis of shell length and CIWEIGHT was also not statistically
significant (df 1 41, P=0.073) and nor was that between whole wet weight and
CIWEIGHT (df 1 41, p=0.107). Although the probability values for the likely hood of a
significant relationship between CIWEIGHT and the two measures of abalone size were
>0.05 they were much closer to being statistically significant than those of percentage
flesh weight and abalone size, measured by weight and length. Thus percentage flesh
weight was felt to provide a better measure of relative "fleshyness" or condition than
either dry weight condition index.
A normal range for percentage flesh weight data was established by calculating
means for 24 samples of 10 abalone between 20 and 70 mm in length. Mean
percentage flesh weight was 71.1% (SD=2.1%, n=24). By contrast, an assessment of
% flesh weight on remnant stock infested with mud worm in the mid 1990's and held
at Tasmanian Tiger Abalone showed mean % flesh weight of 52.6 (SD=3.1%, n=10).
These abalone had blistering to approximately 25% of their shells with approximately
one third having the maximum SSDR of 3. Data for abalone transferred and spionid
infested during 1998-2001 is presented below.
Huon Aquaculture August 1998 cohort 1
Two-way ANOVA after arcsine transformation of % flesh weight data found
P values of <0.001 and 0.007 for time and treatment as factors respectively. Air dried
stock samples were approximately 3 percentage points higher than those of non-
treated control stocks following the initial December 1998 treatment episode (Figure
5.10). This differential was maintained throughout the length of the study. Percentage
flesh weight of treated and untreated stock fell from peak values in May 1999 (Figure
5.10). This coincided with decrease in growth and increases in blistering and mud
worm counts for both air dried and untreated stock. The final sample value for the
untreated control stock was 61.2% (SD=4.3, n=23), significantly lower (P<0.01,
U=18.0, Mann-Whitney U Test) than the mean for the established normal range of
71.1% (SD=2.1%, n=24).
FRDC Final Report Page 74
Data on spionid impacts affecting abalone health relate to project objective 2
on interactions between mud worms and abalone and in part to objective 3 on long
term efficacy of drying treatment.
Early pilot scale, sea based abalone grow out ventures in southern Tasmania
were severely compromised by stock mortality associated with high spionid mud
worm counts and shell blistering. With one exception, in the present study, there was
little evidence of mud worm related mortality in stock transferred to 2 sites with a
previous history of severe infestation. Some stock were exposed to mud worms for up
to 27 months, acquired in excess of 50-150 worms and blistering to approximately
one third of the shell area yet did not suffer the mortality episodes previously seen at
Stock mortality occurred in one intake time group (August 1998) at one site but
not the other. Shell blister damage levels were similar in this intake time group at
both sites but there was steady, albeit, slow growth of the stock at one site but not the
other. At the latter site stock mortality began approximately 18 months after initial
stocking and subsequent spionid colonisation. Growth data indicated suppressed
growth at the Aquatas site generally and this was considered to be largely due to
relatively infrequent feeding. However, relatively poor nutrition alone, in the absence
of significant spionid infestation did not result in mass stock mortality. Thus it
appeared that high levels of shell blistering in addition to poor nutrition resulted in
stock mortality. Whereas, high levels of spionids and shell damage, in the presence of
adequate nutrition did not result in significant mortality at Huon Aquaculture.
The effect of spionid infestation on growth rate of abalone has not been
addressed in previous studies on wild stocks but is of considerable interest to the
grower. The comparison between spionid treated (air dried) and infected control
abalone (August 1998, Huon Aquaculture cohort 1) showed that the air dried stock
grew significantly faster in the 6 months post treatment (to June 1999). The
suppression of the growth rate in the untreated infected stock was estimated at 20
percent. Maximum spionid infestation associated with this growth depression was 4
worms per abalone corresponding to blister coverage of 7%. Growth depression was
also seen in section 5.4 where spionid infestation with 3-5 worms and subsequent
blistering in spirorbid fouled stock caused a mean 28% reduction in shell growth
compared to minimally infested control stock. Regression analysis on heavily infested
abalone (Huon Aquaculture, August 1998 intake cohort 2) showed that size of
abalone defined as both length and weight declined with increased blister cover.
Of the many stock cohorts placed at the study sites for temporal health studies
(reported in this section), risk factor analysis, or larval dispersal experiments only the
August 1998 intakes became heavily blistered. Blister coverage of approximately
30% was seen in the present study as in the previous abalone mortality episodes that
led to this research. However, while blister coverage levels were similar, SSDR data
from remnant stock surviving the original mortality episode until 1997-1998
(Appendix 3,1B) suggested the original shell damage episodes were more severe.
Those previously seen blisters were often very soft consisting of 5 mm or more of
apparent conchiolin material over the ventral surface of the shell. Blisters of this
nature were absent during the course of the present study and the maximum SSDR
score was rarely used. Large blisters in the present study were usually covered with a
hard layer of pearly nacre. Possibly factors such as nutrition could account for some
of the differences between blisters in past and present episodes. Certainly, in Australia
FRDC Final Report Page 75
considerable research and development effort has gone into the area of formulated
abalone diets since the original spionid outbreaks.
Blisters formed by B. knoxi worms were frequently located in the shell apex.
This was consistent with the finding (section 5.4.2) that chimney structures created by
this species were often located in the groove under the shell apex. It would therefore
appear that B. knoxi is capable of burrowing directly through the shell of an abalone.
Blake and Evans (1972) in reviewing mode of spionid blistering noted that two routes
of host invasion were reported. In the first the larvae swim into the mantle cavity or
burrow between the shell edge and the mantle. In the second spionid larvae settle on
the outside and penetrate directly. This latter method is undoubtedly favoured by
B. knoxi with initial settlement of the apical groove consistent with the report of
Zottoli and Carriker (1974) that P. websteri larvae settle wherever there are crevices
in oyster shells. The respiratory pores of abalone appear to be another favoured site
for spionid settlement. Whether this is solely because they present a shell irregularity
and thus a degree of protection or because spionid larvae actually enter the mantle
cavity by this means is unclear. There is no doubt P. hoplura larvae can enter the
abalone shell through the leading edge but the diversity of blister locations suggests
they may also be capable of burrowing directly through the shell. Some second
generation larvae appear to never leave the shell (section 5.1) creating new burrows
adjacent to the maternal blister.
As noted earlier, the majority of the different intake time cohorts suffered
negligible spionid infestation. The spring 1998 intake at Huon Aquaculture, stocked
at 18 mm was exposed to 3 full or partial spring/summer mud worm settlement
seasons and acquired an average of only 1 spionid per abalone. Interestingly, this
group was minimally infested after the first spring exposure and remained that way
despite subsequent growth to 58 mm (Table 5.30). The only stocks to become heavily
spionid infested were the August 1998 intakes at both study sites. These animals have
since been shown to be at higher risk due to some initial fouling with spirorbids, shell
irregularities and greater stocking size. These data may give industry some confidence
that the events of the mid 1990's were not typical.
Of the five mud worm species found to infest stock in the present study only
B. knoxi and P. hoplura were present in sufficient numbers to be considered pest
species. Interestingly, P. hoplura was far more numerous than B. knoxi in this study.
This is the reverse of the pattern seen in the remnant stock from Huon Aquaculture
that was used in the treatment options studies (sections 5.2 and 5.3).
Clavier (1989), by the use of a condition index found no physiological effect of
mud worm infestation on abalone. However, the author was of the opinion that
extreme infestations (not considered in the random sampling method used) were
certainly detrimental to H. tuberculata. Kojima and Imajima (1982) found that 10
spionids per abalone reduced the flesh weight in a statistically significant way. In the
present study percentage flesh weight declined significantly with time (as blistering
increased) and for untreated infected stock compared to less infected air dried stock.
The severe declines in percentage flesh weight seen previously (to 53% in remnant
stock from original mortality reports) were not repeated in this study, with the
exception of some cohorts that were underfed rather than heavily infested.
Considerable variability exists in the literature regarding lethal and sublethal
effects of spionid mud worms in molluscs. Whitelegge (1890) investigated mass
mortality in Sydney rock oysters Saccostrea commercialis Iredale and Roughley
associated with P. ciliata, but later considered to be P. websteri (Blake and Evans,
FRDC Final Report Page 76
1972). Whitelegge recommended changes to culture techniques including lifting
oysters off the substrate and air exposure at low tide. These were highly effective and
are still used today. Smith (1982, 1984) reviews the collapse of the New South Wales
and Queensland oyster industries in the late 1880's and early 1900's and attributes a
great part of the blame to mortality caused by spionid mud worms. Korringa (1952)
reviews spionid infestation in oysters from the 1940's and early 1950's. In one case
involving high levels of infestation widespread mortality occurred but generally the
affects were more minor from a host health perspective.
Korringa (1952) states that when oysters contained over 25 P. ciliata or over 5
P. hoplura they showed poor growth and were often leaner than non infested oysters.
The P. hoplura species is among the largest of the spionids that infest shellfish and
usually considerably larger than B. knoxi. Therefore, it is true that larger worms cause
greater damage to the host, then infestation by 35 B. knoxi (as seen in remnant stock
from the original outbreak examined in 1997- section 1.3) should be less serious than
infestation by 150 P. hoplura (as seen in this study). Yet as this was not apparently
the case it may be suspected other factors are involved.
The work of Owen (1957) contrasts with reports attributing oyster mortality to
high levels of mud worm infestation. In this study 100% of the oysters studied were
infested and the spionid (P. websteri) numbers exceeded 100 per host at some study
sites. The author concluded that though in some areas a positive correlation was found
between oyster mortality and degree of infestation this was not confirmed in
controlled laboratory experiments. He concluded that P. websteri does not cause
oyster mortality per se but contributes towards the formation of a poor environment.
Sub-lethal impacts of spionids on bivalve molluscs have reported by several
authors. Kent (1979) found that heavy infestations of P. ciliata were associated with
reduced flesh content in mussels. Wargo and Ford (1993) found a significant negative
correlation between % blister coverage and condition index in the oyster species
Crassostrea virginica. When half of the shell was blistered the condition index of
oysters was reduced by up to one third. This study concluded that spionid infestation
reduced the ability of the host to accumulate nutritional reserves. Handley (1997)
found that B. knoxi produced a statistically significant negative impact on condition of
sub-tidally cultured oysters but that the effect was too weak to have any biological
significance. Cacerez-Marinez et al. (1999) found that heavy infestation of the black
clam Chione fluctifraga Showerby with Polydora sp. could break the shell leading to
In further studies Caceres-Martinez et al. (1998) found no correlation between
Polydora sp. numbers and blister area with condition of the oyster species C. gigas.
However, infestation levels were light with generally 1-3 worms recorded per shell.
Similarly, low level of infestation (generally < 5 worms per shell) in intertidally
cultured C. gigas was not considered to have any significant biological impact by
Handley and Bergquist (1997). Low level infestation by P. ciliata in the Indian oyster
Crassostrea madrasensis Preston was not reported to seriously injure the host
(Stephen, 1978). Heavy infestations of a mixture of spionids were reported in the
Japanese scallop Patinopecten yessoensis by Sato-Okoshi and Nomura (1990) but
health effects if any were not mentioned.
Contrasting conclusions in previous studies as to the health impacts of spionids
on molluscs may be due in large part to differences in the severity of infestation
(measured by spionid count or blister damage), the size of the host, host species and
other environmental factors such as food availability.
The long term treatment trial (objective 3, page 9) involving air drying in
December 1998 and 1999 was highly effective in reducing infestation by B. knoxi.
FRDC Final Report Page 77
Unfortunately P. hoplura rather than B. knoxi was the more numerous polychaete in
this study - reversing the pattern seen during the original mortality episodes. Some
post treatment infestation by P. hoplura meant that stock did not remain essentially
mud worm free between treatments. Even so, in the long term the treatment regime
resulted in lower infestation with P. hoplura, less blister damage and higher
percentage flesh weight in treated as opposed to untreated control stock. The treated
air dried stock also grew faster in the initial 6 months post treatment.
In summary, the majority of abalone intake time cohorts transferred to the 2
southern Tasmanian study sites failed to become significantly mud worm infested.
This was despite some abalone being exposed to up to 3 successive annual
spring/summer spionid larval dispersal periods. This is a positive finding for industry
indicating as it does that spionid infestation of stock may be a comparatively rare
event. Where abalone stocks did become heavily infested they appeared, in
retrospective, to have been at increased risk of infestation in terms of size and shell
fouling. None the less these stocks were able to sustain very high mud worm numbers
and associated shell blister coverage for up to two years without significant mortality.
Where stock mortality did occur abalone were exposed to poor husbandry conditions
in addition to high levels of shell blistering. Spionid infestation was shown to
potentially cause growth suppression and reduced flesh yield. A post larval settlement
treatment regime based on air drying reduced spionid numbers and associated
blistering. Monitoring of mud worm levels in future farmed stock from susceptible
areas would be prudent so as decisions can be made as to whether treatment is
5.6 Abalone health II: Physiology and histology
5.6.1 Clinical pathology data
Testing of apparently healthy abalone populations was conducted to ascertain a
normal range for farmed abalone and allow comparison with moderately and severely
mud worm infested stocks (Table 5.33). Only potassium (P<0.05, t=2.98, 18 df) and
the Na+/K+ ratio (P<0.001, t=-5.41, 22 df) were significantly different (by 2 tailed t
test) between spionid infested and control stocks for foot bleed data (Appendices 5R
and 5S). Statistical analysis of the smaller data group for the cephalic sinus bleed site
showed no significant differences between mud worm infested and normal abalone
(p>0.05 Mann-Whitney U Test) for any of the variables. As for foot bleed data there
was a rise in the Na+/K+ ratio in spionid infested compared to normal abalone for the
cephalic sinus data (Table 5.33), but this was not significant. Testing of water samples
from the abalone culture sites found a mean Na+/K+ ratio of 44.4 (SD=0.5, n=5).
Sufficient sample for full testing of electrolytes could not be consistently drawn
from the cephalic sinus in abalone under 50 mm in length. Sampling haemolymph
from the foot of abalone less than 20 mm length was also difficult. Samples taken
from 15 mm abalone (including composite samples from 2 or more animals) had a
Na/K ratio of 18.3 (SD=1.7, n=3), rising to 30.0 (SD=2.7, n=7) for 19 mm abalone
and 32.2 (SD=2.7, n=5) for 28 mm abalone.
