98-0422
The Plant Cell, Vol. 11, 1195–1206, July 1999, www.plantcell.org 1999 American Society of Plant Physiologists
A Recessive Arabidopsis Mutant That Grows
Photoautotrophically under Salt Stress Shows Enhanced
Active Oxygen Detoxification
Kazuo Tsugane,a Kyoko Kobayashi,a Yasuo Niwa,a Yasushi Ohba,b Keishiro Wada,b
and Hirokazu Kobayashia,1
a Laboratory of Plant Cell Technology, Graduate School of Nutritional and Environmental Sciences, University of Shizuoka, 52-1 Yada, Shizuoka 422-8526, Japanb Department of Biology, Faculty of Science, Kanazawa University, Kakuma, Kanazawa 920-1192, Japan
Mutagenized Arabidopsis seedlings (ecotype Columbia) were screened for the ability to grow photoautotrophically on
solid medium containing 200 mM NaCl. A novel mutant line, designated pst1 (for photoautotrophic salt tolerance1), was
obtained. There were no significant differences between pst1 and wild-type plants with regard to their ability to induce
proline as an osmoregulatory solute. In addition, the content of monovalent cations in pst1 plants grown with or without
salt stress was equal to that in the wild type. We observed that light, even at moderate intensities, increased the effects
of salt stress on wild-type plants. The pst1 seedlings were nearly 10 times more tolerant to methyl viologen than were
wild-type seedlings. We also found that the activities of the active oxygen scavengers superoxide dismutase and ascor-
bate peroxidase were enhanced significantly in pst1 plants. The pst1 plants also were tolerant to other stresses, such
as high light intensity and toxic monovalent cations. The recessive nature of the pst1 mutation indicates that the poten-
tial for salt-stress tolerance is blocked in wild-type Arabidopsis.
Arid areas are expanding gradually but steadily on earth. It is
ample, osmolyte concentrations have been manipulated by
known that salt accumulation caused by unsuitable irriga-
constitutively expressing genes for a protective protein (Xu
tion practices decreases crop productivity (Epstein et al.,
et al., 1996) or genes for key enzymes involved in the pro-
1980). Under these conditions, high concentrations of NaCl
duction of osmosolytes occasionally associated with pro-
arrest plant development and lead to plant death. Several in-
tective activities (Tarczynski et al., 1993; Thomas et al.,
teracting events are triggered in plants by salt stress, includ-
1995; Hayashi et al., 1997; Karakas et al., 1997).
ing the inhibition of enzyme activities in metabolic pathways,
Photosynthesis, one of the most important metabolic
decreased uptake of nutrients in roots, decreased carbon-
pathways in plants, is a target of salt stress. Abscisic acid
use efficiency, and the denaturation of protein and mem-
produced in response to salt stress (Leung et al., 1994) de-
brane structures. However, the complete response of plants
creases turgor in guard cells and thus limits the CO2 avail-
to salt stress has not been explained systematically (Benzel
able for photosynthesis. During water stress, reduction of
and Reuveni, 1994).
chloroplast stromal volume (Gupta and Berkowitz, 1988)
Several mechanisms of defense and adaptation to water
and generation of active oxygen species (Price and Hendry,
deficiency resulting from salt stress have been well studied.
1991) also are thought to play important roles in inhibiting
Adaptation to water deficiency can involve the accumulation
of osmolytes, such as proline (Igarashi et al., 1997), glycine–
Analysis of salt-tolerant mutants may help researchers to
betaine, and sugar alcohols (Bohnert et al., 1995), or late-
elucidate the mechanisms of salt tolerance and to increase
embryo-abundant (Lea) proteins (Dure, 1993). With this
resistance to salt stress in sensitive species. Several Arabi-
knowledge, researchers have attempted to improve salt tol-
dopsis mutants with altered responses to salt have been re-
erance in plants by increasing levels of osmolytes. For ex-
ported, including mutants tolerant to salt during germination(Saleki et al., 1993; Werner and Finkelstein, 1995) and mu-tants that are salt hypersensitive (Wu et al., 1996). However,
no mutants have been reported whose photoautotrophic
To whom correspondence should be addressed. E-mail hirokazu@
u-shizuoka-ken.ac.jp; fax 81-54-264-5584.
growth is salt tolerant. To address the mechanisms of salt
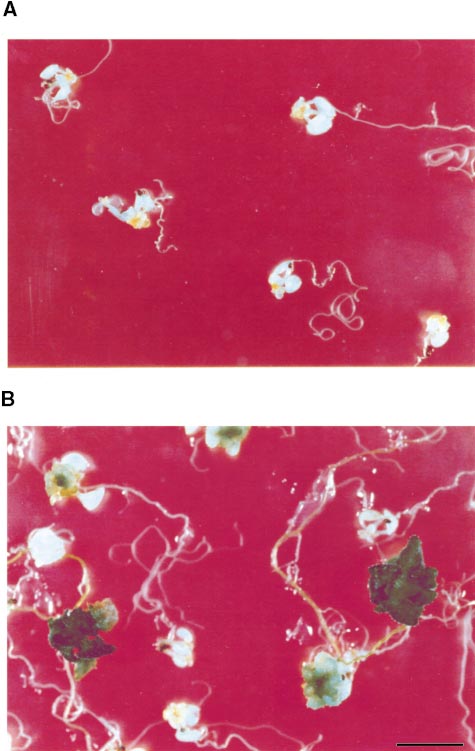
tolerance in photoautotrophic growth of plants and their ap-plication to plant improvement, we subjected Arabidopsisplants to mutagenesis, and we selected mutant seedlingssurviving under salt stress.
Selection of Mutants Capable of Photoautotrophic
Growth during Salt Stress
Instead of widely used media, such as Murashige and Skoog(MS) medium enriched with K1 (Murashige and Skoog,1962), a low-salt mineral medium containing essential ele-ments was used for screening mutants. M2 seedlings weregerminated on low-salt mineral medium and then trans-ferred to 200 mM NaCl in solid medium for 2 weeks. Underthese conditions, cotyledons of nonmutagenized plantscompletely bleached and died. By using this screen we havesucceeded in isolating two salt-tolerant mutant lines desig-nated pst (for photoautotrophic salt tolerance) from a total of148,300 plants from 22,300 lines mutagenized through ei-ther ethyl methanesulfonate treatment or T-DNA tagging(Table 1). Although two mutants designated pst1 and pst2were obtained, we focused on pst1 in this study.
