Anthropology.ua.edu2
YEARBOOK OF PHYSICAL ANTHROPOLOGY 36:153—178 (1993)
G6PD Deficiency as Protection Against
falciparumMalaria: An Epidemiologic Critique of Population andExperimental Studies
LAWRENCE S. GREENE
Department of Anthropology and the Biology of Human Populations
Program, University of Massachusetts, Boston 02125, and Boston Sickle
Cell Center and the Department of Pediatrics, Boston City Hospital,
Boston, Massachusetts 02118
KEY WORDS Glucose-6-phosphate dehydrogenase deficiency, Malaria, Ep-
idemiology, Natural selection
ABSTRACT Several recent reviews in the medical literature maintain that
only heterozygous G6PD deficient females are relatively protected against
falciparum malaria. However, a number of population studies pro-vide
compelling evidence that both the hemizygous G6PD deficient male and
homozygous G6PD deficient female are also relatively protected against
falciparum parasitization. An epidemiologic critique of a sample of these
field investigations points out the methodological problems that un-derlie
some of the negative findings. In vitro studies also provide compelling
evidence that erythrocytes from
all G6PD deficient genotypes are relatively
protected against
falciparum infestation, and that this protection is en-
hanced by oxidant substances derived from a number of food crops such asfava beans.
It is suggested that "quinine" taste sensitivity reflects taste sensitivity to
bitter-tasting, naturally occurring antimalarial substances of plant origin,
and that the G6PD polymorphism and the genetic loci coding for "quinine"
taste sensitivity have co-evolved in human populations. It appears that
adaptation at the G6PD locus in human populations reflects an intricate
web of interactions between a large number of different G6PD deficient
alleles which have reached polymorphic frequencies and a variety of food
crops from which oxidant substances may be derived.
1993 Wiley-Liss, Inc.
The specific goal of this article is to review the evidence indicating that
all
glucose-6-phosphate dehydrogenase (G6PD) deficient genotypes are relatively pro-
tected against
Plasmodium falciparum malaria. A discussion of this issue is timely
and necessary because several influential reviews of G6PD and G6PD deficiency in
the medical literature over the past few years have reiterated the position that only
G6PD deficient
heterozygotes are protected against
falciparum parasitization, while
G6PD deficient hemizygotes and homozygotes are not (Luzzatto and Mehta, 1989;
Luzzatto and Battistuzzi, 1985; Usanga and Luzzatto, 1985). However, this
position is based on a single study (Bienzle et al., 1972), while a number of other
field investigations support the view that all G6PD deficient genotypes are rela-
tively protected against
falciparum parasitization (Allison and Clyde, 1961; Gilles
et al., 1967; Butler, 1973; Kar et al., 1992). The inaccuracy of the "heterozygote"
position seriously obscures our understanding of how selection operates at the
1993 Wiley-Liss, Inc.
YEARBOOK OF PHYSICAL ANTHROPOLOGY
[Vol.
36, 1993
G6PD locus and clouds any discussion of the relative fitnesses of the various G6PD
genotypes in areas where
falciparum malaria is endemic.
The first portion of the article will review evidence from population studies that
have evaluated the relative protection provided by the various G6PD genotypes
against parasitization with
Plasmodium falciparum. The data from these investi-
gations are conflicting; therefore, this review will present an in-depth evaluation,
from an epidemiologic perspective, of the designs of the various studies in order to
elucidate their relative strengths and weaknesses. A detailed epidemiologic cri-
tique of these population studies also provides a useful guide to future investiga-
tors with respect to a number of important issues encountered in such biomedical
population studies—whether the investigations are concerned with G6PD defi-
ciency or with other topics.
The second portion of the article reviews in vitro studies evaluating the relative
protection provided G6PD deficient erythrocytes against
falciparum parasitiza-tion.
These investigations are also reviewed in some detail so as to provide the reader
with a sense of the process of epidemiologic enquiry, rather than merely presenting
a summary of findings that are frequently somewhat equivocal.
The third goal of this article is to reiterate and expand a biocultural perspective
developed by Katz and others (Katz and Schall, 1979, 1986; Etkin, 1979; Golenser et
al., 1983, 1988). It stresses the point that adaptation at the G6PD locus involves an
interaction between genetic adaptation and cultural adaptation mediated through
dietary consumption of foods containing naturally occurring antimalarial
substances of plant origin. This viewpoint maintains that we can only come to an
appreciation of the web of interacting selective forces that underlie population
adaptation at the G6PD locus through an understanding of how cultural adapta-
tion acts upon and modifies biological adaptation. This section presents a brief
discussion of an hypothesis that there has been co-evolution at the G6PD locus and
the genetic locus coding for "quinine" taste sensitivity and some preliminary data in
support of this suggestion.
Again, the article focuses on a single narrow issue. That issue is whether the
epidemiologic and experimental evidence indicates that
all G6PD deficient geno-
types are relatively protected against
falciparum parasitization. Considerable at-
tention is paid to epidemiologic detail in order to provide a fuller picture of the
process of enquiry and to indicate some of the pitfalls encountered in such inves-
tigations. However, this article is
not a comprehensive review of the selective factors
influencing the G6PD polymorphism. Such a review will be presented elsewhere (LS
Greene, in preparation).