Sodium/potassium ratio values changed significantly with time at both Huon
Aquaculture (df 8 69, P<0.001) and Aquatas (df 7 67, P<0.001). The initial control
sample for both sites (August 1998) had a value of 34.0 (SE=0.65, n=20) while the
final sample taken Oct 2000 at Huon Aquaculture had risen to 36.6 (SE=0.5,
n=10)(Figure 5.11). This was a lower rise than that of the equivalent cohort at
Aquatas where the final two Na+/K+ ratio samples in March and June 2000 exceeded
FRDC Final Report Page 78
40 (Figure 5.11). Aquatas stock of this cohort had begun to die in early 2000 (section
There was no significant difference by Kruskal-Wallis test (Hc = 6.154 <
χ2 0.05, 3 = 7.815) between Na+/K+ ratio data for monthly samples (Feb, Mar, Apr, May
2001) from experimentally starved abalone. The grand mean was 37.2 (SD=3.4,
Table 5.33 Haemolymph parameters from two bleed sites for normal and mud
worm infested abalone. Means with SD and n values in parenthesis.
normal abalone spionid infested abalone
235.1 (100.5, 10)
Glucose (mmol.l1)
* site location pairs for row means are significantly different (P<0.05)
FRDC Final Report Page 79
sample date
sample date
Figure 5.11 Temporal change in haemolymph sodium/potassium ratio for mud
worm infested abalone at two study sites (means ± SE)
FRDC Final Report Page 80
5.6.2 Haemocyte Data
A normal range for farmed abalone haemocyte counts was established by taking a
mean of 16 sample means from presumed healthy stock. These abalone between 30and 65 mm in length had a mean haemocyte count of 6.1×106 cells.ml-1 (SD=1.9×106
n=16). Individual sample means are shown in Figure 5.12, regression analysis found
no significant relationship between shell length and haemocyte count (df 1 14
Temporal data for the August 1998 intake groups showed significantly higher
haemocyte counts (P<0.05, U=11.0, Mann-Whitney U test) were maintained in mudworm infested abalone at Huon Aquaculture (5.41×106 ± 3.94×105, X ± SE, n=9) thanat Aquatas (3.41×106 ± 6.47×105, X ± SE, n=7) (Figure 5.13). There was only onesample from the Aquatas sample sequence with a count greater than 4×106 cells.ml-1
and therefore approaching the normal range (Figure 5.12). By contrast, all except onesample from the Huon Aquaculture stock had a count of approximately 4×106
cells.ml-1 or greater.
ocyte count (x10
shell length (mm)
Figure 5.12 Haemocyte count data for 16 populations of presumptive healthy
abalone (means ±SE, n=5 unless otherwise indicated).
FRDC Final Report Page 81
ocyte count (x10
ocyte count (x10
Figure 5.13 Temporal change in abalone haemocyte counts for August 1998
intake stock at two study sites (means±SE, n=5 unless otherwise indicated)
FRDC Final Report Page 82
Comparison of haemocyte counts in non-spionid infested, fast growing and
stunted abalone of the same age showed a trend for decreased haemocytes with
increasing degree of stunting (measured by size and relative to age)(Figures 5.14 and
5.15). This contrasts with data for presumptive normal abalone (Figure 5.12) where
haemocyte counts were independent of size (and age)
The "runts" of 2 year classes examined in 2001 had haemocyte counts
greater than 1 standard deviation below the normal range as previously established
above and comparable to spionid infested stock at Aquatas (Figure 5.13). Two way
ANOVA (size and age as factors) showed the biggest, fastest growing of the 1 year
old stock had significantly higher haemocyte counts (P<0.05) than the runts of the 2
year age class (Appendix 3, 5T for ANOVA table), although the latter abalone were
larger (Figure 5.14). Likewise, the runts of 2 year classes examined in 2000 had
significantly lower haemocyte counts (df 5 52, P<0.05) by ANOVA than other
abalone in their age group. Fast growing stock less than 1 year old had a higher mean
haemocyte count than larger, older 2 and 3 year old runts (Figure 5.15) but this was
not statistically significant (Appendix 3, 5U).
The value of haemocyte variation as an indicator of health was further explored
in relation to temperature stress. The first trial used 2 year old stock from farm 1 thathad been exposed to water at 22-23 °C for 4-8 weeks. These animals showed a
significant increase in haemocyte counts by ANOVA (df 2 27, P<0.05) at 3 sampletimes after transfer to water at 16 °C (Table 5.34). The mean count of 8.02 ×106
cells.ml-1 14 d post transfer was approximately 1 standard deviation above the
previously established normal mean. Count data from 2 abalone 14 d post transfer
were excluded from statistical analysis as they were much lower than other data and 1
of the animals was moribund. The mean count for these animals was1.4 ×105 cells.ml-1 (SD= 4.2 ×104), more than an order of magnitude lower than count
data for the other abalone (Table 5.34).
FRDC Final Report Page 83
ocyte count (x10
Length (mm)
Figure 5.14 Haemocyte data 2001. Two year classes, three size classes. Means ±
ocyte count (x10
Length (mm)
Figure 5.15 Haemocyte count data 2000 with samples from three age classes.
Means ± SE, n=10 unless otherwise specified.
FRDC Final Report Page 84
Table 5.34 Haemocyte counts following exposure to temperature reduction
from 21-22 °C to 16 °C, mean ± SD (cells. ml-1×106)
n=10, Means with shared superscripts are not significantly different (P>0.05)
Table 5.35 Haemocyte counts following exposure to elevated temperature,
mean ± SD (cells. ml-1×106)
control (14-15 °C)
Mean ±SD (n) 6.65 A ±3.1 (n=10)
8.11 A±5.50 (n=16)
7.27 A ±2.46 (n=9)
Means with shared superscripts are not significantly different (P>0.05)
Table 5.36 Variation in haemocyte counts with experimental starvation,
mean ± SD (cells. ml-1×106)
Mean ±SD 4.58AB ± 1.54
5.63 AB ± 1.29 7.61 A ± 1.96
Means with shared superscripts are not significantly different (P>0.05)
n = 10 initial count, n=5 remaining data
In a second temperature trial abalone that had been maintained at 14-15°C for 4
weeks were transferred to water at either 16 or 21°C. This resulted in cumulative
mortality after 6 d of 55 and 10% respectively. Haemocyte counts in the treatment
groups were elevated compared to the initial measure (Table 5.35), but not
significantly so (df 2 27, P>0.05, ANOVA). Three moribund abalone had a meanhaemocyte count of 4.89×106 cells.ml-1 (SD=1.11×106), lower than other groups in the
trial but with insufficient data for an appropriate statistical test.
Nine days exposure to 0.2 mg.l-1 copper sulphate resulted in an elevated meanhaemocyte count of 7.83×106 cells.ml-1 (SD=1.95×106, n=9) compared to the meancontrol count of 5.79×106 cells.ml-1 (SD=2.18×106, n=9). There was no mortality in
either group. The statistical significance of this small data set was borderline by t-test
(df 16, t=2.09, P=0.053) and Mann-Whitney U-test (U=19.5, P=0.06).
Kruskal-Wallis testing of haemocyte count data from abalone starved for threemonths found significant (Hc = 10.2991 > χ2 0.05, 3 = 7.815) variation between monthly
sample means (Table 5.36) as a result of the considerable decline in counts for the 3
month starved sample.
FRDC Final Report Page 85
Abalone infested in 1994-1996
All heavily mudworm infested stock showed elevated levels of brown staining
pigment granules in the right kidney tubules (Figure 5.16). Low levels of fine pigment
were sometimes present in non mudworm affected presumptive healthy control
animals. Severely spionid infested abalone also showed reduced right kidney
definition (Figure 5.16) and the lumen was often enlarged compared to controls
(Figure 5.17). Brown pigmented granules were typically located in the tubules of the
digestive gland or digestive diverticulum (Figure 5.18) and not seen in control
animals (Figure 5.19). Enlargement of the digestive tubule lumen and decrease in
interstitial tissue were also seen in severely mud worm infested abalone. Epithelial
cells of the stomach and intestine also showed the presence of brown pigment, more
so than normal controls.
There appeared no consistent changes in gill structure of mud worm infested
abalone but ciliates were not uncommon. Sections of foot tissue stained with PAS
showed reduced PAS positive glycogen tissue (Figure 5.20) contrasting with normal
abalone (Figure 5.21). Glycogen tissue was also depleted in the digestive tubules of
mud worm infested stock compared to healthy abalone. There was no evidence of
increased haemocyte activity or lesions in the shell muscle adjacent to blistered shell.
Nor was there obvious or consistent depletion of muscle mass seen by H&E or
specific Martius Scarlet Blue stain for muscle fibres.
Histology: August 1998 Intake
Animals in this time cohort were at least 3 years old in mid 1998 and were
considered relatively slowly growing. Histology of abalone collected May 1998
before transfer of animals to the sea showed no pigment in the right kidney. Further
histology on abalone collected August 1998 at transfer to the sea based farms showed
the presence of grade 1 right kidney pigment in 3/5 animals and grade 1 pigment in
the digestive tubules of one specimen. Two animals also had some pigment in the
epithelial cells of the gut. In abalone from Huon Aquaculture, moderate to heavy
(grades 2 and 3 respectively) quantities of brown pigment in the right kidney became
progressively more common in the 2 years following transferral to this site (Figure
5.22). Conversely the final sample taken October 2000 showed a return to the
minimal kidney pigmentation observed at the beginning of the time sequence. The
extreme levels of pigment seen previously in abalone surviving the first reported mud
worm outbreaks were not recorded. Reduction in right kidney definition and
enlargement of the lumen were rarely seen.
Brown pigment in the digestive tubules was absent during the first year of
sampling, with some scores of "1" and "2" recorded post October 1999.
Moderate reduction in digestive tubule height and interstitial tissue was associated
with light to moderate digestive tubule pigmentation in abalone sampled December
1999 and later. Pigmentation in the gut epithelium was widespread in all abalone
sampled June 2000. Final samples taken October 2000 showed the presence of gill
ciliates in 4 of 7 abalone. Gonad development was observed in samples taken summer
1999 and 2000. There was insufficient material to determine whether the heavy mud
worm infestation present by late 2000 suppressed sexual maturity.
FRDC Final Report Page 86
The equivalent August 1998 stock transfer to Aquatas developed grade "2"
pigment in the right kidney by September 1999 as well as some digestive tubule
pigment. By March 2000 when many of the animals had died, scores of 2 and 3 were
recorded in the right kidney of remaining specimens. The last sample taken in June
2000 was characterized by poor right kidney definition including the presence of
vacuoles and an increase in the lumen area of many tubules. Fine brown pigment was
widespread but only rated "1" in 4 abalone sectioned. There was a reduction of
interstitial tissue between digestive tubules in all samples, and a decrease in digestive
tubule height in 3 of 4 samples. Pigment in the digestive gland was rated 1-2. Other
than spionid infestation there was no evidence of significant pathology of abalone
tissue or shells.
FRDC Final Report Page 87
Figure 5.16 Right kidney in abalone with severe mud worm infestation.
P = large pigment aggregations, bar = 100 µm
Figure 5.17 Right kidney structure in control, non-mud worm
infested abalone, bar = 100 µm
FRDC Final Report Page 88
Figure 5.18 Digestive gland in severely mud worm infested abalone.
P = pigment deposits, bar = 100 µm
Figure 5.19 Normal digestive gland structure, bar = 100 µm
FRDC Final Report Page 89
Figure 5.20 Foot tissue, spionid infested abalone showing reduced
PAS +ve (red staining) glycogen tissue, bar = 100 µm PAS stain.
Figure 5.21 Foot tissue, normal abalone showing glycogen (red
staining) tissue, bar = 100 µm PAS stain.
FRDC Final Report Page 90
Figure 5.22 Temporal change in subjective right kidney pigment score for August
1998 intake abalone at Huon Aquaculture
5.6.4 Blister environment and microflora
All 10 blisters tested had pH in the range 7.5-8.0 as indicated by test strips (test
strip range 4.5-10.0). Water drawn from blister cavities and cultured on standard
media (Fish Health Unit, Launceston) showed no known pathogens and bacteria were
not considered present in large quantities by subjective assessment (Wagner 1999
pers. Comm.). Mixed Vibrio species were present and 2 of these were identified as
V. splendicus and V. aestuarianus.
Histological sections of severely mud worm infested shells from a variety of
sites showed no evidence of fungal hyphae in PAS and H&E stained sections.
Similarly, no evidence of fungi was observed in soft tissues adjacent to blisters or
elsewhere in infested abalone.
5.6.5 Tissue chemistry
Initial mean protein level in foot tissue was 2.98% (SE=0.53, n=5) wet weight
in September 1998, 1 month post transfer. In animals from Huon Aquaculture proteinlevels decreased significantly with time, (Hc = 20.731 > χ2 0.05, 5 = 9.488, Kruskal-
Wallis test) concurrent with increasing shell blistering and recovering to initial levels
in the final October 2000 sample (Table 5.37). In terms of shell blistering the October
2000 sample had the greatest blister coverage but also the highest proportion of
substantially nacre covered blisters (Figure 5.4). In abalone from Aquatas foot proteinlevels declined significantly (Hc = 13.45 > χ2 0.05, 3 = 7.815, Kruskal-Wallis test)
during the study period to a final value of 1.25% (SE=1.25, n=5) (Table 5.37).
FRDC Final Report Page 91
Table 5.37 Temporal variations in foot tissue protein content with mud worm
infestation at two study sites (means ± SE).
2.98AB ± 0.53, n=5
2.98A ± 0.53, n=5
1.81BCD ± 0.01, n=4
1.01 AB ± 0.03, n=5
1.68BC ± 0.14, n=7
0.79 B ± 0.08, n=5
1.59C ± 0.29, n=4
1.25AB ± 0.21, n=5
2.58AD ± 0.20 n=13
Column means with shared superscripts are not significantly different (P>0.05)
5.6.6 Respiration rate
Mean oxygen consumption was 1.50 ± 0.19 µmol.g-1.h-1 ( X ± SE, n=8) inchronically mud worm infested stock at 20 °C, significantly higher (U=3.0, P=0.01
Mann-Whitney U Test), than that of non mud worm infested control abalone0.81 ± 0.15 µmol.g-1.h-1 ( X ± SE, n=4). Dissection of animals revealed thatpercentage flesh weight of mud worm infested stock was 60.3% ± 2.3% compared to82.3% ± 4.4% for non -infested stock ( X ± SE, n=8 and 4 respectively) a statistically
significant difference (U=0, P<0.01 Mann-Whitney U Test).