Individuals showing the phenotype of pst1 were selected
from pst1 progeny for seven generations. However, not allprogeny of pst1 parents showed the phenotype of the pst1mutant. When grown photoautotrophically, these resistantplants survived for 2 weeks on medium containing 200 mMNaCl or for 1 month on medium containing 100 mM NaCl(Figures 1A and 1B). Nonmutagenized plants apparentlydied under the same conditions. Salt-tolerant and salt-sen-sitive plants bore the same percentage of salt-tolerant prog-
Figure 1. Seedlings Maintained on 100 mM NaCl Medium for 1
eny, indicating that the plants were homozygous at the pst1
locus. Survival rates of pst1 plants at NaCl concentrations
(A) Wild-type seedlings.
from 100 to 300 mM, at which all wild-type plants died, were
(B) pst1 seedlings.
nearly constant at 20% (Figure 2). Therefore, the penetrance
Seedlings were germinated and grown in the light for 7 days without
of pst1 phenotype is concluded to be incomplete at z20%.
salt stress and then transferred to NaCl-containing medium and
Most pst1 plants were unable to survive on 400 mM NaCl
maintained at a light intensity of 39 mmol m22 sec21. Under thesegrowth conditions, wild-type plants did not survive but pst1 mutants did.
medium (Figure 2). The salt tolerance of pst1 mutants was
Bar in (B) 5 1 cm for (A) and (B).
maintained throughout their development from seedlings to
Table 1. Selection of Arabidopsis pst Mutants
mature plants (data not shown). There were no significant
morphological differences between pst1 and wild-type lines
Screened Screened pst
when they were grown without stress (data not shown).
Ethyl methanesulfonate 17,400
Wassilewskija T-DNA tagginga
Mapping of the pst1 Locus
Two factors complicated genetic analysis of the pst1 locus:
a Made by Feldmann (1991) and supplied by the Nottingham Arabi-
(1) the phenotype of pst1 mutation exhibited the incomplete
dopsis Stock Centre.
penetrance; and (2) the mutant occurs in ecotype Columbia,
A Salt-Tolerant Arabidopsis Mutant
whose usual partner for crossing (ecotype Landsberg erecta)is less sensitive to salt stress than is ecotype Columbia. Wefound that salt sensitivity of ecotype RLD is similar to that ofecotype Columbia. Polymorphism of genetic markers be-tween RLD and Columbia allowed us to map the pst1 alleleby using the simple sequence length polymorphism method(Bell and Ecker, 1994) with 82 plants. The pst1 allele is lo-cated at the top of chromosome 3 at 6.71 6 3.28 centimor-gans from nga162 and 9.8 6 3.9 centimorgans fromAthCHIB. No other loci related to environmental stress havebeen found in this area. It is concluded that pst1 is a previ-ously undescribed locus.
Sensitivity to Salt during Germination
Both pst1 and nonmutant lines were sensitive to salt duringthe imbibition period of germination (Figure 3). In contrast,previously reported mutants were capable of germinatingunder salt stress but were sensitive to salt during vegetative
Figure 3. Inhibition Rate of Germination of Seeds on MS Medium
growth (Saleki et al., 1993; Werner and Finkelstein, 1995).
Containing 200 mM NaCl.
Although germination of pst1 and wild-type seeds was in-
Germinating seeds were counted after 10 days on the NaCl-contain-
hibited by salt, the seeds remained viable, germinating when
ing medium. Seeds incapable of germinating without salt stress
transferred to standard MS medium.
were excluded. The data are averages of three individual experi-ments.
Salt Tolerance in pst1 Is Not Due to
Osmosolyte Accumulation
1993), and it is known to play a role in adaptation of Arabi-dopsis to salt stress (Yoshiba et al., 1995). These observa-
Proline is thought to function as an osmoregulatory solute in
tions indicated that increased salt tolerance in pst1 plants
stress adaptation in many plants (Delauney and Verma,
might be due to increased levels of cytoplasmic proline. Inall plants cultured on MS medium for 3 weeks without saltstress, proline was undetectable. Subsequent exposure to200 mM NaCl for 5 days caused proline accumulation of upto 20 mmol g fresh weight21 in wild-type plants and up to 8
mmol g fresh weight21 in pst1 progeny (Figure 4). When pst1plants showing the salt-tolerant phenotype were analyzed,similar results were obtained (data not shown). Because theinduced levels of proline in the pst1 mutants are lower thanthose in the parental lines, proline accumulation does notconfer salt tolerance to the pst1 mutants. The reduced accu-mulation of proline in the pst1 mutants may indicate that pst1plants have gained a different mechanism of salt tolerance.
Accumulation of Na1 and Uptake of Other Cations
The cation content of pst1 plants before and after exposureto salt stress was determined. The Na1 content of both lines
Figure 2. Survival Rates of pst1 Plants at Increasing Concentrations
of NaCl in Mineral Medium.
increased 11.5 times in the presence of 200 mM NaCl (Fig-ure 5A). The concentration of K1 in wild-type and pst1 plants
Shown are the survival rates of pst1 plants (filled circles) at concen-
decreased under salt stress (Figure 5B). With or without salt
trations above which all wild-type plants (open circles) died. One-
stress, levels of Ca21 (Figure 5C) and Mg21 (Figure 5D) were
week-old seedlings were transferred to NaCl-containing medium
not significantly different between nonmutagenized and pst1
and maintained for periods during which all wild-type plants died.
The data are averages of the results obtained from three indepen-
mutant lines. MS medium containing 19.8 mM K1 (Murashige
dent Petri dishes.
and Skoog, 1962) slightly reduced salt stress compared with
months but did not grow. In contrast, the plants bleachedand died under the same conditions when the salt stresswas eliminated (data not shown).
The expression of members of the gene families of RBCS,
which codes for the small subunit of ribulose-1,5-bisphos-phate carboxylase/oxygenase (Rubisco), and CAB (alsoknown as LHCB), which codes for chlorophyll a/b bindingprotein, without salt stress increased and kept plateaus withelevating light intensities (Figures 7A and 7B). In contrast,RBCS or CAB expression in wild-type plants showed maxi-mal at z10 mmol m22 sec21 in the presence of 200 mM NaClbut diminished with increasing intensities of light (Figures 7Aand 7B). Therefore, salt stress was strengthened even bymoderate intensities of light. This indicates that salt stressmay be mediated by photosynthetic activity. However, saltstress interfered less with the expression of photosynthesisgenes in the mutant plants (Figures 7A and 7B).