In a second experiment at 16 °C mean oxygen consumption was 0.96 ±0.05µmol.g-1.h-1 ( X ± SE, n=5) in heavily spionid infested abalone, significantly higher
(U=0.0, P=0.01 Mann-Whitney U Test) than that of lesser (methods: section 4.16.6)mud worm affected animals 0.68 ±0.04 µmol.g-1.h-1 ( X ± SE, n=5).
5.6.7 Ammonia excretion
Ammonia excretion in heavily mud worm blistered abalone was0.29 ± 0.08 µmol.g-1.h-1 ( X ± SE=, n=10), not significantly higher (U=44, P>0.05,Mann-Whitney U Test) than that of lesser affected animals 0.21 ± 0.04 µmol.g-1.h-1( X ± SE, n=10). The less blistered abalone were intended to be a non-spionid infested
control but post experiment dissection revealed blister coverage of 10.9% compared
to 28.6% for the highly infested animals.
5.6.8 Starvation Trial
Sixteen of 40 abalone died in the week following transport and the
commencement of the trial period. Clearly these deaths could not be assigned to
starvation but appeared to be due to transport stress. A further abalone died 8 weeks
following the commencement of the trial. Following 12 weeks starvation abalone had
recorded a mean weight loss of 11.9% (SD=6.5%, n=6). Histological and clinical
pathology findings are discussed in the appropriate sections above.
FRDC Final Report Page 92
As for the preceding health section the data above relate to objective 2 on
investigation of interactions between spionids and abalone. The haemolymph of
marine molluscs, which corresponds to the blood and interstitial fluid of higher
animals, is very similar in ionic composition to the surrounding seawater (Burton,
1983). Haemolymph solute concentrations were similar between this study and that of
greenlip abalone (Harris, 1999) for potassium, sodium and chloride. Calcium and
magnesium levels are somewhat higher in this study than that of Harris (1999) and
both sodium and chloride levels are about 50 mmol.l-1 higher than those reported from
greenlip abalone by Boarder (1997). However this is likely to reflect slight differences
in salinity of the surrounding sea-water. These studies in total comprise a useful data
base of blood parameter regulation that may be useful in determining health status of
abalone generally.
While there is little if any regulation of sodium, chloride and magnesium in
marine molluscs, potassium concentration in haemolymph may exceed that of
seawater by a factor of 1.1-1.5 (Burton, 1983). Potassium levels in normal abalone
from this study averaged 13.5 and 11.6 mmol-1 for foot and cephalic sinus sample
sites respectively (Table 5.33, ionic composition). These are elevated compared to
mean potassium concentration of 9.7 mmol-1 from southern marine farms
(Appendix 3, 5V) and thus consistent with Burton (1983). Interestingly, while
potassium levels in healthy abalone were regulated at higher than seawater
concentrations these levels fell, significantly so, for samples drawn from the foot of
mud worm infested animals (Table 5.33). As noted above, potassium haemolymph
levels were greater in the foot than cephalic sinus of abalone as was the magnitude of
the decrease in moderately to severely mud worm infested animals. In part this may
be a consequence of potassium leakage from cells damaged as a result of the foot
sample method. Russell and Evans (1989) report that several authors describe means
by which the foot can be isolated from the rest of the abalone cardiovascular system.
If this is true it may be that mud worm stressed abalone attempt to maintain
osmoregulation in the visceral mass at the expense of the foot, leading to a greater
drop in haemolymph potassium from this site relative to the cephalic sinus. Whatever
the cause of this variation between sampling site, haemolymph potassium levels are
regulated in abalone, with less effective regulation in mud worm infested animals. As
the magnitude of potassium variation was greatest in samples from the foot this site
may provide a more sensitive measure of stress than the cephalic sinus. Potentially,
testing of haemolymph potassium levels may have application as a general stress test
in abalone, provided this is coupled with external salinity and / or haemolymph Na /
Cl levels to establish relative unregulated levels.
Testing of haemolymph copper found no significant differences between
moderately to severely mud worm infested abalone and normal animals from either
bleed site. The foot bleed data set for worm infested stock was highly variable (Table
5.33). Such variation was noted in experimental control abalone by Harris (1999) and
in field studies by Ainslie (1980). Therefore assessment of respiratory pigment level
by indirect copper measurement appears of little value as a health indicator. Possibly,
direct measurement of the abalone respiratory pigment haemocyanin is worth
Haemolymph glucose levels in mud worm infested abalone were approximately
half that in normal abalone (Table 5.33). However, this difference was not statistically
significant due to the small size of these data sets (Appendix 3,5S). A reduction in
FRDC Final Report Page 93
glucose levels in spionid infested stock would not be unexpected given the respiration
data (section 5.6.6) indicated an increase in metabolic rate for these abalone. Cheng
and Lee (1971) and Livingstone and De Zwaan (1983) reported significantly lower
glucose levels in the haemolymph of parasitized molluscs. The mean level of 0.2
mmol-1 glucose in the present study mud worm affected stock was very similar to the
levels reported for reduced salinity stressed abalone by Boarder (1997). Normal
levels of haemolymph glucose were higher in the study by Boarder (1997) compared
to the present study (0.75 compared to 0.40). Sample collection trips were conducted
over 2-3 days in the present study followed by relocation to the laboratory before
processing. Abalone were not fed in this time which would be sufficient, based on
data presented by Carefoot et al., (1993), for glucose levels to drop markedly. For this
reason haemolymph glucose sampling was not considered a high priority in this study.
It may, however, be worthy of further investigation as an indicator of abalone health,
especially where stock are still feeding.
There were no significant differences between haemolymph protein levels in mud
worm infested and normal stock. This is consistent with the copper data as most
protein in the haemolymph would be expected to be associated with the copper in the
haemocyanin respiratory pigment. Lee and Cheng (1972) as cited by Malek and
Cheng (1974) found that haemolymph protein reduction in the planorbid snail
Biomphalaria glabrata as a result of parasitism with Schistosoma mansoni was
largely due to reduction in the respiratory pigment (haemoglobin) concentration.
A normal haemocyte range was established for cultured blacklip abalone that
was found to be well above that for long term mudworm infested abalone in poor
health at Aquatas. Malek and Cheng (1974) note that there is no total agreement on
types of haemolymph cells that occur in molluscs – except that all species possess
leukocytes. Various classification systems are discussed by these authors and by
Simkiss and Mason (1983) and Sahaphong et al. (2001). Bevelander (1988) states that
only one leukocyte type is found in the haemolymph of abalone with other cell types
present in connective tissue, although this does not appear true of all abalone species
(Sahaphong et al, 2001). Haemocyte counts have previously been found useful in
abalone disease investigations by Shields et al. (1996). These authors found
significant differences in subclasses of leukocytes between healthy H. cracherodii and
those with wasting or withering syndrome. Friedman et al. (2000) in a study of the
same disease in the same host abalone species found that the haemocytes of diseased
abalone were compromised in their immune functions and these may serve as early
indicators of the disease. Friedman et al. (1997) found the New Zealand abalone H.
australis had a mean circulating haemocyte count of 6.72 × 106 which is within thenormal range established here for H. rubra and close to the mean of 6.1 × 106.
Furthermore, these authors found that haemocytes were significantly elevated in H.
australis affected by fungal lesions of the shell (to 1.19 × 107). By contrast, there was
no difference between fungal infected and control abalone of a further species, H. iris.
Changes in gastropod haemocyte numbers as a result of parasitism have also been
reported by Stumpf and Gilbertson (1980, as cited by Bayne 1983) and as a result of
temperature variation (Pauley and Krassner 1971).
Haemocyte count variation was also seen in the present study in relation to
temperature stress and also to sub lethal toxin exposure (copper). These changes
occurred relatively rapidly (1-2 weeks) and therefore have potential as a general stress
indicator. The three-month starvation trial resulted in an initial rise in haemocyte
count followed by a significant decline in the last sample. By contrast there were no
significant changes in Na+/K+ ratio during this time.
FRDC Final Report Page 94
Histological examination was performed on abalone surviving from the initial
mud worm outbreaks in the mid 1990's and on a sequence of increasingly infested
stock placed at the study sites in 1998. Major findings involved changes to the right
kidney and digestive tubules, including substantial deposition of brown pigment
granules. Reduction in right kidney definition and enlarged lumen associated here
with moderate and severe mud worm infestation has been reported by Harris et al.
(1998) in relation to ammonia exposure. Brown pigment granules were described in
the cytoplasm of normal abalone right kidney cells (Bevelander, 1988) and the
elevated quantities associated with moderate and severe mud worm infestation have
been previously reported by Harris et al (1998) in relation to elevated nitrite exposure.
These authors suggested increased pigment might be a reflection of increased kidney
protein and hence cell turnover. Malek and Cheng (1974) note that yellow/brown
"excretory or ferment globules" are a feature of parasitized molluscan digestive
glands and especially so when the host parasite interaction is of long duration. These
authors state that the globules represent accumulated metabolic wastes in the digestive
gland. The pigment granules in the two tissue types therefore indicate a long term
stress consistent with length and severity of mud worm infestation in the present
Mud worm infestation apparently leads to depletion of host energy reserves.
During starvation of abalone glycogen, triglyceride and protein reserves are utilized
consecutively (Takami et al., 1995). In withering foot syndrome of H. cracherodii
(caused by a rickettsia), where the digestive diverticula or tubules are directly
damaged by infection and carbohydrate storage is reduced in non-infected tubules of
infected animals (Gardner et al., 1995), the resulting prolonged starvation leads to
foot muscle atrophy including severe depletion of muscle fibres. PAS staining of
severely mud worm infested abalone showed reduced carbohydrate stores in the
digestive gland and routinely stained sections showed increased tubule lumen size,
also indicative of poor nutrition. However while the foot of severely mud worm
infested abalone was sometimes completing lacking in glycogen tissue, depletion of
muscle fibres was not seen, confirming that the degree of foot atrophy was less than
with severe withering foot syndrome. Histological examination of severely spionid
infested abalone was negative for the characteristic rickettsia seen in abalone with
withering foot syndrome.
Normal levels of soluble protein in the foot were similar to data given by
Takami et al. (1995) for H. discus and the reduction in values to less than about 1.5%
wet weight in mud worm infested stock was similar to levels in 30 d starved abalone
(Takami et al., 1995). Extreme low levels reached in some infested stock from
Aquatas (Table 5.37) were like those of 70 d starved stock reported by Takami et al.
(1995). Interestingly, foot protein levels in mud worm infested stock at Huon
Aquaculture recovered from low values to close to the normal level in the final
October 2000 sample (Table 5.37). This foot protein recovery coincided with an
absence of moderate pigment deposits in the right kidney. These latter, had been
present in most samples taken in the previous year and the finding supports the notion
that the right kidney pigment was the result of catabolism of foot protein.
This is interesting in relation to temporal shell blistering for the cohort, in that,
blisters present were progressively better healed with the maximum value (80%
healed – derived from Figure 5.4, Section 5) occurring in the October 2000 sample.
By contrast, in the comparable Aquatas cohort, blisters assigned to the "healed"
category constituted less than half of blister area in the last two samples (Figure 5.8,
Section 5). Thus the assignment as "active" blisters on the basis of their conspicuous
conchiolin protein colour was consistent with continued low foot protein values.
FRDC Final Report Page 95
Respiration rates in this study are in line with previous studies on abalone by Uki
and Kikuchi (1975), Barkai and Griffiths (1987), Nimura and Yamakawa (1989),
Segawa (1991), Carefoot et al., (1993), Paul and Paul (1998) and Harris (1999).
Studies on heart rate and respiration of the gastropod B. glabrata parasitized by the
larval trematode S. mansoni have generally showed an increase in infected stock
relative to controls (Malek and Cheng, 1974). This was consistent with the two
respiration trials in the present study but contrasted with oxygen consumption data for
abalone with withering foot disease where consumption decreased (Kismohandaka et
al., 1993) or remained unchanged (Kismohandaka et al., 1995) relative to unaffected
animals. Possibly these differences reflect interaction between the disease and extent
of host starvation. It has been shown by Segawa (1991) and Gaty and Wilson (1986)
that oxygen consumption in abalone decreased in starved animals.
There was no significant difference between ammonia excretion for greater and
lesser mud worm infected stock. Excretion rates were similar to those of abalone of
comparable size reported by Segawa (1991) for H. diversicolor but higher than
excretion rates for H. midae (Barkai and Griffiths, 1987). There is little literature
relating ammonia excretion in abalone or other molluscs to disease. Kismohandaka et
al. (1993) found that abalone with withering syndrome excreted 3.8 times more
ammonia than did healthy animals. The contrast between this study and the mud
worm infestation data may be due to the lack of a suitable mud worm free control in
the latter case. As for oxygen consumption data the extent of starvation associated
with the disease condition might also influence the outcome. Segawa (1991) found
that ammonia excretion at first increased in starved abalone then decreased after 17 d.
The changes that occurred in abalone that contracted mud worm infestation as
the result of experimental placement in 1998 were not as great as those that occurred
when abalone were first farmed in susceptible areas. Stock first infected in 1994-96
and examined 1996-1999 had greater subjective shell damage ratings, decreased
percentage flesh weight, increased B. knoxi counts and more extreme histological
change compared to abalone placed subsequently to replicate the pattern for study
purposes. These changes manifested themselves in high mortality rates at a number of
farms in the mid to late 1990's. Possible reasons for different levels of infestation and
spionid impacts between past and present are examined in the general discussion.
In summary, moderate to severe mud worm infestation lead to depletion of
abalone energy reserves. This was indicated by histological changes to the right
kidney and digestive tubules consistent with increased cell turnover; with depletion of
glycogen and protein tissue reserves; reduction in haemolymph glucose and with
increased oxygen consumption. Such changes were likely the result of mobilization
and direction of host resources to shell repair, that may have manifested itself as
reduced growth, and in some instances death. Spionid infestation was also associated
with reduced capacity to regulate potassium and in severely impacted abalone with
significantly lowered circulating haemocyte counts. Similar low levels of haemocyte
counts were seen in "runted" stocks and 3 month experimentally starved animals.
Haemocyte counts are considered potentially useful as a general indicator of health
FRDC Final Report Page 96
5.7 General discussion
Background and Need
High levels of mortality of sea-farmed abalone in southern Tasmania during
the mid 1990's, which led to this research, was linked to spionid mud worm
infestation after extensive laboratory examination showed this to be the only
consistent finding (Handlinger, unpublished). These mortalities progressed slowly
over many months in most groups on several farms in the sea based farms in southern
Tasmania and involved the mortality of > 30 000 animals, comprising a very high
percentage of total stock. It should be noted, however, that mud worm problems were
not and are not confined to southern Tasmanian off shore sites. Severe infestations,
including mortality, have also been found in abalone reared in land based systems in
Tasmania, South Australia and Chile, and in sea based systems in West Australia and
New Zealand (reviewed in Sections 1 and 2). There was considerable data on spionid
infestation of mollusc populations, including abalone, but until the development of
abalone farming, the perception of negative impacts was largely restricted to oysters,
for which shell treatment by air drying or other osmotic insults had long been
developed and were readily used with minimal impact on oyster health. As univalves,
abalone are unable to avoid these osmotic effects through shell closure, and a more
comprehensive approach to spionid control was needed. The spionid species B. knoxi,
previously unknown in Australia, appeared to be the major cause of the losses, being
present on all affected farms and numerically dominant at the farm with the greatest
stock losses. As this species was not previously recorded in Tasmania (Wilson et al.,
1993) it was speculated that it may have been a recent and relatively harmful spionid
introduction from New Zealand. The research was therefore intended to focus on the
spionid species B. knoxi, though unexpectedly, P. hoplura was the most numerous
species present in abalone during this field research and was therefore also the focus
of study.A multi-pronged approach was devised with the aim of producing an
integrated package of measures to minimize the impact of spionids on abalone culture
in these susceptible locations. The first control strategy investigated was chemical and
physical treatments. Once treatment options were found to be limited and stressful to
stock, broader studies were undertaken to allow parasite management through
understanding and manipulating the host-parasite interaction. The final
recommendations involve a mixture of parasite avoidance through minimising
opportunities for spionid settlement on stock, particularly timed placement of stock,
and strategic treatment.
Developing a treatment of mud worm infected abalone
The testing of chemical treatments was undertaken as part of the preliminary
CRC funded project. Sixteen potential chemotherapeutic agents and fresh water
immersion were tested, using available literature on effective concentrations for bath
treatment of fish and shellfish parasites as a starting point. It was concluded that the
anti-parasitic compounds trichlorofon, praziquantel, febantel, pyrantel embonate,
FRDC Final Report Page 97
metronidazole, dimetronidazole, and the surface treatment agents hydrogen peroxide
and methylene blue were not effective against spionid polychaetes at doses which
were high relative to dosage quoted in available literature. Immersion in freshwater,
formalin, the topical treatment dyes potassium permanganate, gentian violet,
malachite green, and the levamisole, ivermectin, and the benzimidazoles
mebendazole and fenbendazole was highly effective against mud worms in vitro.
However, the protection conferred by the burrows of spionids in situ rendered
effective concentrations unsafe for abalone. The most promise in vitro treatment was
shown by the benzimidazole class of drugs. These may have some potential for
improvement as a treatment for various polychaetes by using microencapsulation of
the drug as burrow protection in these trials is likely to have been compounded by
poor solubility in water. Further work in this direction was abandoned in favour of the
promising results from preliminary air drying experiments.
Treatment by air drying
Treatment by air drying not only showed the most promising results from the
preliminary treatment experiments, but had the advantages of practicality, safety to
operators, and lack of residue concerns. Like most traditional mollusc treatments for
spionids, it utilises osmotic challenge with relative host protection provided on the
basis of size, rather than metabolic differences which the above results indicate are
generally slight between these two marine invertebrate groups. While abalone have
poor tolerance to osmotic changes when emersed, they are moderately well protected
by mucus against short-term air exposure, which is a natural experience of abalone in
some parts of their range. Air drying of stock has a history of use in oyster culture
(Smith 1984, Nell and Smith 1988) and is the basis of the success of intertidal oyster
culture which sacrifices feeding opportunities (when immersed) for the treatment
benefits when exposed.
Treatment duration - time for effective treatment: All spionids were shown to
be highly susceptible to drying in vitro, but to be protected to a greater or lesser extent
from effective drying in vivo by their location within the shell. The body of
experimental work demonstrates that treatment of small B. knoxi (and small P.
hoplura) can be highly effective, with reduction in numbers for all classes of worm in
almost all experiments using treatment times of 4 hours. Larger mud worms (> 5 mm
and typically 10-25 mm) may be susceptible to drying treatment depending on a host
of factors. These appeared to include: infestation time, blister severity, stock size and
stock fouling. Increasing infection period allowed mud worms time to become
established in burrows deep within the shell. Where larger chambers occurred within
blisters survival appeared especially likely.
In relation to this, observed differences in blister morphology between mud
worm infested abalone and bivalve molluscs in Tasmania are of interest. In bivalves
B. knoxi is observed to inhabit very large water filled blisters whereas in abalone
blisters rarely have a significant volume. Traditionally treatment for mud worms in
Sydney rock oysters utilizes much longer drying times, such the 10-14 day emersion
time noted by Nell and Smith (1988), which appears to be based on the work of
Whitelegge (1890). While this may be excessive for shells relatively clear of mud,
there has been little effort to define a minimum effective time as Sydney rock oysters
have a high tolerance to both air exposure, and to freshwater as an alternate treatment.
Recent trials on treatment of large Pacific oysters heavily infested with B. knoxi
suggested freshwater treatment of at least 24 hours duration was required to kill the
FRDC Final Report Page 98
majority of the mud worms protected by large blisters (Lleonart and Handlinger,
unpublished). Such treatment is certainly beyond the physiological limits of abalone.
In view of the lower resistance of abalone to drying it is fortuitously that blisters
typical of B. knoxi and to a lesser extent P. hoplura in abalone may provide relatively
poor protection against desiccation strategies.
Treatment duration - safe levels: Abalone of 15-20 mm or greater proved
surprisingly tolerant of air drying. Spionid treatment data (section 5.3) showed that
abalone consistently survived 2-4 h air drying, sufficient to significantly reduce mud
worm levels. There was no mortality of stock of this size dried for up to 3 h and of 40
mm animals dried for 11 h water under conditions appropriate for elimination of
spionids. This proves ample scope for effective treatment. This contrasts with poorsurvival reported for smaller abalone (5-10 mm) air dried in shade at 24°C (Whang
and Chung 1977). These longer exposure data relate mainly to blacklip abalone. There
may be differential drying tolerances between haliotid species requiring investigation
in the future. However while it was shown that several hours of air-drying rarely
resulted in immediate post-treatment mortality, there was the potential for reduced
growth over the following months in at least some groups. As heavy spionid burdens
also reduce growth (at levels below overt mortality), current recommendations would
be to avoid longer air drying by treating while shorter exposure times (2-4 hours) are
likely to be effective, and to practice avoidance strategies to minimize the number of
Temperature and humidity: Treatment for this duration (2-4 hours) was more
effective at 21 °C (up to 90% reduction under trial conditions), than at temperatures of15 – 18 °C (approximately 70% reduction). Treatment at 24 °C failed due to higher
associated humidity which resulted in incomplete drying of shells. However other
factors (such as duration and severity of infection, and the degree of treatment
induced stress) had a greater influence on the optimum economic time for treatment.
Avoiding extremes and minimising the differential between air and sea temperature
were considered more important than timing treatments to achieve an optimum
Methods for mud worm control - Recommended protocol for air-drying
The following protocol summarises the more detailed treatment protocol given in the
attached Farm Manual (Appendix 4). Short term treatment air drying may potentially,
but not necessarily, reduce the growth rate of stock. To minimize stock stress it is
suggested that the differential between air and sea temperature be minimized,temperatures > 24 °C or < 15 °C be avoided, and stock be starved for several days
before treatment in line with the findings of Watanabe et al. (1994). Air humidity
should be less than 64%, to ensure that the shell of the abalone dries sufficiently. If
shells of stock are not apparently drying within 30 minutes of air exposure the
conditions are probably unsuitable. Typically, days with minimal cloud cover will be
sufficiently dry for treatment in southern Australia. Abalone should be dried in shade,
not direct sunlight.
FRDC Final Report Page 99
Methods for control of mud worm – avoidance strategy
SETTING UP THE FARM
Assessing overall level of mud worm risk
Assessment of the risk of significant mud worm infection is an important
component of site selection and design, but must be balanced against other
considerations. The economically significance will depends both on the value of lost
production and the cost of treatment or risk amelioration.
To define factors affecting this risk, the spionid life cycles and host-parasite
interaction was studied by replicating as far as possible the original culture situation
between mid 1998 and early 2001, using in excess of 9000 abalone placed at two
locations with a previous history of severe spionid infestations. Interestingly only
200-300 of this stock became infested to the levels previously associated with abalone
mortality. This indicates that the risk of severe mud worm problems in these locations
are not as great as previously feared by growers on the basis of events in 1995-1996.
The mortality rate in this study was low and confined to mud worm infested
underfed stock at one site. This contrasts markedly with events in the mid 1990's
where considerable mortality occurred at several locations. Explanations for the
difference in mortality rates between past events and the present study are of
considerable interest to growers. There appear to be two major possibilities: that
either, during the past mortality episodes there were other important stress factors
operating in addition to mud worm infestation, or that infestation levels were higher
and more severe in the past due to a different infestation pattern at that time. Factors
affecting the level of infection and the of severity of consequences are discussed
below, as both are relevant to management decisions regarding utilisation and
management of mud worm susceptible sites.
Factors affecting the level of infection.
Spionid species: In trying to reconstruct factors that may have lead to the mass
mortality of the mid 1990's the role of spionid species must be examined. Although B.
knoxi was implicated as the most significant spionid species in the original
outbreaks, P. hoplura was unexpectedly found to be the most numerous species
present in abalone during this field research, outnumbering B. knoxi by 5-10 to 1 in
heavily infested stock. B. knoxi was the dominant species present at the Huon
Aquaculture Company during the original infestations, contrasting with its
representation at both study sites during field trials. It should be recalled, however,
that infested abalone at sea based operations other than Huon Aquaculture showed the
presence of both B. knoxi and P. hoplura. Furthermore in the early days of land based
abalone farming one such farm in each of Tasmania and South Australia suffered
stock mortality associated with purely P. hoplura infestation. Since that time verbal
reports of abalone mortality associated with spionids other than B. knoxi have
originated from Australia and overseas. Thus it is difficult to make a convincing case
that B. knoxi is an unusually destructive spionid species. However different life-cycle
strategies may affect the level of infection.
Spionid life cycle and larval dispersal pattern: In the present research, the
reproduction of the two major spionids, B. knoxi and P. hoplura, was investigated
FRDC Final Report Page100
with an emphasis on the possibility of avoiding larval settlement. B. knoxi was found
to have exclusively planktotrophic larval development confined to the Austral spring,
consistent with the findings of Handley (2000) for B. knoxi in New Zealand. The
reproductive strategy behind planktotrophy is one of high larval production (estimated
at 700 larvae/brood – section 5.1) to account for a high attrition rate. There is also
considerable scope for dispersion, due to the length of time in the plankton. This
"boom or bust" capacity for larval production may account for both the original
mortality episodes as described above and the annual variation and relative scarcity of
the species 1998-2001. Thus B. knoxi (and other planktotrophic spionids) may have
the potential to cause significant harm to mollusc culture enterprises when
environmental conditions favour high larval survival. The consistency of B. knoxi
seasonal reproduction fortuitously allows a simple avoidance strategy to be practiced
in susceptible areas. Clearly, transfer of stock to susceptible sites post November
allows approximately 10 months growth before potential infestation in the following
Larval settlement data for P. hoplura (section 5.1) suggested that initial
colonization with this species could also substantially be avoided by placement of
stock post spring and preferably after mid summer. Once established in abalone,
however, the species showed the potential for year round lecithotrophic reproduction
(crawling not swimming larvae, leading to a local spread). Thus numbers of this
species have the potential to rise steadily over time and this needs to be taken into
account and monitored by growers – especially if the grow out period extends over 2-
3 years in the sea.
Variations in annual spionid settlement rates: Anecdotal evidence from Tasmanian
oyster farmers that "there are good and bad mud worm years" is supported by Pacific
oyster blister data collected by the Fish Health Laboratory (Appendix 3, 1C). This
data gives the percentage of blister positive oysters in farmed populations but not the
extent of blistering or the spionid species responsible. None the less it is interesting
that on the basis of this data 1995 recorded the highest blistering levels. Available
abalone mortality data shows death rates of 12% and 32% between December 1995
and April 1996 for animals stocked January and August 1995 respectively. Stock
placed June 1994 suffered little mortality until December 1995, with an overall death
rate by April 1996 of 31% (Appendix 3,1A- Figure 1). These farm mortality data are
consistent with the growth trial studies of Hindrum (1996) which reported 40%
abalone mortality between November 1995 and April 1996. These records support the
possibility of heavy B. knoxi settlement at this site in the spring of 1995, and the
likelihood of future annual variations of risk. Thus data over several years may be
required to fully assess the mud worm risk of a site.
Most of the abalone for which temporal mortality data are available were
placed at 20-30 mm. This contrasts with the present study, where stock placed at
between 20-40 mm were minimally infected, in line with experiments on stock size
and mud worm settlement (section 5.4.1). In combination these also suggests
relatively high numbers of larvae in the water spring 1995. The rainfall data for Dover
(Appendix 3, 5W) near Huon Aquaculture are of interest in relation to factors that
might contribute to spionid infestation. July and August 1995, just before the
presumptive B. knoxi larval dispersion phase of 1995 received approximately twice
the 100 year average for these months. Calculation showed that rainfall for July and
August of that year, plus the spring months when the B. knoxi is in the water column
was the third highest in 100 years (Appendix 3, 5W). Runoff from heavy rain could
FRDC Final Report Page101
benefit spionids by putting more nutrients in rivers and the sea leading to enhanced
production of spionid larval food sources. This is consistent with references to
increased spionid populations near drains and other sources of organic pollution
(Woodwick 1964, Anger 1977). This has predictive value, identifying wet springs as
years requiring vigilance, and should also be taken into consideration is assessing
mud worm risk data from a limited number of seasons.
The rate of mud worm recruitment rather than the numbers per se may be
important determinants of host impact, with a likely interaction with host size.
Abalone from Huon Aquaculture, placed 1994-1995 and surviving until 1997 had up
to 40-50 B. knoxi chimneys (section 4.5). It is plausible therefore that stock deceased
before this time had even higher levels of infestation. Total spionid numbers in the
August 1998 Huon Aquaculture experimental intake abalone eventually reached
levels seen in the 1997 survivors but this took approximately 2 years (section 5.5). If,
by contrast, in spring 1995 the Huon Aquaculture Company abalone experienced
settlement of, for example, >30 B. knoxi the effects might have been sufficiently
devastating to explain the onset of mortality in December 1995 - April 1996.