Figure 4. Accumulation of Proline in Leaves of Plants Growing in
MS Medium Containing 200 mM NaCl.
Tolerance to Superoxide Radicals
Plants were cultured without salt stress for 3 weeks before exposureto NaCl. Contents of proline as a major osmoregulatory solute were
The apparent coupling of salt sensitivity with photosynthesis
determined after a 5-day incubation on medium containing 200 mMNaCl. The data presented are averages of the results obtained from
led us to speculate that salt may promote the generation of
three individual experiments.
active oxygen species through photosynthesis. The herbi-cide methyl viologen, commonly known as paraquat, gener-ates superoxide radicals during photosynthesis that causedamage to photosystems I and II (Dodge, 1994). The pst1line was more tolerant of methyl viologen than was the wild
mineral medium containing 7.5 mM K1 (data not shown).
type (Figures 8A and 8B). Plants that were sensitive to me-
Under all conditions tested, there were no significant differ-
thyl viologen began to bleach after 2 days of exposure to the
ences in K1, Na1, Ca21, and Mg21 contents and the ratio of
herbicide. The survival rate of pst1 plants treated with the
K1 to Na1 (Figure 5E) between wild-type and pst1 plants.
herbicide for 7 days was 10 times higher than that of non-
Therefore, the mechanism of salt tolerance in pst1 cannot
mutagenized plants (Figure 8C). The damage to plants
be explained by alteration of cation uptake ability.
caused by the herbicide also was evaluated by measuringelectrolyte leakage. Reduced electrolyte leakage from pst1plants exposed to methyl viologen (Figure 9) indicated thatthe pst1 line was more tolerant of the herbicide.
Salt Stress Is Increased by Moderate Light Intensities
We found that light intensity affected the sensitivity of wild-type seedlings to salt. Seedlings grown without stress were
Activity of Active Oxygen–Scavenging Enzymes
exposed to NaCl under different intensities of light. In theabsence of salt stress, light at moderate intensities (26 and
It is an intriguing question whether active oxygen species
39 mmol m22 sec21) did not inhibit seedling growth. In con-
are detoxified rapidly in pst1 mutants or whether the meta-
trast, moderate light caused severe damage to wild-type
bolic machinery in the mutants in some way tolerates active
plants grown under salt stress (Figure 6). Seedlings grown
oxygen. We determined the activities of superoxide dismu-
for 7 days with 200 mM NaCl and 26 or 39 mmol m22 sec21
tase (SOD), which catalyzes the conversion of superoxide to
light did not survive, whereas z90% of seedlings grown in
hydrogen peroxide, and of ascorbate peroxidase (APX),
13 mmol m22 sec21 light survived. Nearly 100% of salt-
which is involved in removal of hydrogen peroxide. When
stressed seedlings grown in low light (6.5 mmol m22 sec21)
plants were exposed to 200 mM NaCl for 5 days, the activity
or in the dark survived. The survival rates of wild-type and
of both enzymes was elevated in the pst1 line compared
pst1 plants were different at 26 mmol m22 sec21 or higher
with wild types (Figures 10A and 10B). These results indi-
light intensities; the mutant plants were more tolerant than
cate that salt stress enhances the production of active oxy-
were the wild-type plants (Figure 6). In the presence of 200
gen species and that active oxygen species detoxified more
mM NaCl, both wild-type and pst1 plants cultured in the
efficiently in the pst1 line, resulting in enhanced stress toler-
dark without sugar remained viable and green for at least 3
A Salt-Tolerant Arabidopsis Mutant
Tolerance of pst1 Mutants to High Light Intensity
The association of increased SOD and APX activity with salttolerance in pst1 led us to speculate that these mutants alsowould show increased tolerance to high-intensity light,which promotes active oxygen production. It is known thathigh-intensity light affects the chlorophyll fluorescenceemission from leaves. A simple fluorescence parameter Fv/Fm (Fv 5 Fm 2 FO; Fm, maximal fluorescence; FO, steadystate fluorescence) can be used routinely to estimatechanges in the quantum yield of noncyclic electron transport(Genty et al., 1989). As shown by Fv/Fm measurement, pho-tosystem II suffered less damage in pst1 mutants than inwild-type plants when exposed to high-intensity light (675mmol m22 sec21) for 3 hr (Figure 11).
Tolerance of pst1 Mutants to LiCl or CsCl
It is known that Cs1 enters through K1 influx channels inhigher plants (Very et al., 1995; Ichida et al., 1997) and thatNa1-hypersensitive mutants of yeast are highly sensitive toLi1 (Mendoza et al., 1994). Plants grown without salt stressfor 7 days were transferred to medium containing either 20mM LiCl or 10 mM CsCl. The wild-type plants were less via-ble on the 10 mM CsCl medium than were the pst1 plants(Figures 12A and 12B). Wild-type plants exposed to LiCl orCsCl for 12 days died, whereas z40% of pst1 plants grownunder the same conditions survived (Figures 12C and 12D).
After a 5-day exposure to Li1 or Cs1, the levels of Li1 or Cs1were similar in the wild-type and pst1 lines (Figure 13).
These results indicate that the capacity for cation uptake inpst1 mutants is not different from that of wild-type plants.
The near constant percentages of surviving pst1 plants be-tween NaCl concentrations of 100 and 300 mM (Figure 2) in-dicate the incomplete penetrance of the pst1 phenotype(Brown, 1992). The profile of survival rates as a function of
Plants were cultured without salt stress for 3 weeks before exposure
to NaCl, after which they were maintained for 5 days on media with-
out (left) or with (right) 200 mM NaCl. The data presented are means
of three individual experiments.
(A) Na1 leaf content.
(B) K1 leaf content.
(C) Ca21 leaf content.
Figure 5. Concentrations of Cations in Leaves of Plants on MS Me-
(D) Mg21 leaf content.
dium Containing 200 mM NaCl.
(E) Ratios of K1 to Na1.
tants are defective in high-affinity K1 uptake and in low-affinity Na1 uptake (Ding and Zhu, 1997). The pst1 mutantsaccumulated Cs1, which is known to enter through K1 influxchannels (Very et al., 1995; Ichida et al., 1997), to the samelevel as wild-type plants (Figure 13B). This observation con-firms that the activity of K1 influx channels is not enhancedin the pst1 mutants.