The effect of shell fouling on spionid settlement: In addition to avoidance
strategies based on spionid reproduction and settlement, the research identified other
factors of importance in minimizing spionid impacts. Tube building polychaetes,
especially Spirorbis sp. when present on abalone were found to enhance settlement of
B. knoxi. Culture facilities in susceptible areas would thus be advised to source spat
without significant quantities of these fouling organisms. Fortunately, in southern
Tasmania the settlement season for spirorbids is similar to that of spionids (section
5.4.2). Therefore stocking regimes designed to avoid mud worm infestation in the first
year will result in minimal spirorbid fouling and thus relief from the B. knoxi
enhancement effect for a substantial time. Control of spirorbids in land based farms
may be an area requiring further research, and the level of spiorbids another factor in
assessing site risks.
Effect of abalone size on settlement rates: When stock of several size classes were
exposed to spionids at the same time, larger stock became significantly more infected.
By contrast, as small minimally affected abalone grew from approximately 20 to 60
mm over 2 years spionid infestation remained at very low levels.
Effect of height in water column on settlement rates: Abalone reared closer to the
bottom were found to recruit higher numbers of P. hoplura. Thus it may be prudent to
raise culture vessels from the bottom as has been recommended previously for oyster
culture (section 5.4.4).
Factors affecting severity of consequences.
Additional stress – underfeeding: As noted previously, there was mortality of the
underfed spionid infested stock relative to the better managed cohorts in the study.
This suggests the potential for adverse conditions to magnify the impact of spionid
infestation. However, at least one grower has indicated that stocks were properly
maintained with regard to feeding before and during the original mud worm
outbreaks. Further, Hindrum (1996) reported growth rates of 80-100 µm.day-1 (not
typical of underfeeding) in sea farmed abalone that later experienced heavy mortality
FRDC Final Report Page102
Additional stress – water quality factors: Retrospective shell grading of abalone
submitted from Huon Aquaculture in 1997 and used for treatment trial experiments
(sections 5.2 & 5.3) showed that 16% had slight or no mud worm infestation (SSDR
grades 0-1, Appendix 3,1B). Shells examined from this source in 1997 sometimes
included high proportions of shells from dead abalone and at least some of these
shells had little or no mud worm infestation. It is possible, therefore, that the most
severely affected abalone, (grade 3 rated shells account for 36% of the 1997 sample-
Appendix 3,1B), died largely as a result of their spionid infestation. Subsequently,
the resulting poor water quality, in combination with some degree of mud worm
infestation may have caused the deaths of further stock.
Available farm mortality data (Appendix 3, 1A) for 1994-96 shows a high
death rate in the Austral summer for 1995/1996. Another factor of potential
importance in these deaths was the widespread use in the mid 1990's of "tube" type
rearing vessels. These had relatively restricted water flow, especially once fouled with
seaweed after some time in the water (sections 4.3 & 5.4.3). Thus the combination of
mud worm infestation, summer water temperature and the presence of dead stock in a
small, relatively sheltered containment vessel could potentially magnify the impact of
severe mud worm infestation.
Physiological consequences of infestation: As discussed above, where experimentally
exposed abalone became heavily spionid infested, mortality could be attributed to the
combination of severe shell blistering and underfeeding but not to significant
infestation as a sole known stress factor. Spionid infestation led to reduced growth
and a decrease in percentage flesh weight. These gross changes were manifestations
of disruption to normal physiology and biochemistry, including increase in oxygen
consumption and utilization and reduction of tissue glycogen and protein stores.
These latter were reflected in histological changes to the right kidney and digestive
gland. Thus the health of abalone was compromised, possibly because resources were
diverted from normal functions to repair of the shell. Severely spionid compromised
abalone also showed a reduced capacity to regulate potassium and had lower
circulating haemocyte counts than normal animals. These changes, especially the
latter may have potential as general indicators of chronic stress in abalone. Similar
low levels of haemocyte counts were seen in "runted" stocks and 3 month
experimentally starved animals. For effective interpretation of such changes, however,
knowledge is also required of the responses and variability of haemocyte responses to
short term stresses. For this, repeat experiments need to be performed using larger
numbers of abalone and a variety of challenges such as other infectious agents and
adverse water conditions such as sub optimal ammonia, nitrite, pH and dissolved
oxygen levels. If the response patterns are sufficiently predictable, long term
monitoring of haemocyte counts in stocks before and during summer could be a
useful addition to health studies on so called "summer stress syndrome". This has
emerged as a serious problem for land based abalone farms in parts of Australia and is
linked to elevated water temperature and bacterial infection, but there are reports that
mud worm infestation may also sometimes be a factor.
Thus there is ample evidence, despite the low levels of mortality during these
experiments, that spionid mud worm shell infestation does significantly compromise
production and health. As treatment may also compromise health, the general
principles of mud worm control should be aimed at optimizing growing conditions,
minimizing spionid settlement through timing of stock deployment and selection of
FRDC Final Report Page103
appropriate sites and farming systems to ensure adequate water quality and flow, plus
treatment by air drying when necessary. Though primarily for marine farming, this is
applicable to abalone farming using other culture technology and in different
locations. As abalone farming expands into new locations and production increases it
is likely that severe spionid infestations will be encountered from time to time.
Vigilance will be required!
Strategies for avoidance
Farm site selection: Ideally this should be based on data on levels of mud worm
infection from pilot studies or other abalone sites in the area, but the findings above
suggest this may be confounded unless considerable data is available. Review of
general principles regarding spionid abundance suggest that suitable sites for abalone
culture should exclude very sheltered, static sites with muddy sediments near estuaries
and sources of organic pollution (Medcof 1945, Korringa 1951, Woodwick 1964,
Anger 1977, Pregenzer 1983, Baxter 1984, Smyth 1990). While sites with better water
flow will favour spionid settlement less, the broad dispersal pattern of planktotrophic
larvae means that sea based farms without these characteristics may require
deployment of stock to restricted to limited periods. Stock growth rates and cost
structures for sea based sites will determine whether individual farms use these sites
for all or part of the grow-out phase.
Cage design: The settlement intensity and impact of B. knoxi were seen to vary
considerably depending on the design of the abalone containment vessel, with
restricted flow at the time of settlement apparently restricting entry of planktotrophic
larvae, but favouring the local spread of P. hoplura via lecithotrophic larvae.
Restricted flow and possible secondary reduction in water quality can provide an
additional stress on infected abalone, increasing the detrimental effect on health.
Settlement of P. hoplura but not B. knoxi was greater on abalone reared near the
bottom than higher in the water column. Thus the design of rearing vessels, especially
with respect to water flow and their placement in the water column has implications
for farming of abalone in mud worm susceptible areas. Generally containers favouring
good water flow are preferable, but it has been suggested that cleaning of rearing
vessels could be timed to allow fouling to remain during the spring B. knoxi
settlement period, while ensuring maximum water flow over the warmer summer
months. This could be useful during the year following initial deployment. Containers
which restrict water flow favour local spread of species such as P. hoplura throughout
the year.Cage design may also affect whether abalone can be appropriately air dried in
situ within rearing vessels. This will depend largely on the degree of fouling acquiredby such vessels and their degree of enclosure. The Aquatek trays (section 4.3) with
lids removed allow appropriate drying of stock compared to more enclosed designs
such as tubes (section 4.3). Abalone culturists may find that air drying treatment is
best combined with other handling activity such as grading or thinning out of rearing
vessels. In such situations sheet plastic may serve as an appropriate substrate during
Cage position Vertical position of containers appears more critical for P. hoplura than
B. knoxi, with a general recommendation of good clearance from the sea floor which
may harbour wild reservoirs of infected shells, in a variety of mollusc species.
FRDC Final Report Page104
Clearance of 1 m was shown to be insufficient, as enhanced P. hoplura levels were
seen at this level, compared to 4 m clearance.
MANAGEMENT FOR AVOIDANCE
Timing of deployment of stock: The consistency of B. knoxi seasonal reproduction
fortuitously allows a simple avoidance strategy to be practiced in areas susceptible to
this species, by transfer of stock to susceptible sites post November. This allows
approximately 10 months growth before potential infestation in the following
September. Management strategies will necessarily be site specific and depend on the
stocking size, growth rate and marketed size of stock. Placement of stock at
approximately 25 mm in November/December may allow growth under ideal
conditions to approximately 55-60 mm during the next year. This represents the
probable minimum market size for abalone and stock grown under this regime would
thus be subjected to little B. knoxi influence. Where there is a requirement to grow
stock to larger sizes e.g. 80-100 mm, potential exposure to B. knoxi settlement in the
second year may necessitate treatment.
Larval settlement data for P. hoplura (section 5.1) suggested that initial
colonization with this species could substantially be avoided by placement of stock
post spring and preferably after mid summer. Once established in abalone, however,
the species showed the potential for year round lecithotrophic reproduction. Thus
numbers of this species have the potential to rise steadily over time and this needs to
be taken into account and monitored by growers – especially if the grow out period
extends over 2-3 years in the sea.
Monitoring of spionid levels and the effect on abalone health: Because exposure may
vary from year to year, and treatment constitutes an additional stress, monitoring of
mud worm levels and species and of the general condition of the animals, is essential
in areas with significant mud worm risk. This is to ensure treatment is utilised only
where warranted, but before the stress of heavy infection and of rising water
temperatures increases the treatment associated risks. As the effect of stressors on
energy metabolism is essentially additive, such monitoring will highlight situations
where intervention may be required earlier than schedules. The research findings
suggest in particular an interaction of mud worm associated mortality with
underfeeding, which may not otherwise be apparent.
Methods for mud worm control – treatment schedule
Treatment on needs basis:
Given the potential for air drying treatment to suppress growth of stock this
management option should be used prudently with the emphasis on avoidance of
infestation. Where treatment is required, stress to abalone will likely be minimised by
early intervention, reduced drying time, reduced air temperature and reduced
differential between sea and air temperatures. Additionally, Watanabe et al. (1994)
found that starved abalone survived air exposure at considerably greater levels than
fed animals.
As infestation with approximately 5 spionids may reduce growth by about
25% (sections 5.4.2 & 5.5.2) as may, potentially, air drying treatment (section 5.3)
infestation at this level or above is considered worth treating. In practice this decision
FRDC Final Report Page105
will be based on the duration of time remaining before stock can be sold and on the
spionid species involved. For instance, it may be counterproductive to treat recent
infestations if stock can be sold within a few months. Growers should also consider
that B. knoxi numbers will not increase in stock until the following spring dispersal
period whereas P. hoplura counts have the potential to rise steadily from a low level
of infestation to >50 per abalone in a 2 year period (section 5.5). It is recommended
that abalone infested with greater than 10 - 20 spionids early in the grow out phase be
treated by air drying as on balance the risks associated with not doing so appear
greater than the potential consequences of treatment side-effects.
Treatment by schedule:
Although the need for treatment should be monitored, and treatment brought
forward where necessary to avoid severe mud worm stress, practicalities of potentially
treating large numbers of tanks require a planned treatment schedule based on
predicted risk.
For B. knoxi susceptible sites, transfer of stock should be planned for post
November, which allows approximately 10 months growth before potential infestation
in the following September. To be fully effective, treatment is recommended within 6
months of infestation, but avoiding either low or high temperature extremes ( > 15 oC
but > 24oC) and excessive humidity should be avoided if possible, as outlined in the
treatment protocol. Therefore the first treatment should be scheduled for the early or
late summer of second summer at sea, with the actual month of treatment likely to
vary between locations. Stock placed at other times will also require treatment within
6 months of larval dispersal around September.
Where P. hoplura is the major problem, placement of stock post spring and
preferably after mid summer will minimise initial colonisation, but as this species has
the potential to rise steadily over time, predicting the time when treatment is needed is
less certain. Monitoring of this species is particularly important, especially if the grow
out period extends over 2-3 years in the sea.
6. BENEFITS
As identified in the original application, the sea cage sector of the abalone culture
industry is the major beneficiary of the research. This sector previously appeared non-
viable and affected the confidence of the abalone culture sector in Tasmania
generally. All the original sea based farms most affected by mud worm in the mid
1990's had ceased commercial abalone culture attempts by this method within a year
or two. The loss of confidence associated with the early mortality episodes has at least
been partly alleviated as a result of this project. A new and relatively large sea based
farm has been established since the project began and consulted with project staff on
mud worm risk assessment during the development phase. In the last year or two
further experimental or pilot scale sea based farms have begun to re-emerge in
Tasmania and new land based farms have been established.
The treatment regimes are also applicable to abalone in other farming sytems,
though these have greater options for avoidance of spionid infestations. Techniques
and data generated by the project have also been utilised by the oyster industry in
relation to mud worm assessment and risk management in relation to export of spat
FRDC Final Report Page106
from Tasmania. The data on health testing has contributed to the body of knowledge
on abalone health in the culture situation and will assist in future abalone health
investigations such as that concerning summer stress syndrome.
7. FURTHER DEVELOPMENT
The results of the research have been distributed directly to local industry via
regular attendance at Tasmanian Abalone Growers Association meetings and to the
wider abalone culture industry through the annual FRDC subprogram workshops.
Project results indicated severe mud worm infestations may not be common and
could possibly occur only once or twice a decade. Consequently, corporate memory of
the potential for spionid stock damage may be lost. This has in part been addressed by
production of a small handbook suitable for growers, which includes a pictorial guide
to early signs and progression of infestation, a species identification guide, notes on
spionid reproduction and a protocol for treatment (Lleonart, 2001). The farm guide
has been submitted to FRDC Abalone Aquaculture Subprogram to be supplied to all
existing farms and distributed by the state bodies to new grower members.
8. PLANNED OUTCOMES
The major project outputs are that mud worm infestations can be substantially
avoided in the first instance and treated if necessary. The major project outcome this
knowledge and a new sense of perspective regarding the risk of severe infestation has
produced has been a rise in investor confidence leading to renewed interest in sea
based abalone farming. This has contributed to the development of at least three new
sea based abalone farms in Tasmania.
In so far as this project incorporated a Ph.D. studentship, a further outcome is
the completion and submission of a Ph.D. Thesis based on this work (Lleonart, 2002),
and awarding of Doctor of Philosophy to Mark Lleonart.
Widespread stock mortality in Tasmanian sea cage farmed abalone prompted this
research project. The general objective of developing a sustainable means to control
mud worms in farmed abalone was met. Of the specific project objectives the
objective of investigation of the ecology and reproductive biology of spionids found
that the major focus of the project, Boccardia knoxi, had a strictly seasonal
reproductive cycle with larval settlement in the spring months. Thus post spring
placement of stock in susceptible areas allows up to a year's relief from the impacts of
infestation by this species.