It has been shown that Ca21 is required to maintain or en-
hance the selective import of K1 into plants exposed to highconcentrations of external Na1 (Lahaye and Epstein, 1969).
Intercellular Ca21 signaling through a calcineurin-like path-way has been reported to play a crucial role in salt tolerance(Liu and Zhu, 1998). Furthermore, the Ca21-dependent pro-tein kinases CDPK1 and CDPK1a are involved in stress sig-
Figure 6. Influence of Moderate Light Intensities on Survival of
Plants Cultured in the Presence of 200 mM NaCl.
nal transduction (Sheen, 1996). Salt tolerance of pst1 doesnot appear to be related to changes in total intracellular con-
Seven-day-old seedlings were transferred to mineral medium con-
centrations of Ca21, because Ca21 content in pst1 and wild-
taining 200 mM NaCl and incubated under different intensities of
type plants was equivalent under salt stress (Figure 5C).
continuous white fluorescent light. The values next to the lines indi-cate light intensities in micromoles per square meter per second.
The open symbols and dotted lines are for wild-type plants, and theclosed symbols and solid lines are for pst1 plants.
salt concentrations (Figure 2) shows that the upper thresh-old is near 300 mM, above which osmotic pressure or Na1and Cl2 concentrations are high enough to kill the mutantplants. The incomplete penetrance could result from insuffi-cient activity of enzymes that detoxify active oxygen speciesfor conferring complete salt tolerance on all pst1 plants. Be-cause activities of SOD or APX in individual pst1 plants ineach generation that showed the salt-tolerant and wild-typephenotypes were nearly identical within standard deviationsof z10% (data not shown), we speculate that events thatcaused death were complex, possibly including interactionswith other factors.
When plants were exposed to salt stress, they accumu-
lated the osmosolyte proline (Figure 4). Rubisco, located inthe chloroplast stroma, is the most abundant protein onearth and constitutes up to 50% of total chloroplast protein(Ellis, 1979). It has been reported that the massive accumu-lation of amino acids or their derivatives under conditions ofwater depletion may be associated with increased proteinbreakdown (Nover et al., 1989; Callis, 1995). The expressionof the photosynthesis genes RBCS and CAB was stronglyinhibited by salt stress (Figures 7A and 7B). We speculatethat the reduction of RBCS expression during salt stressmay be necessary for terminating incorporation of amino
Figure 7. Effect of Moderate Light Intensities on Expression of
acids into proteins. Decreased protein synthesis and en-
RBCS and CAB in Leaves of Plants Grown on MS Medium Contain-
hanced protein degradation could yield the high con cen-
ing 200 mM NaCl.
trations of free amino acids needed to maintain osmotic
Three-week-old plants were transferred to the high-salt medium and
pressure in salt-stressed plants.
incubated for 5 days under different intensities of continuous white
Proton-coupled K1 transport generates osmotic pressure
fluorescent light. Transcript levels were normalized by using the 25S
in cells and in their intracellular compartments (Läuchi and
Bieleski, 1983). Increases in osmotic pressure may contrib-
(A) RBCS transcripts.
ute to salt tolerance. The sos1 (for salt–overly sensitive) mu-
(B) CAB transcripts.
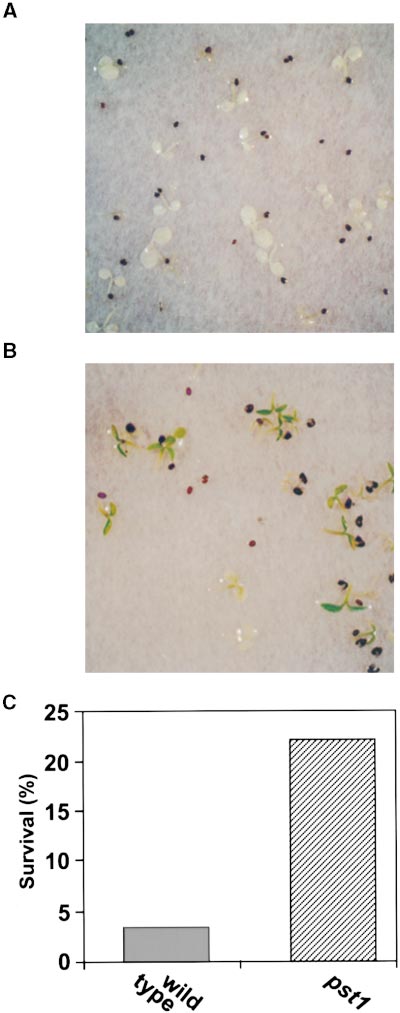
A Salt-Tolerant Arabidopsis Mutant
We found that light, even at moderate intensities, in-
creased salt stress (Figure 6). We also have observed thatsalt-stressed plants cultured without sugar in the dark re-main alive and green for at least 3 months but do not grow.
In contrast, plants bleach and die under the same conditionswhen salt stress is eliminated (data not shown). Changes inlight intensity have dramatic effects on the salt sensitivity. Itis possible that salt stress in the dark causes seedling dor-mancy. This response could result from a signal transduc-tion pathway activated by salt exposure in the dark, possiblyinvolving abscisic acid, which induces dormancy in plants.
The survival rates (Figure 6) and levels of transcripts for pho-tosynthesis genes (Figures 7A and 7B) were significantlyhigher in pst1 plants than they were in wild-type plants un-der salt stress and increasing intensities of light, leading tothe speculation that pst1 plants are more tolerant to activeoxygen species, which are generated under higher intensi-ties of light.
pst1 seedlings were more tolerant than wild-type plants to
methyl viologen and high light intensity as well as Li1 andCs1. Active oxygen species reportedly have been generatedby drought (Elstner, 1991), herbicide treatment (Asada,1994), and cold stress (Malan et al., 1990). Increases in bothSOD and APX activities in pst1 mutants support the sugges-tion that the pst1 mutation increases active oxygen–scav-enging activity and thus confers tolerance to generalenvironmental stresses. The Cu/Zn–SOD in chloroplasts isthe major SOD detected in plant leaves (Asada et al., 1973).