Likewise, settlement of a further common spionid species Polydora hoplura was
mainly confined to the spring and summer allowing for an avoidance strategy. Unlike
B. knoxi, P. hoplura was found to be capable of near year round reproduction of larvae
once established in the shell. Thus, the numbers of this species infecting abalone have
FRDC Final Report Page107
the potential to rise steadily with grow out time whereas B. knoxi numbers only
increase in the spring. Three other spionid species were found in abalone, two of
which had not previously been reported from Tasmania. These species were present at
very low levels.
The original objectives relating to chemical treatments and their application
within the production cycle were altered to reflect the use of a non-chemical treatment
option. In addition, an original objective to examine the efficacy of antifoulant paints
could not be satisfactorily met as no application method suitable for abalone was
found. The use of air drying to kill mud worms in abalone rather than chemicals or
drugs was considered a very favourable outcome in terms of health and safety issues,
withholding periods, licensing issues and public perception.
Air exposure for 2-4 hours in shade at 15-21 °C and humidity less than
approximately 63% are the recommended treatment conditions. In laboratory and
field trials such treatment eliminated >90 % of infesting spionids present for less than
6 months. Spionid reduction in long term severely infested stock is possible but varies
with severity of blisters, size of stock and degree of fouling organisms present on
abalone shells. It is suggested growers in mud worm susceptible areas monitor stock
in mid summer to determine whether treatment is warranted. As there was some
evidence that drying treatment could depress growth in the medium-long term
avoidance is preferred in the first instance, followed by treatment, if required after
potential spionid exposure the following spring/summer. Treatment could be
combined with other handling activities such as grading, stock transfer or cage
A further specific project objective related to gathering of epidemiological
information on culture environment and stock characteristics. Experiments conducted
on stock characteristics showed that larger stock attracted more spionid settlement at
initial placement. Fouling organisms such as Pacific oysters and calcareous tube
building worms especially spirorbids substantially increased the risk of settlement by
B. knoxi mud worms. Abalone were found to be at increased risk of P. hoplura
infestation near the bottom than higher in the water column and rearing vessels with
reduced mesh area excluded planktonic B. knoxi larvae. A survey of the literature with
regard to spionid infestation in commercial bivalves suggests that abalone farms
should avoid areas with muddy or silty sediments, estuaries and locations susceptible
to organic pollution.
In conclusion the abalone mud worm project has shown that severe spionid
infestation is not as common as first feared following the mortality events of the mid
1990's; that infestation can be substantially avoided by appropriate management of
stock transfer times and stock selection; and that mud worm infestation can be
successfully treated if required. These findings have contributed to renewed
confidence in abalone farming in Tasmania, including the re-establishment of some
sea based farms. The knowledge that spionid infestation can be managed should
contribute to the abalone industry generally in the future as such outbreaks are likely
to occur again from time to time.
FRDC Final Report Page108
Anonymous, 1994. Draft plan for Huon River and Port Esperance. Department of
Primary Industry and Fisheries, Tasmania. November 1994. 147 pp.
Anonymous, 1997. Marine farming development plan D'Entrecasteaux Channel.
Department of Primary Industry and Fisheries, Tasmania. February 1997. 122 pp.
Anonymous, 2001. Fish Health Methods Manual. Fish Health Laboratory, Mt.
Pleasant Laboratories, DPIW&E Tasmania.
Almeida, M.J., Moura, G., Machado, J., Coimbra, J., Vilarinho, L, Ribeiro, C., and
Soares da Silva, P. 1996. Amino acid and metal content of Crassostrea gigas shell
infested by Polydora sp. in the prismatic layer, insoluble matrix and blister
membrane. Aquat. Living Resour. 9:179-186.
Anger, K. 1977. Benthic invertebrates as indicators of organic pollution in the western
Baltic Sea. Int. Revue ges. Hydrobiol. 62 (2): 245-254.
Anger, K., Anger, V. and Hagmeier, E. 1986. Laboratory studies on larval growth of
Polydora ligni, Polydora ciliata, and Pygospio elegans (Polychaeta, Spionidae).
Helgolander Meeresunters. 40, 377-395.
Ainslie, R.C.1980. Haemocyanin concentrations in field populations of three species
of southern Australian abalone. Aust. J. Mar. Freshw. Res., 31(5):627-633.
Bailey-Brock, J.H and Ringwood, A. 1982. Methods for control of the Mud blister
worm, Polydora websteri, in Hawaiian oyster culture. University of Hawaii Sea Grant
Program. Volume 4, No. 3. 6 pp.
Barkai, R. and Griffiths, C. 1987. Consumption, absorption efficiency, respiration and
excretion in the South African abalone Haliotis midae. The Benguela and comparable
ecosystems. Payne, A., Gulland, J. and Brink, K. (Eds.). S. Afr. J. Mar. Sci. 5:523-
Baxter, J.M. 1984. The incidence of Polydora ciliata and Cliona celata boring the
shell of Patella in Orkney. J. Mar. Biol. Assoc. UK. 64: 728-729.
Bayne, C.J 1983. Molluscan immunobiology. In: Hochachka, P.W. (Editor), The
Mollusca Vol 5, Physiology, Part 2. Academic Press, New York. pp.408-466.
Bevelander, G. 1988. Abalone gross and fine structure. The Boxwood Press.
Blake, J.A. 1969. Reproduction and larval development of Polydora from northern
New England (Polychaeta: Spionidae). Ophelia, 7: 1-63.
Blake, J.A and Evans, J.W. 1972. Polydora and related genera as borers in mollusc
shells and other calcareous substrates. The Veliger 15: 235-249
FRDC Final Report Page109
Blake, J.A. and Kudenov, J.D. 1978. The spionidae (polychaeta) from southeastern
Australia and adjacent areas with a revision of the genera. Mem. Natl. Museum
Blake, J.A and Kudenov, J.D. 1981. Larval development, larval nutrition and growth
for two Boccardia species (Polychaeta: Spionidae) from Victoria, Australia. Marine
Ecology Progress Series Volume 6:175-182.
Boarder, S.J. 1997. Effects of dietary vitamin and mineral inclusion levels on the
greenlip abalone Haliotis laevigata Donovan. Honours thesis, University of Tasmania,
Bonaventura, C and Bonaventura, J. 1983. Respiratory pigments: Structure and
function In: Hochachka, P.W. (Editor), The Mollusca, Vol. 2. Environmental
biochemistry and physiology. Academic Press, New York, pp. 1-50.
Brandal, P.O and Egidius, E. 1979. Treatment of salmon lice (Lepeophtheirus
salmonis) with neguvon- description of method and equipment. Aquaculture, 18: 183-
Burton, R.F. 1983. Ionic regulation and water balance. In: Saeuddin, A.S.M. and
Wilbur, K.M., (Editors). The Mollusca Vol. 5, Physiology Part 2. Academic Press,
Sydney, pp. 291-352
Caceres-Martinez, J., Macias-Montes de Oca, P., and Vasquez-Yeomans, R.1998.
Polydora sp. infestation and health of the Pacific Oyster Crassostrea gigas cultured in
Baja California, NW Mexico. J. Shellfish Res. 17(1): 259-264.
Caceres-Martinez, J., Tinico, G.D., Bustamente, M.L. and Gomez-Humaran, I.M.
1999. Relationship between the burrowing worm Polydora sp. and the black clam
Chione fluctifraga Showerby. J. Shellfish Res. 19( 1): 85-89.
Cameron, J.N. 1986. Principles of physiological measurements. Academic Press
(London) pp 278.
Carefoot, T., Qian, P., Taylor, B., West, T. and Osborne, J. 1993. Effect of starvation
on energy reserves and metabolism in the Northern abalone, Haliotis Kamtschatkana.
Aquaculture 118: 315-325.
Cheng, T.C. and Lee, F.O. 1971. Glucose levels in the mollusc Biomphalaria
glabrata infected with Schistosoma mansoni. J. Invert. Path. 18: 395-399.
Clavier, J. 1989. Infestation of Haliotis tuberculata shells by Cliona celata and
Polydora species. In abalone of the world, biology, fisheries and culture-
supplementary papers. Proceedings of the 1 st international symposium on abalone.
La Pez, Mexico 21-25 November 1989. Shepherd, S.A., Tegner, M.J and Guzman del
Proo, S.A (Editors).pp16-20.
Cobas –MIRA, 1987. Roche: Cobas-MIRA – Scientific Methods Manual. Roche Inc.,
New York, 293 pp.
FRDC Final Report Page110
Cross, G.M and Needham, D. J. 1988. Disease control and therapeutics In Fish
Diseases, Refresher Course for Veterinarians. Proceedings 106, 23-27 May 1988, pp.
Day, J.H. 1967. A monograph on the polychaeta of southern Africa Part 2 Sedentaria.
Trustees of the British Museum (Natural History) London 656: 459-878.
Dinamani, P. 1986. Potential disease causing organisms associated with mantle cavity
of Pacific oyster Crassostrea gigas in northern New Zealand. Dis. Aquat. Org. 2: 55-
Dorsett, D.A. 1961. The reproduction and maintenance of Polydora ciliata Johnston
at Whitstable. Mar. Biol. Assoc. UK 41: 383-396.
Edwards, S.J. 1996. Effects of handling on oxygen consumption of Australian
abalone.Proceedings of the 3 RD annual abalone aquaculture workshop, Port Lincoln.
Edwards, S.J., Burke, C., Hindrum, S. and Johns, D. 2000. Recovery and growth of
anaesthetic and mechanical removal on greenlip (Haliotis laevigata) and blacklip
(Haliotis rubra) abalone. J. Shellfish Res. 19 (1): 510 (Abstract).
Ellis, R. 1992. Histopathology Notebook. A manual of methodology for the histology
laboratory with safety in mind. Queen Elizabeth Hospital, Woodville South Australia
Fallu, R. 1991 Abalone Farming. Fishing news books. England. 195 pp.
Fauchald, K. 1977. The polychaete worms: Definitions and keys to the Orders,
Families and Genera. Natural History Museum of Los Angeles County In conjunction
with The Allan Hancock Foundation University of Southern California. Science series
Fleming, A. and Hone, P. 2001. Abalone aquaculture. In Australian aquaculture
yearbook 2001, National Aquaculture Council, pp 33-36.
Friedman, C.S., Robbins, T., Jacobsen, J.L., and Shield, J.D. 2000. The cellular
immune response of black abalone, Haliotis cracherodii Leach, with and without
withering syndrome. J. Shellfish Res. 19(1): 514 (Abstract).
Gade, G. 1988. Energy metabolism during anoxia and recovery in shell adductor and
foot muscle of the gastropod mollusc Haliotis lamellosa: formation of the novel
anaerobic end product tauropine. Bio. Bull. 175: 122-131.
Gardner, R.G., Harshbarger, J.C., Lake, J.L., Sawyer, T.K., Price, K.L., Stephenson,
M.D., Haaker, P.L, and Togstad, H.A. 1995. Association of prokaryotes with
symptomatic appearance of withering syndrome in black abalone Haliotis
cracherodii. J. Invert. Path. 66: 111-120.
FRDC Final Report Page111
Gaty, G. and Wilson, J. (1986) Effect of body size, starvation, temperature and
oxygen tension on the oxygen consumption of hatchery reared ormers Haliotis
tuberculata L. Aquaculture 56: 229-237.
Grindley, R.M., Keogh, J. A., and Friedman, C.S. 1998. Shell lesions in New Zealand
Haliotis sp. (Mollusca, Gastropoda). J. Shellfish Res. 17(3): 805-811.
Gromadzki, A. 1994. Light as an ecological factor in the dispersal of spionid
polychaetes infecting Pacific Oysters. Honours thesis, Department Marine Biology,
Flinders University South Australia. 52 pp.
Hahn, K.O., 1989. Survey of the commercially important abalone species in the
world. In: Culture of abalone and other marine gastropods, Hahn, K.O. (Editor) CRC
Press, Boca Raton, Florida. 335 pp.
Handley, S.J. 1997. Spionid polychaete worm infestations of the Pacific oyster
Crassostrea gigas in New Zealand. Ph.D. Thesis University of Auckland. June 1997.
Handley, S.J. and Bergquist, P.R. 1997. Spionid polychaete infestations of intertidal
pacific oysters Crassostrea gigas (Thunberg), Mahurangi Harbour, northern New
Zealand. Aquaculture 153: 191-205.
Handley, S.J. 2000. Larval development of Boccardia knoxi, a shell infesting spionid
polychaete. NZ J. Mar. Freshwater Res. 34: 681-686.
Hansen, J.C. 1970. Commensal activity as a function of age in two species of
California abalones. The Veliger. Vol. 13(1): 90-94.
Harris, J.O. 1999. Chronic effects of adverse water quality on the greenlip abalone
Haliotis Laevigata Donovan. Ph.D. Thesis, University of Tasmania, 1999, pp. 143.
Harris, J.O., Maguire, G. B., and Handlinger, J.H. 1998. Effects of chronic exposure
of greenlip abalone, Haliotis laevigata Donovan, to high ammonia, nitrite, and low
dissolved oxygen concentration on gill and kidney structure. J. Shellfish Res. 17(3):
Harris, J.O., Maguire, G. B., and Handlinger, J.H. 1998. Effect of ammonia on the
growth rate and oxygen consumption of juvenile greenlip abalone, Haliotis laevigata
Donovan. Aquaculture 160: 259-272
Hindrum, S.M. 1996. Performance of greenlip (Haliotis laevigata) and
Blacklip*Greenlip hybrid abalone in land based or sea based production systems.
Proceedings of the 3rd annual abalone aquaculture workshop, Port Lincoln (August).
Hopkins, S.H. 1958. The planktonic larvae of Polydora websteri Hartman (Annelida,
Polychaeta) and their settling on oysters. Bull. Mar. Sci. Gulf Caribbean 8: 268-277.
Horne, G. KTFC Abalone project report. Produced for Warren Nagata and the
Kwakiutl territorial fisheries commission. March 27, 1996. 33 pp.
FRDC Final Report Page112
Hutchings, P.A aand Turvey, S.P. 1984. The spionidae of South Australia (Annelida:
Polychaeta). Trans. R. Soc. S. Aust. 108(1): 1-20.
Jorgensen, D.D, Ware, S.K and Redmond, J.R. 1984. Cardiac output and tissue blood
flow in the abalone, Haliotis cracherodii (Mollusca, Gastropoda). J. Exp. Zool.
Kirby, E.C. and Baker, S.W 1995. Earthworm population, casting and control in
sports turf areas: a review. J. Sports Turf Res. Inst. 71: 84-98
Kismohandaka, G., Friedman, C.S., Roberts, W., and Hedrick, R.P. 1993.
Investigation of physiological parameters of black abalone with withering syndrome.
J. Shellfish Res. 12:131-132. (Abstract).
Kismohandaka, G., Friedman, C.S., Roberts, W., and Hedrick, R.P. 1995.
Physiological alterations of the black abalone, Haliotis cracherodii Leach, with
withering syndrome. Triennial meeting of fish culture section of American fisheries
society. 14 (1): 269-270. (Abstract).
Kent, R.M. 1979. The influence of heavy infestations of Polydora ciliata on the flesh
content of Mytilus edulis. J.Mar. Biol. Ass. U.K 59: 289-297
Kojima, H. and Imajima, M. 1982. Burrowing polychaetes in the shells of the abalone
Haliotis diversicolor aquatalis Chiefly on the species of Polydora. Bulletin of the
Japanese Society of Scientific Fisheries 48(1) 31-35. English Abstract, text in
Japanese. Entire paper translated to English by Atsuko Byakuno, 1999.
Korringa, P. 1951. The shell of Ostrea edulis as a habitat. Arch. Neer. Zool. 10:32-
Korringa, P. 1952. Recent advances in oyster biology. Quart. Rev. Bio. 27(4): 339-
Langdon, J.S. 1990. Major parasitic diseases of Australian finfish, In fin fish diseases,
refresher course for veterinarians, Proceedings 128. pp 233-256.
Lauckner, G. 1983. Diseases of Mollusca: Bivalvia, Agents: Annelida. In Kinne, O.
(Editor), Diseases of marine animals Volume 2. Biologische Anstalt Helgoland
Hamburg, pp. 805-816.
Lee, F.O and Cheng, T.C. 1972. Schistosoma mansoni: alterations in total protein and
haemoglobin in the haemolymph of infected Biomphalaria glabrata . Exp. Parasitol.
31: 203-216.
Leighton, D.L. 1998. Control of sabellid infestation in green and pink abalones,
Haliotis fulgens and H. corrugata, by exposure to elevated water temperatures. J.
Shellfish Res.17 (3): 701-705.
Lester, R.J. 1988. Metazoan diseases of fish. In Fish Diseases, Refresher Course for
Veterinarians. Proceedings 106, 23-27 May 1988, pp. 115-126
FRDC Final Report Page113
Livingston, D.R. and De Zwaan, A. 1983. Carbohydrate metabolism of gastropods.
In: The Mollusca Volume 1, Metabolic biochemistry and molecular biomechanics.
Hochachka, P.W. (Editor). Academic Press. pp 177-230.
Lucas, A. and Beninger, P.G.1985. The use of physiological condition indices in
marine bivalve aquaculture. Aquaculture 44: 187-200.
Mackenzie, C.L and Shearer, L.W. 1959. Chemical control of Polydora websteri and
other annelids inhabiting oyster shells. Proc. Nat. Shellfisheries Assoc. 50: 105-111
Malek, E.A and Cheng, T.C. 1974 Medical and economic malacology. Academic
Press. New York. 383 pp.
Mance, G. 1987. Pollution threat of heavy metals in aquatic environments. Elsevier
Publishers. pp. 193-205
Medcof, J.C.1945. The mud-blister worm, Polydora, in Canadian oysters.
J. Fish. Res. Bd. Can. 6 (7): 498-505.
The Merck Index. 1989. An encyclopaedia of chemicals drugs and biologicals.
Eleventh edition. Budavari, S. (Editor). 1989. Merck & Co. Inc.
Nel, R., Coetzee, P.S., Niekerk, G.V. 1996. The evaluation of two methods to reduce
mud worm (Polydora hoplura Claparede) infestation in commercially reared oysters
(Crassostrea gigas Thunberg). Aquaculture 141:31-39.
Nell, J. and Smith, G.S. 1988. Management, production and disease interactions in
oyster culture In Fish Diseases, Refresher Course for Veterinarians. Proceedings 106,
23-27 May 1988, pp.127-134.
Nimura, Y. and Yamakawa, H. 1989. Oxygen uptake rate and heart rate of small
abalone Sulculus supertexta as related to the ambient oxygen concentration. Nippon
Suisan Gakkaishi 55(10): 1869.
Overweter, A. 2000. Microencapsulated chemotherapy against fouling worm of
abalone. Honours thesis, University of Tasmania, 53 p.
Owen, H.M. 1957. Etiological studies on oyster mortality.(ii) Polydora websteri
Hartmann-(Polychaeta: Spionidae). Bull. Mar. Sci. Gulf Caribbean 7:35-46.
Owens, L; Glazebrook, P.W; Ladds, P.W and Campbell, R.S. 1988. Disease in
tropical mariculture in Australia. In Fish Diseases, Refresher Course for
Veterinarians. Proceedings 106, 23-27 May1988, pp. 375-416
Paul, A. and Paul, J. 1998. Respiration rate and thermal tolerances of pinto abalone
Haliotis kamtschatkana. J. Shellfish Res. 17(3): 743-745.
Pauley, G.B. and Krassner, S.M. 1971. The effect of temperature on the number of
circulating hemocytes in the California sea hare, Aplysia californica. California fish
and game 57: 308-309
FRDC Final Report Page114
Pregenzer, C. 1983. Survey of metazoan symbionts of Mytilus edulis (Molllusca:
Pelecypoda) in Southern Tasmania. Aust. J. Freshwater Res. 34: 387-396.
Radashevsky, V. I. 1994. Life history of a new Polydora species from the Kurile
Islands and evolution of lecithotrophy in polydorid genera (Polychaeta: Spionidae).
Ophelia 39 (2): 121-136.
Rainer, S. 1973. Polydora and related genera (Polychaeta: Spionidae) from Otago
waters. J. Royal Soc. NZ 3(4): 545-564.
Read, G. B. 1975. Systematics and biology of polydorid species (Polychaeta:
Spionidae) from Wellington Harbour. J. Royal Soc. NZ 5(4): 395-419.
Rouse, G.W. 2000. Morphology and Physiology pp. 23 in Beesley, P.L, Ross, G.J.B
and Glasby, C.J. (Editors) Polychaetes and Allies: The southern synthesis. Fauna of
Australia. Vol 4A Polychaeta, Myzostomida, Pogonophora, Echiura, Sipuncula.
CSIRO Publishing: Melbourne 465 p.
Russell, C.W. and Evans, B.K. 1989. Cardiovascular anatomy and physiology of the
black-lip abalone, Haliotis ruber. J. Exp. Zool. 252:105-117.
Ruck, K.R. and Cook, P.A. 1999. Polychaete worms : a threat to abalone farming?
Book of abstracts, The annual international conference and exposition of the world
aquaculture society. 26 April-2 May Sydney, Australia. p. 658 (Abstract).
Ruck, K.R. and Cook, P.A. 1998. Sabellid infestations in the shells of South African
molluscs: implications for abalone mariculture. J. Shellfish Res. 17(3): 693-699.
Ryan, F.B. 2000. Towards the maintenance of an ecologically sustainable Pacific
Oyster industry. Final Report for FRDC Project Number T93/222.
Sahaphong, S., Linthong, V., Wanichanon, C., Riengrojpitak, S., Kangwanrangsan,
N., Viyanant, V., Upatham, S.E., N., Pumthong, T. Chansue, N., and Sobhon, P.
2001. Morphofuctional study of the hemocytes of Haliotis asinina. J. Shellfish Res.
20(2): 711-716.
Sato-Okoshi, W. and Nomura, T. 1990. Infestation of the Japanese scallop
Patinopecten yessoensis by the boring polychaetes Polydora on the coast of Hokkaido
and Tohoku district. Nippon Suisan Gakkaishi 56(10): 1593-1598. (English abstract,
text in Japanese).
Segawa, S. (1991). Oxygen consumption and ammonia excretion by the abalone
Sulculcus diversicolor aquatalis in starved condition. Nippon Suisan Gakkaishi
57(11): 2001-2006. In Japanese with English abstract.
Shepherd, S.A. 1973. Studies on southern Australian abalone (genus Haliotis).
1.Ecology of five sympatric species. Aust. J. Mar. Freshwat. Res., 24:217-257.
Shepherd, S.A., and Hearn, W.S.1983. Studies on southern Australian abalone (Genus
Haliotis). 4 Growth of H. laevigata and H. ruber. Aust. J. Mar. Freshwat. Res.
FRDC Final Report Page115
Shields, J.D., Perkins, F.O. and Friedman, C.S. 1996. Hematological pathology of
wasting syndrome in black abalone. J. Shellfish Res. 15:498. (Abstract)
Shields, J.D, Buchal, M., Morre, J. and Friedman, C.S. 1997. Liposome encapsulation
as a potential control technique against sabellid worms. Third international abalone
symposium, Monterey, CA, Oct. 26-31, 1997. (Abstract).
Simkiss, K. and Mason, A.Z. 1983. Metal ions: metabolic and toxic effects, p.117 in:
The Mollusca Volume 2 Environmental biochemistry and physiology. Hochachka,
P.W. (Editor). Academic Press. New York.
Sinclair, M. 1963 Studies on the paua Haliotis iris (Martyn) in the Wellington district
1945-46. Zool. Publ. Univ. Wellington, No.35
Skeel, M. E. 1979. Shell-boring worms (Spionidae: Polychaeta) infecting cultivated
bivalve molluscs in Australia. Proc. World Maricul. Soc. 10: 529-533.
Smith, G.S.1982. What happened to the Queensland oyster industry? A lesson from
history. Australian Fisheries, March. pp 41-45.
Smith. I.R.1984. Diseases important in the culture of the Sydney Rock Oyster. Report
of the Brackish Water Fish Culture Research Station, NSW Department of
Agriculture. 10 pp.
Smyth, M.J. 1989. Bioerosion of gastropod shells: with emphasis on effects of
coralline algal cover and shell microstructure. Coral Reefs 8: 119-125.
Smyth, M.J. 1990. Incidence of boring organisms in gastropod shells on reefs around
Guam. Bull. Mar. Sci. 46(2): 432-449.
Sokal, R.R and Rohlf, F.J. 1995. Biometry The principles and practice of statistics in
biological research. Third Edition. WH Freeman and Co. New York. 887 pp.
Stephen, D.1970. Mud blister formation by Polydora ciliata in the Indian backwater
oyster Crassostrea madrasensis (Preston). Aquaculture, 13: 347-350.
Stumpf, J.L and Gilbertson, D.E. 1980. Differential leukocytic responses of
Biomphalaria glabrata to infection with Schistosoma mansoni. J. Invertebrate
Pathology 35: 217-218. Cited by Bayne, C.J. 1983 in Molluscan immunobiology. In:
Hochachka, P.W. (Editor), The Mollusca Vol 5, Physiology, Part 2. Academic Press,
New York. pp.408-466.
Tissot, B.N. 1992. Water movement and the ecology and evolution of the Haliotidae.
In: Shepherd, S.A Tegner M.J and Guzman del Proo S.A (Editors), Abalone of the
world, biology, fisheries and culture. Fishing News Books, Oxford, pp. 34-48
Tjeerdema, R. S., Kauten, R. J and Crosby, D. G. 1991. Sublethal effects of hypoxia
in the abalone (Haliotis rufescens) as measured by in vivo 31P NMR spectroscopy.
Comp. Biochem. Physiol. Vol. 100B(4): 653-659.
FRDC Final Report Page116
Tonkin, G. and Tonkin, S. 1997. Polydora (alias mudworm) the story of a little worm
with a big message. Intra industry paper. 5 pp.
Uki, N. and Kikuchi, S. 1975. Oxygen consumption of the abalone, Haliotis discus
hannai in relation to body size and temperature. Bull. Tohoku Reg. Fish. Res. Lab.
Verdouw, H., van Echteld, C., and Dekkers, E. 1978. Ammonia determination based
on indophenol formation with sodium salicylate. Water Res. 12: 399-402
Wargo, R.N. and Ford, S.E. 1993. The effect of shell infestation by Polydora sp. and
infection by Haplosporidium nelsoni (MSX) on the tissue condition of oysters,
Crassostrea virginica. Estuaries 16 (2): 229-234
Watanabe, H., Yamanaka, H. and Yamakawa, H. 1992. Seasonal variations of
extractive components in the muscle of disk abalone. Nippon Suisan Djakarta 58(5):
Watanabe, H., Yamanaka, H., and Yamakawa, H. 1994. Influences of hypoxia on
changes in content of glycolytic metabolites in the muscle of juvenile disk abalone fed
with different algae or starved. Nippon Suisan Gakkaishi 60(2): 241-246. (In Japanese
with English abstract and tables).
Wells, R.M.G., and Baldwin, J. 1995. A comparison of metabolic stress during air
exposure in two species of New Zealand abalone, Haliotis iris and Haliotis australis:
implications for the handling and shipping of live animals. Aquaculture 134: 361-370.
Whang, H.O and Chung , K.O, 1977. Study on relationship between exposure time
and mortality of young abalone, Haliotis discus (Reeve). Bull. Fish. Res. Dev. Agency
18: 123-129. (In Japanese with English summary and captions).
Whitlegge, T. 1890. Report on the worm disease affecting the oysters on the coast of
New South Wales. Rec. Aust. Museum 1:1-15.
Wilson, D. P. 1928. The larvae of Polydora ciliata Johnston and Polydora hoplura
Claparede. J. Mar. Biol. Assoc. UK. 15: 567-603.
Wilson, J., Handlinger, J. and Sumner, C.E. 1993. The health status of Tasmania's
bivalve shellfish. Technical Report No. 47. Sea Fisheries Division, Marine Research
Laboratories, Department of Primary Industry and Fisheries, Tasmania. 63 pp.
Wislocki, P.G., Grosso, L.S and Dybas, R.A. 1989. Environmental aspects of
abamectin use in crop protection. In Ivermectin and Abamectin. Cambell, W.C
(editor). Springer-Verlag N.Y. pp. 183-200.
Woodwick, K.H. 1964. Polydora and related genera (Annelida, Polychaeta) from
Eniwetok, Majuro, and Bikini Atolls, Marshall Islands. Pacific Sci. 18: 146-159.
Woodwick, K.H. 1977. Lecithotrophic larval development in Boccardia proboscidea
Hartman. – In D.J Reish and K. Fauchald (Editors): Essays on polychaetous annelids
FRDC Final Report Page117
in memory of Dr. Olga Hartman. Allan Hancock Foundation, University of Southern
California, Los Angeles. pp. 347-371
Zar, J. 1984. Biostatistical analysis. Prentice-Hall, Inc. N.J. 718 pp.