Ascorbate peroxidase, which catalyzes the conversion of
Figure 8. Sensitivity of Plants to Superoxide Radicals Generated
with Methyl Viologen.
Superoxide radicals were generated by treatment with 30 mM methylviologen for 7 days under moderate light intensity (29 mmol m22sec21). The seedlings were treated on filter paper soaked in methyl
Figure 9. Electrolyte Leakage from Seedlings Treated with Methyl
viologen dissolved in distilled water. Seedling survival rates are
means of three individual experiments.
Plants were soaked in 10 nM methyl viologen solution in 24-well
(A) Wild type.
plates (Stem, Tokyo, Japan) under moderate light intensity (29 mmol
m22 sec21). Leakage values were calculated from slopes maintained
(C) Survival rate.
over 16 hr. The data presented are means of three individual experi-ments. S, sec; wt, weight.
single mutation, activities of at least two enzymes for detox-ifying active oxygen species were enhanced in this mutant.
Thus, the PST1 gene may regulate the expression of a seriesof genes involved in active oxygen detoxification.
Plant Growth
Seeds (Arabidopsis thaliana ecotype Columbia) were surface-steril-ized in 70% ethanol for 1 min and then in 5% NaCl containing 0.05%Tween 20 for 10 min, followed by five washes with sterile distilled wa-ter. The seeds were sown in plastic Petri dishes containing solidifiedgellan gum (0.2% [w/v]; Kelco, San Diego, CA) with either low-saltmineral medium (5 mM KNO3, 2.5 mM KH2PO4, 2 mM MgSO4, 2 mMCa[NO3]24H2O, 50 mM Fe–EDTA, 70 mM H3BO4, 14 mM MnCl2, 0.5mM CuSO4, 1 mM ZnSO4, 0.2 mM Na2Mo4, 10 mM NaCl, and 0.01 mMCoCl2) for screening of mutants or Murashige and Skoog (MS) me-dium (Murashige and Skoog, 1962) for synchronized growth of seed-lings. Unless otherwise noted, plants were grown axenically at 228Cfor 7 days under moderate intensity (26 mmol m22 sec21) of continu-ous white fluorescent light. Seedlings were then transferred to newmedium of the same composition, treated with NaCl or otherstresses, and kept under the same growth conditions for the indi-cated periods.
Figure 10. Activities of SOD and APX in Leaves of Plants Main-
tained on 200 mM NaCl MS Medium.
Plants were grown for 3 weeks without salt stress before exposure to
NaCl for 3 days. The data are averages of three individual experiments.
(A) SOD.
(B) APX.
H2O2 to H2O, also is found in chloroplasts (Miyake and Asada,1992). With or without salt stress, the activities of SOD andAPX in pst1 were z1.3 and 3 times the activities of the wildtype, respectively (Figures 10A and 10B). A 30 to 100% ele-vation of SOD activity in transgenic tobacco and alfalfa hasbeen reported to confer tolerance to oxidative stress (Guptaet al., 1993; Van Camp et al., 1994) and freezing stress
Figure 11. Effect of High Light Intensity on Photosystem II in
(McKersie et al., 1993). Therefore, the detected increase of
SOD and APX activities in pst1 plants may play an importantrole in the enhanced tolerance of the mutants to salt and
Plants were cultured for 4 weeks without salt stress. Experimentswere conducted under high light intensity (675 mmol m22 sec21) at
other stresses.
208C for 3 hr. Remaining photosystem II (PS II) activities were esti-
The recessive nature of the pst1 mutation indicates that
mated by measuring chlorophyll fluorescence immediately after ex-
the potential for salt tolerance is blocked in wild-type Arabi-
posure to irradiance. Leaves exhibited Fv/Fm values between 0.75
dopsis. Therefore, land plants may have suppressed but not
and 0.85, which are similar to values reported for photosystem II in
lost the capacity for salt tolerance during their evolution
unstressed plants (Genty et al., 1989). The ordinate indicates Fv/Fm
from ancestral organisms exposed to salt stress or more se-
values normalized to those of untreated leaves (average Fv/Fm 5
vere oxidative stress. Although plants in the pst1 line have a
0.8). The data are averages of three individual experiments.
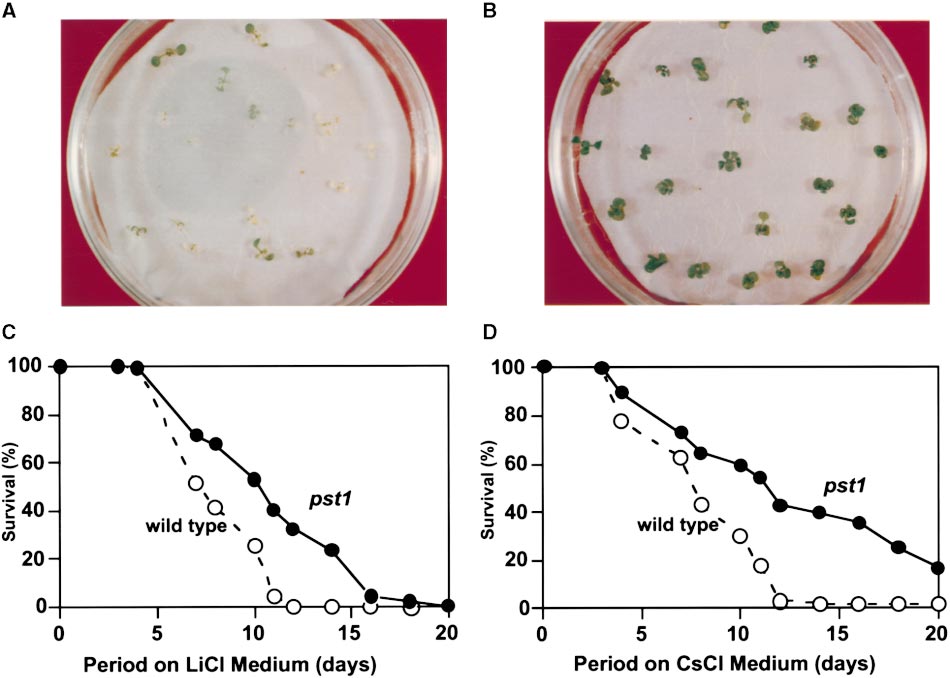
A Salt-Tolerant Arabidopsis Mutant
Figure 12. Influence of LiCl and CsCl on Survival of Seedlings.
(A) and (B) Sensitivity of wild-type (A) and pst1 (B) plants grown on MS medium containing 10 mM CsCl for 7 days under light at 19.5 mmol m22
sec21 is shown.
(C) and (D) Seven-day-old seedlings were transferred to MS medium containing 20 mM LiCl (C) or 10 mM CsCl (D). Seedlings were incubated at
a light intensity of 39 mmol m22 sec21. The survival rates presented are means of three individual experiments.
To map the photoautotrophic salt tolerance pst1 locus, the pst1 mu-
Cation contents were determined by atomic absorption spectrome-
tant line was crossed with ecotype RLD, and the F2 progeny were
try. Harvested leaves were washed with distilled water and dried at
screened for salt tolerance. Using standard methods (Bell and Ecker,
608C for 7 days in 1.5-mL tubes. Samples then were weighed and
1994), we found the pst1 locus to be linked to the simple sequence
ashed at 5008C overnight in a muffle furnace (Electric Muffle Furnace
length polymorphism marker nga6 on chromosome 3. Primers for
KL-160; Advantec Toyo, Tokyo, Japan). The ash was solubilized by
this analysis were purchased from Research Genetics (Huntsville,
heating in 1 M HCl. Cation contents were determined by using a po-
AL). Map distances were calculated as described by Koornneef and
larized Zeeman atomic absorption spectrophotometer Z-8000 (Hita-
Stam (1992).
chi, Ltd., Hitachi, Japan).
Determination of Transcript Levels
Determination of Proline and Protein Concentrations
RNA was isolated from leaves by using Isogen (Wako, Tokyo, Japan)
Proline was extracted from leaves, and its concentration was deter-
according to the manufacturer's instructions. RNA gel blot analysis
mined by the method reported by Sanada et al. (1995). Protein con-
was performed following Sambrook et al. (1989). Probes used for hy-
centrations were determined using a BCA (bicinchoninic acid)
bridization were a 393-bp NcoI-NsiI fragment of RBSC-3B (Krebbers
protein assay kit (Pierce Chemical Co., Rockford, IL), according to
et al., 1988) from expressed sequence tag clone 36G8T7, supplied
the manufacturer's instructions.
by the Arabidopsis Biological Resource Center (Columbus, OH); a
bleached. Seedlings also were exposed to 10 nM methyl viologen formeasuring conductivity to evaluate electrolyte leakage. The conduc-tivity of the ambient solution was determined using a twin conductiv-ity meter B-173 (Horiba, Kyoto, Japan).
Determination of Superoxide Dismutase and Ascorbate
Peroxidase Activities
Plant leaves were homogenized in 0.1 M Na2HPO4/NaH2PO4, pH7.0, at 48C and centrifuged at 4000g for 15 min at 48C. The super-natant was filtered through filter paper (No. 1; Whatman) and sub-jected to gel filtration in Cellulofine GH-25-m (Chisso, Osaka, Japan)preequilibrated with 50 mM Na2CO3/NaHCO3, pH 10.2 (Spychallaand Desborough, 1990). Superoxide dismutase (SOD) activity wasdetermined using an SOD-525 assay kit (OXIS International, Inc.,Portland, OR) according to the manufacturer's instructions. The as-say is based on the catalytic conversion of SOD to autoxidate5.6.6a,11b-tetrahydroxybenzo(C) fluorene in alkaline solution and ismonitored by measurement of absorbance of the resultant chro-mophore at 525 nm.
Ascorbate peroxidase (APX) was assayed as described previously
by Chen and Asada (1990). The reaction mixture contained 50 mMK–phosphate, pH 7.0, 0.5 mM hydrogen peroxide, and 1 mM ascor-bate. One and one-half to 11.5 sec after initiating the reaction by ad-dition of hydrogen peroxide, we measured the activity of APX by thedecrease in absorbance at 290 nm (absorbance coefficient for ascor-bate of 2.8 mM21 cm21).
Chlorophyll fluorescence from leaves was determined by using mon-itoring system MFMS II (Hansatech, King's Lynn, UK). Detachedleaves were placed on solid mineral medium in the assay chamberand exposed to high intensity light (675 mmol m22 sec21) at 208C for
Figure 13. Concentrations of Li1 and Cs1 in Plant Leaves.
3 hr before measurement. Leaves did not become dry over this pe-
(A) Plants grown in 20 mM LiCl for 5 days under light at 39 mmol m22
sec21.
(B) Plants grown in 10 mM CsCl for 5 days under light at 39 mmol
m22 sec21.
Plants were grown for 3 weeks before transfer to MS medium con-taining LiCl or CsCl. The data presented are means of three individ-ual experiments.
We thank Kozi Asada and Junichi Mano for their invaluable com-ments on the active oxygen detoxification system, Yasuyuki Hayashifor his advice on measurement of conductivity, Mari Saeki-Narusakafor her technical assistance during the early stages of the research,Enno Krebbers for his comments on the designation pst, Sachiko
592-bp HindIII-SacI fragment of CAB1 (Leutwiler et al., 1986) of DNA
Takase and Toshinao Goda for use of the muffle furnace, and
amplified by polymerase chain reaction with genomic DNA of Arabi-
Kimitaka Yakura for the VER18 clone. K.T. is a recipient of a research
dopsis ecotype Columbia; and a 3.7-kb fragment for 25S rRNA from
fellowship for young scientists from the Japan Society for the Pro-
the clone VER18 harboring DNA from Vicia faba (Yakura and Tanifuji,
motion of Science (JSPS). The research was supported by Grants-
in-Aid from the Ministry of Education, Science, and Culture of Japan(Monbusho) and by grants from the New Energy and Industrial Tech-nology Development Organization (NEDO), the Research Institute of
Treatment with Methyl Viologen and Measurement of
Innovative Technology for the Earth (RITE), and the Toray Science
Seedlings grown on filter paper wetted with distilled water weresoaked in 30 mM methyl viologen and incubated until plants became
Received November 9, 1998; accepted April 8, 1999.
A Salt-Tolerant Arabidopsis Mutant
plants that overexpress chloroplastic Cu/Zn superoxide dismu-
tase. Proc. Natl. Acad. Sci. USA 90, 1629–1633.
Hayashi, H., Mustardy, L., Deshnium, P., Ida, M., and Murata, N.