Zottoli, R.A. and Carriker, M.R 1974. Burrow morphology, tube formation and
microarchitecture of shell dissolution by the spionid polychaete Polydora websteri.
Mar. Biol. 27: 307-316.
Workshops and seminars
Lleonart, M. 1997 Progress on the DPIF studies on mudworm distribution
1997. Proceedings of the 4th Annual FRDC/CRC Abalone Workshop, Port Fairy.
Lleonart, M and Handlinger, J. 1998. Treatment of Abalone "mud worms".
Proceedings of the Fifth Annual Abalone Culture Workshop Hobart, July 1998.
Lleonart, M. 1999. Mudworm Treatment Trials. Proceedings of the 6th Annual
Abalone Aquaculture Workshop, Sydney,April 1999.
Lleonart, M. 1999. Abalone Mudworm Field Studies on Infection and
Progression Patterns. Proceedings of the 6th Annual Abalone Aquaculture
Workshop Sydney, April 1999.
Lleonart, M. 2000. Mudworms and seabased abalone culture in Tasmania.
Proceedings of the 7th Annual Abalone Aquaculture Workshop, Dunedin. August
Lleonart, M., Handlinger, J., and Powell, M. 2001. Overview of mud worm research
1997- 2001. Proceedings of the 8th Annual Abalone Aquaculture Workshop,
Freemantle, July 2001.
Lleonart, M. 2001. Australian Abalone Mudworms: avoidance & identification. A
Farm Manual. FRDC Abalone Aquaculture Subprogram.
Lleonart. M. 2002. Management of spionid mud worm infestations of Tasmanian
cultured abalone. Ph.D. Thesis, University of Tasmania.
Lleonart, M., Handlinger, J. and Powell, M. 2003. Treatment of spionid mudworm
(Boccardia knoxi Rainer) infestation of cultured abalone. Aquaculture 217:1-10.
Farm Manual
AUSTRALIAN ABALONE MUDWORMS: AVOIDANCE & IDENTIFICATION.
FRDC Final Report Page118
APPENDIX 1: Intellectual Property
There are no intellectual property issues associated with this project.
APPENDIX 2: Staff
Handlinger, Judith – Principal Investigator, TAFI Fish Health Unit
Powell, Mark - Academic supervisor, TAFI, University of Tasmania
Lleonart, Mark - PhD student Uni of Tasmania
O'Brien, Dominic – Huon Aquaculture Company P/L
Lee, Peter - Aquatas P/L
Cieura, Joe - Aquatas P/L
Finnigan ,Greg - Technical Officer, TAFI
FRDC Final Report Page119
APPENDIX 3 (Raw data & statistics)
Section 1: Pre-project data for Huon Aquaculture Company
1A: Mortality data for Huon Aquaculture Company, 1994-1996.
1996 TOTALS %kill
1996 TOTALS %kill
1996 TOTALS %kill
FRDC Final Report Page120
1A Figure 1. Cumulative mortality for June 1994 abalone intake at Huon AquacultureCompany.
ortality
m 20
ulative % 10
cum 5
1B Subjective shell damage ratings (SSDR) for remnant Huon Aquaculture
Company abalone sampled 1997-1998.
SSDR
n = 149, shell kept and assessed retrospectively May 2001
1C Annual Pacific Oyster blister frequency data
% stock with shell blisters
# Data from Ryan. (2000)
* Data from Wilson et al. (1993)
Other data from DPIWE Fish Health Lab records.
1D SSDR scores for wild abalone from Tasmania (Taroona collection)
(% values in parentheses)
SSDR
n=490, some shell damage may be due to boring sponge
Chi-square analysis found significant difference between species (P<0.001)
FRDC Final Report Page121
1E Comparison of SSDR scores between stunted and normal
population samples (Taroona collection)
(% values in parentheses)SSDR score
Chi-square analysis found significant difference between size groups (P<0.05)
Section 5: Chemical Immersion treatment screening data]
5A Fresh water
Spionids in vitro Time (min)Survival
48 h post treatment
Temperature 18 °C
Spionids in situ, X (SD) Fresh water Immersion Time (min.)
Mean surviving B. knoxi
Mean B. knoxi (EI)% Kill
(GMC)% Kill –B. knoxi
Mean survival – Total
(GMC)%Kill – Total worms
% Abalone mortality
n=10 all treatments (5/10 live in control group)
5B Potassium permanganate
Spionids in vitro Concentration KMnO4 (mg.l-1)Survival
7 d post treatment
Temperature 18 °C
FRDC Final Report Page122
Spionids in situ, X (SD) Concentration KMnO4 (mg.l-1)
Mean surviving B. knoxi
(GMC)% Kill –B. knoxi
Mean survival – Total
(GMC)%Kill – Total worms
% Abalone mortality
KMnO4 toxicity to abalone. Combined data for 3 exposure time and concentration
experiments.
40-50 mm abalone
Mortality 14 d post treatment (3 h)
Mortality 16 d post treatment (4 h)
Mortality 8 d post treatment (9 h)
5C Gentian violet
Spionids in vitro Concentration of Gentian Violet (mg.l-1)Survival
Immediate post treatment
4 d post treatment
Temperature 15°C
Spionids in situ, X (SD) Concentration gentian violet (mg.l-1)
Mean surviving B. knoxi
(GMC)% Kill -B. knoxi
Mean survival – Total
(GMC)%Kill -Total worms
Gentian violet toxicity to abalone. Data for 2 exposure time and concentration
experiments40-50 mm abalone
10 mg.l-1 20 mg.l-1
Mortality after 17 d (3 h
Mortality after 11 d (9 h
FRDC Final Report Page123
Spionids in situ Concentration of mebendazole (mg.l-1)Survival
Immediate post treatment
9 days post treatment
Temperature 16 °CSpionids in situ, X (SD) Concentration of Mebendazole (mg.l-1)
mean surviving B. knoxi
(GMC)% Kill -B. knoxi
mean survival – Total worms 8.8(9.6)
(GMC)%Kill -Total worms
Mebendazole toxicity to abalone. Combined data one week post exposure for
three exposure time and concentration experiments40-50 mm abalone
Spionids in vitro Concentration of fenbendazole (mg.l-1)Survival
Immediate post treatment
10 Days post treatment
Temperature 15 °C
Spionids in situ, X (SD) Concentration of fenbendazole (mg.l-1)
Mean surviving B. knoxi
(GMC)% Kill -B. knoxi
Mean survival – Total
(GMC)%Kill -Total worms
Fenbendazole toxicity to abalone (3 h exposure)40-50 mm abalone
Mortality 19 d post treatment
FRDC Final Report Page124
5F Levamisole
Spionids in vitro. Combined trial data. Concentration levamisole (mg.l-1)Survival
Trial 1 Immediate post
8 d post treatment
Trial 2 Immediate post
8 d post treatment
Temperature 15 -16°C
Spionids in situ. X (SD) Concentration of levamisole (mg.l-1)
2 h dry & 64 mg.l-1
mean surviving B. knoxi
(GMC)% Kill -B. knoxi
mean survival - Total worms 30.6(14.5)
(GMC)%Kill - Total worms
% Abalone mortality
n=10 infested shells, n=5-6 abalone mortality data
Levamisole exposure data at 320 mg.l-1, mudworms in situ, X (SD), n=20
Mean surviving B. knoxi
(GMC)% Kill -B. knoxi
Mean survival - Total worms 8.8(9.6)
(GMC)%Kill –Total worms
Levamisole toxicity to abalone (exposure 3 h). Combined data for two stock sizes40-50 mm abalone
0.32 mg.l-1 3.2 mg.l-1 32.0 mg.l-1
Mortality 18 d post
64 mg.l-1 320 mg.l-1 512 mg.l-1
Mortality 8 d post treatment
5G Malachite green
Malachite green toxicity to abalone data (exposure time 3 h)18-20 mm abalone
Mortality 8 days post
FRDC Final Report Page125
Spionids in situ and in vitro, X (SD) Concentration malachite green (mg.l-1)
Mean surviving B. knoxi
(GMC)% Kill -B. knoxi
Mean survival – Total
(GMC)%Kill – Total worms
Spionid survival immed. post 22/22
Spionid survival 7 post treat. 22/22
n=10 each abalone treatment
Spionids in vitro Concentration of trichlorofon (mg.l-1)Survival
Immediate post treatment
8 d post treatment
Temperature 13 °C
Trichlorofon toxicity to abalone (exposure time 3 h)40-50 mm abalone
Mortality 11 d post treatment 0/5
Spionids in vitro Concentration of Praziquantel (mg.l-1)Survival
Immediate post treatment
20 d post treatment
Temperature 14 °C
5J Hydrogen peroxide
Spionids in vitro Concentration of Hydrogen Peroxide (ppm)Survival
Immediate post treatment
9 d post treatment
Temperature 15 °C
Hydrogen peroxide toxicity to abalone (exposure time 3 h)40-50 mm abalone
50 mg.l-1 200 mg.l-1
Mortality 14 d post
FRDC Final Report Page126
5K Formalin
Formalin toxicity to abalone ( 3h exposure)18-20 mm abalone
100 mg.l-1 200 mg.l-1
Mortality after 7 d
Spionids in vitro and in situ, X (SD). Concentration of Formalin (ppm)
mean surviving B. knoxi
(GMC)% Kill -B. knoxi
mean survival - Total worms 39.1(21.9)
(GMC)%Kill - Total worms
spionid survival immed. post 5/5
Spionid survival 8 d post
Spionids in situ, X (SD). Concentration Ivermectin (mg.l-1)
Mean surviving B. knoxi
(GMC)% Kill –B. knoxi
Mean survival – Total
(GMC)%Kill – Total worms
% Abalone mortality
n=10 infected abalone, n=13 for mortality data
Spionids in vitro Concentration of Ivermectin (mg.l-1)Survival
Immediate post treatment
7 d post treatment
Temperature 16°C
Ivermectin toxicity to abalone data ( 3 h exposure)40-50 mm abalone
0.05 mg.l-1 0.1 mg.l-1 0.2
Mortality after 18 d
FRDC Final Report Page127
5M Exposure to febantel, pyrantel embonate and praziquantel in combination.
Spionids in vitro Concentration of Febantel and Pyrantel Embonate (mg.l-1) *
Survival
50 & 28.8 125 & 72 250 & 144
Immediate post treatment
8 d post treatment
* Febantel concentration shown first in column headings, praziquantel not shown as
previously shown ineffective. Temperature 15°C
Abalone mortality data. Exposure to febantel, pyrantel
embonateand praziquantel in combination40-50 mm abalone
250mg/ l & 144 mg.l-1 *
Mortality after 15 d
* Febantel concentration shown first, praziquantel not shown as above.
5N Metronidazole & Dimetronidazole
Spionids in vitro Concentration metronidazole (mg.l-1)Survival
48 hr post treatment
Temperature 18 °C
Spionids in vitro Concentration dimetronidazole (mg.l-1)Survival
Immediate post treatment
8 d post treatment
Temperature 16 °C (but with large fluctuation)
5O Methylene blue
Spionids in situ, X (SD). Concentration of methylene Blue (mg.l-1)
Mean surviving B. knoxi
(GMC)% Kill -B. knoxi
mean survival – Total worms 30.9(7.2)
(GMC)%Kill – Total worms
% Abalone mortality
n=10 infected shells, n=3 mortality data
Spionids in vitro Concentration methylene Blue (mg.l-1)Survival
Immediate post treatment
7 d post treatment
Temperature 18 °C
FRDC Final Report Page128
5P [Section 5.6 Health (1)]
Regression analysis Total blister Vs shell length
Estimates of parameters
Significance of r value: r 0.05, 47 = 0.288, r critical 0.288 < 0.648 (r observed ), so is
5Q [Section 5.6 Health (1)]
Regression analysis Total blister Vs whole weight
Estimates of parameters
Significance of r value: r 0.05, 47 = 0.288, r critical 0.288 < 0.566 (r observed ), so is
5R [Section 5.6 Health (2)]
t statistics for comparison of spionid infested &healthy abalone stocks.
Foot bleed data for clin. path.
Na/K ratio data arcsine transformed
Mean, SD and n values in Table 5.33
5S [Section 5.6 Health (2)]
Mann-Whitney U Test statistics for comparison of mud worm infested and healthy
abalone stocks.
Foot bleed data for clinical pathology
Mean, SD and n values in Table 5.33
FRDC Final Report Page129
5T [Section 5.6 Health (2)]
Haemocyte counts 2000. Three size classes and 2 year classes. 2 way ANOVASource of variation
Comparison of means for above ANOVA table. Haemocytes/mlSize
Means with shared superscripts are not significantly different (5% level)
5U [Section 5.6 Health (2)]
ANOVA 1999 Haemocyte data for 6 groups of abaloneSource of
variationSize/age group
5.116E+13 1.023E+13 2.43
2.193E+14 4.216E+12
Comparison of means for above ANOVA tableSize&age
< 1 year – big
Means with shared superscripts are not significantly different (5% level)
5V [Section 5.6 Health (2)]
Seawater profile from southern study sites (mmol.l-1)
FRDC Final Report Page130
5W [Section 5.6 Health (2)]
Monthly rainfall data for Dover weather station 1901-2000
FRDC Final Report Page131
5W Continued….
FRDC Final Report Page132
Source: http://www.imas.utas.edu.au/__data/assets/pdf_file/0020/743213/Development-of-an-Integrated-Management-Program-for-the-Control-of-Spionid-Mudworms-in-Abalone.PDF
Lack of bystander activation shows that localization exterior tochromosome territories is not sufficient to up-regulate gene expression Céline Morey, Clémence Kress and Wendy A. Bickmore 2009 19: 1184-1194 originally published online April 23, 2009 Genome Res. This article cites 34 articles, 19 of which can be accessed free at: Receive free email alerts when new articles cite this article - sign up in the box at the
Modelos de organización de fiestas Ponentes: Luisja Ugarte, Manu Alkiza, Jabi Allende e Idoia Ortíz de Moderador: Mikel Etxebarria 1. Luisja Ugarte. Ayuntamiento de 2. Manu Alkiza. Ayuntamiento de Getxo3. Jabi Allende. Ayuntamiento de Zalla4. Idoia Ortíz de Zárate. Ayuntamiento de 5. Debate sobre los modelos de organización Luisja Ugarte. Ayuntamiento de