Asada, K. (1994). Production and action of active oxygen species in
(1997). Transformation of Arabidopsis thaliana with the codA gene
photosynthetic tissues. In Causes of Photooxidative Stress and
for choline oxidase: Accumulation of glycinebetaine and
Amelioration of Defense Systems in Plants, C.H. Foyer and P.M.
enhanced tolerance to salt and cold stress. Plant J. 12, 133–142.
Mullineaux, eds (Boca Raton, FL: CRC Press), pp. 77–104.
Asada, K., Urano, M., and Takahashi, M. (1973). Subcellular loca-
Ichida, A.M., Pei, Z.-M., Baizabal-Aguirre, V.M., Turner, K.J., and
tion of superoxide dismutase in spinach leaves and preparation
Schroeder, J.I. (1997). Expression of a Cs1-resistant guard cell
and properties of crystalline spinach superoxide dismutase. Eur.
K1 channel confers Cs1-resistant, light-induced stomatal opening
J. Biochem. 36, 257–266.
in transgenic Arabidopsis. Plant Cell 9, 1843–1857.
Bell, C.J., and Ecker, J.R. (1994). Assignment of 30 microsatellite
Igarashi, Y., Yoshiba, Y., Sanada, Y., Yamaguchi-Shinozaki, K.,
loci to the linkage map of Arabidopsis. Genomics 19, 137–144.
Wada, K., and Shinozaki, K. (1997). Characterization of the gene
for
D1-pyrroline-5-carboxylate synthetase and correlation
Benzel, M.L., and Reuveni, M. (1994). Cellular mechanisms of salt
between the expression of the gene and salt tolerance in Oryza
tolerance in plant cells. Hortic. Rev. 16, 33–69.
sativa L. Plant Mol. Biol. 33, 857–865.
Bohnert, H.J., Nelson, D.E., and Jensen, R.G. (1995). Adaptations
to environmental stresses. Plant Cell 7, 1099–1111.
Karakas, B., Ozias-Akins, P., Stushnoff, C., Suefferheld, M., and
Rieger, M. (1997). Salinity and drought tolerance of mannitol-
Brown, T.A. (1992). Genetics, 2nd ed. (London: Chapman and Hall).
accumulating transgenic tobacco. Plant Cell Environ. 20, 609–616.
Callis, J. (1995). Regulation of protein degradation. Plant Cell 7,
Koornneef, M., and Stam, P. (1992). Genetic analysis. In Methods
in Arabidopsis Research, C. Koncz, N.-H. Chua, and J. Schell, eds
Chen, G.X., and Asada, K. (1990). Hydroxyurea and p-aminophenol
(Singapore: World Scientific), pp. 83–99.
are the suicide inhibitors of ascorbate peroxidase. J. Biol. Chem.
Krebbers, E., Seurinck, J., Herdies, L., Cashmore, A.R., and
Timko, M.P. (1988). Four genes in two diverged subfamilies
Delauney, A.J., and Verma, D.P. (1993). Proline biosynthesis and
encode the ribulose-1,5-bisphosphate carboxylase small subunit
osmoregulation in plants. Plant J. 4, 215–223.
polypeptides of Arabidopsis thaliana. Plant Mol. Biol. 11, 745–759.
Ding, L., and Zhu, J.-k. (1997). Reduced Na1 uptake in the NaCl-
Lahaye, P.A., and Epstein, E. (1969). Salt toleration by plants:
hypersensitive sos1 mutant of Arabidopsis thaliana. Plant Physiol.
Enhancement with calcium. Science 166, 395–396.
Läuchi, A., and Bieleski, R.L., eds (1983). Inorganic Plant Nutrition.
Dodge, A.D. (1994). Herbicide action and effects on detoxification
processes. In Causes of Photooxidative Stress and Ameliorationof Defense Systems in Plants, C.H. Foyer and P.M. Mullineaux,
Leung, J., Bouvier-Durand, M., Morris, P.C., Guerrier, D., Chefdor,
eds (Boca Raton, FL: CRC Press), pp. 219–236.
F., and Giraudat, J. (1994). Arabidopsis ABA response gene
ABI1: Features of a calcium-modulated protein phosphatase. Sci-
Dure III, L. (1993). Structural motifs in Lea proteins. In Plant
ence 264, 1448–1452.
Responses to Cellular Dehydration during Environmental Stress,T.J. Close and E.A. Bray, eds (Rockville, MD: American Society of
Leutwiler, L.S., Meyerowitz, E.M., and Tobin, E.M. (1986). Struc-
Plant Physiologists), pp. 91–104.
ture and expression of three light-harvesting chlorophyll a/b–bind-
Ellis, R.J. (1979). The most abundant protein in the world. Trends
ing protein genes in Arabidopsis thaliana. Nucleic Acids Res. 14,
Biochem. Sci. 4, 241–244.
Elstner, E.F. (1991). Mechanisms of oxygen activation in different
Liu, J.P., and Zhu, J.-k. (1998). A calcium sensor homolog required
compartments of plant cells. In Active Oxygen/Oxidative Stress
for plant salt tolerance. Science 280, 1943–1945.
and Plant Metabolism, E.J. Pelland and K.L. Steffen, eds (Rock-
Malan, C., Greyling, M.M., and Gressel, J. (1990). Correlation
ville, MD: American Society of Plant Physiologists), pp. 13–25.
between CuZn superoxide dismutase and glutathione reductase,
Epstein, E., Norlyn, J.D., Rush, D.W., Kingsbury, R.W., Kelley,
and environmental and xenobiotic stress tolerance in maize
D.B., Cunningham, G.A., and Wrona, A.F. (1980). Saline culture
inbreds. Plant Sci. 69, 157–166.
of crops: A genetic approach. Science 210, 399–404.
McKersie, B.D., Chen, Y., Beus, M.D., Bowley, S.R., and Bowler,
Feldmann, K.A. (1991). T-DNA insertion mutagenesis in Arabidop-
C. (1993). Superoxide dismutase enhances tolerance of freezing
sis: Mutational spectrum. Plant J. 1, 71–82.
stress in transgenic alfalfa (Medicago sativa L.). Plant Physiol.
Genty, B., Briantais, J.M., and Baker, N.R. (1989). The relationship
between the quantum yield of photosynthetic electron transport
Mendoza, I., Rubio, F., Rodriguez-Navarro, A., and Pardo, J.M.
and quenching of chlorophyll fluorescence. Biochim. Biophys.
(1994). The protein phosphatase calcineurin is essential for NaCl
Acta 990, 87–92.
tolerance of Saccharomyces cerevisiae. J. Biol. Chem. 269, 8792–
Gupta, A.S., and Berkowitz, G.A. (1988). Chloroplast osmotic
adjustment and water stress effects on photosynthesis. Plant
Miyake, C., and Asada, K. (1992). Thylakoid-bound ascorbate per-
Physiol. 88, 200–206.
oxidase in spinach chloroplasts and photoreduction of its primary
Gupta, A.S., Heinen, J.L., Holaday, A.S., Burke, J.J., and Allen,
oxidation product monodehydroascorbate radicals in thylakoids.
R.D. (1993). Increased resistance to oxidative stress in transgenic
Plant Cell Physiol. 33, 541–553.
Murashige, T., and Skoog, F. (1962). A revised medium for rapid
Thomas, J.C., Sephahi, M., Arendall, B., and Bohnert, H.J. (1995).
growth and bioassays with tobacco tissue cultures. Physiol. Plant.
Enhancement of seed germination in high salinity by engineering
mannitol expression in Arabidopsis thaliana. Plant Cell Environ.
Nover, L., Neumann, D., and Scharf, K.D. (1989). Heat shock and
other stress response systems of plants. In Results and Problems
Van Camp, W., Willekens, H., Bowler, C., Van Montagu, M., Inzé,
in Cell Differentiation, W.H. Nijmegen, L.N. Halle, and U.S.
D., Reupold-Popp, P., Sandermann, H., Jr., and Langebartels,
Würzburg, eds (Berlin: Springer-Verlag), pp. 78–105.
C. (1994). Elevated levels of superoxide dismutase protect trans-
Price, A.H., and Hendry, G.A.F. (1991). Iron-catalyzed oxygen radi-
genic plants against ozone damage. Bio/Technology 12, 165–168.
cal formation and its possible contribution to drought damage in nine
Very, A.A., Gaymard, F., Bosseux, C., Sentenac, H., and Thibaud,
native grasses and three cereals. Plant Cell Environ. 14, 477–484.
J.B. (1995). Expression of a cloned plant K1 channel in Xenopus
Saleki, R., Young, P.G., and Lefebvre, D.D. (1993). Mutants of Ara-
oocytes: Analysis of macroscopic currents. Plant J. 7, 321–332.
bidopsis thaliana capable of germination under saline conditions.
Werner, J.E., and Finkelstein, R.R. (1995). Arabidopsis mutants
Plant Physiol. 101, 839–845.
with reduced response to NaCl and osmotic stress. Physiol. Plant.
Sambrook, J., Fritsch, E.F., and Maniatis, T. (1989). Molecular
Cloning: A Laboratory Manual, 2nd ed. (Cold Spring Harbor, NY:
Wu, S.-J., Ding, L., and Zhu, J.-k. (1996). SOS1, a genetic locus
Cold Spring Harbor Laboratory Press).
essential for salt tolerance and potassium acquisition. Plant Cell
Sanada, Y., Ueda, H., Kuribayashi, K., Andoh, T., Hayashi, F.,
Tamai, N., and Wada, K. (1995). Novel light–dark change of pro-
Xu, D., Duan, X., Wang, B., Hong, B., Ho, T.-H.D., and Wu, R.
line levels in halophyte (Mesembryanthemum crystallinum L.) and
(1996). Expression of a late embryogenesis abundant protein
glycophyte (Hordeum vulgare L. and Triticum aestivum L.) leaves
gene, HVA1, from barley confers tolerance to water deficit and
and roots under salt stress. Plant Cell Physiol. 36, 965–970.
salt stress in transgenic rice. Plant Physiol. 110, 249–257.
Sheen, J. (1996). Ca21-dependent protein kinases and stress signal
Yakura, K., and Tanifuji, S. (1983). Molecular cloning and restric-
transduction in plants. Science 274, 1900–1902.
tion analysis of EcoRI-fragments of Vicia faba rDNA. Plant Cell
Spychalla, J.P., and Desborough, S.L. (1990). Superoxide dismu-
Physiol. 24, 1327–1330.
tase, catalase, and a-tocopherol content of stored potato tubers.
Yoshiba, Y., Kiyosue, T., Katagiri, T., Ueda, H., Mizoguchi, T.,
Plant Physiol. 94, 1214–1218.
Yamaguchi-Shinozaki, K., Wada, K., Harada, Y., and Shinozaki,
Tarczynski, M.C., Jensen, R.G., and Bohnert, H.J. (1993). Stress
K. (1995). Correlation between the induction of a gene for D1-pyr-
protection of transgenic tobacco by production of osmolyte man-
roline-5-carboxylate synthetase and the accumulation of proline
nitol. Science 259, 508–510.
in Arabidopsis thaliana under osmotic stress. Plant J. 7, 751–760.
Source: http://sfns.u-shizuoka-ken.ac.jp/pctech/pdf/pl-cell-tsugane.pdf
ANNEX 1. TERMS OF REFERENCE SURVEY ON AVAILABILITY OF MODERN CONTRACEPTIVES AND ESSENTIAL LIFE SAVING MATERNAL/REPRODUCITVE (RH) HEALTH MEDICINES IN SERVICE DELIVERY POINTS IN MONGOLIA I. INTRODUCTION AND OBJECTIVE The Reproductive Health Commodity Security project has been implemented in Mongolia since 2008 under
CC Malo.QXP 26/8/10 13:37 Página 84 Rehabilitación total fija implantosoportada en un paciente con ablación del maxilar,utilizando el concepto de rehabilitación All-on-4 extra-maxila 84 MAXILLARIS, septiembre 2010 CC Malo.QXP 26/8/10 13:37 Página 85 Ciencia y práctica Este artículo tiene como objetivo describir un caso clínico de reduce así la necesidad de trasplantes óseos y permite elrehabilitación fija implantosoportada bimaxilar en un pacien- anclaje en hueso de mejor calidad (zonas anteriores maxila-te con ausencia de maxilar y destrucción severa mandibular, res), posibilitando la utilización de implantes más largos y dis-a través de la técnica de All-on-4 extra-maxila y All-on-4 stan- minuyendo el tamaño del cantilever protésico3,4.dard mandibular.