The role of glucose-6-phosphate dehydrogenase in the metabolism
of the erythrocyte
G6PD is a "housekeeping" enzyme that performs vital functions within all cells of
the body. However, within the erythrocyte, which lacks a nucleus, mitochondria, and
other organelles, there are certain constraints on metabolism and this enzyme has a
particularly important role. G6PD catalyzes the first step of the pentose phosphate
pathway (hexose monophosphate pathway), a series of side reactions off the main
glycolytic pathway in the erythrocyte and in all cells of the body. G6PD initiates
this pathway by catalyzing the oxidation of glucose-6-phosphate to 6-
phosphogluconolactone by the co-enzyme nicotinamide-adenine dinucleotide
phosphate (NADP ), which is reduced to NADPH. The 6-phosphogluconolactone
hydrolyzes spontaneously to 6-phosphogluconate. This serves as a substrate for 6-
phosphogluconate dehydrogenase and NADP . The second enzymatic step in this
pathway is also associated with the reduction of NADP+ to NADPH. The NADPH
produced as a consequence of these reactions reduces oxidized glutathione (GSSG)
to reduced glutathione (GSH) in a reaction catalyzed by glutathione re-ductase.
GSH then reduces hydrogen peroxide, a powerful oxidant produced in the course of
cellular metabolism and as a consequence of the inflammatory response,
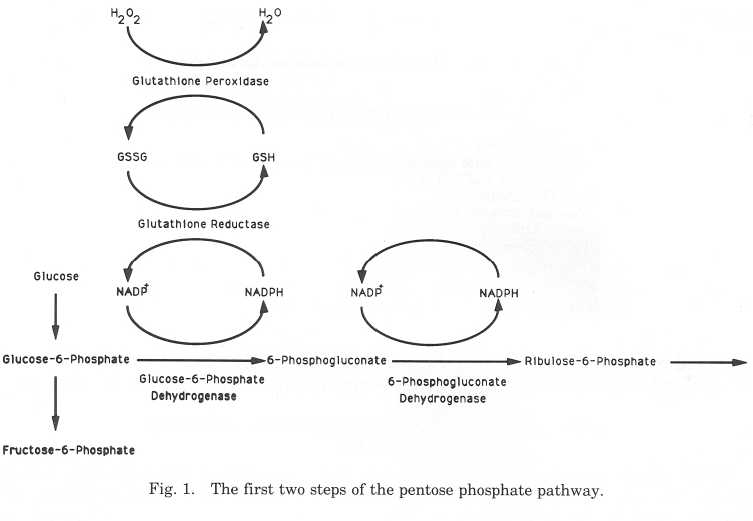
G6PD DEFICIENCY AND FALCIPARUM MALARIA
and other endogenous and exogenous oxidants, in a reaction catalyzed by glu-
tathione peroxidase (Newsholme and Leech, 1983; Beutler, 1983; WHO Working
Group, 1989; Luzzatto and Mehta, 1989). The first part of the pentose phosphate
pathway is outlined in Figure 1.
The main function of the pentose phosphate pathway is the generation of reduc-
ing capacity through the production of NADPH and ultimately GSH. This is the
only mechanism available to the erythrocyte for generating reducing capacity and
is thus essential for cell survival, while in other cells of the body alternative means of
NADPH production exist and the pentose phosphate pathway accounts for only
60% of NADPH production (Newsholme and Leech, 1983; Beutler, 1983; WHO
Working Group, 1989; Luzzatto and Mehta, 1989).
GSH generated through the pentose phosphate pathway, as outlined above, is
the only defense against oxidant stress in the red blood cell (Friedman, 1979; WHO
Working Group, 1989). In the unstressed G6PD normal erythrocyte, G6PD activity is
only about 2% of total capacity (WHO Working Group, 1989). This is increased
greatly to meet the challenge of an oxidant stress and GSH is maintained at stable
levels. However, the G6PD deficient erythrocyte has greatly reduced G6PD activity
(10 to 20% of normal in G6PD A (—) and 0 to 10% of normal in G6PD Medi-
terranean and many similar variants) (Luzzatto and Mehta, 1989). An increased
oxidant stress can lead to a marked depletion of GSH as the ability of the deficient
G6PD to generate NADPH is exceeded by a high rate of GSH loss (Beutler, 1983;
Eckman and Eaton, 1979).
Uncompensated oxidant stress in the normal erythrocyte (or more easily in the
G6PD deficient erythrocyte) results in the oxidation of hemoglobin to methemo-
globin, Heinz body formation, and membrane damage (Beutler, 1983). If extreme
this will result in hemolysis, while a less severe but uncompensated oxidant stress
will decrease the distortability of the erythrocyte and increase the likelihood that it
will be removed from the circulation within the reticuloendothelial system (
Johnson et al., 1986; Beutler, 1983; Arese et al., 1986). With the consequent loss of
red cells hematopoiesis is increased as the body attempts to maintain normal
vascular functioning, and there is a shower of reticulocytes (young erythrocytes
YEARBOOK OF PHYSICAL ANTHROPOLOGY
[Vol. 36, 1993
TABLE I. Classification of G6PD variants
Enzyme deficiency with chronic nonspherocytic hemolytic anemia.
Class II variants
Severe enzyme deficiency. Less than 10% of the activity of G6PD B.
Class III variants
Moderate to mild enzyme deficiency. Ten percent to 60% of
the activity of G6PD B.
Class IV variants
Normal activity. Sixty percent to 150% the activity of G6PD B.
Increased activity. More than 150% the activity of G6PD B.
After Luzzatto and Mehta (1989) and Beutler (1990).
released from the bone marrow). Reticulocytes normally account for less than 1%
of total red cells, but following hemolysis may comprise up to 15% of erythrocytes.
GLUCOSE-6-PHOSPHATE DEHYDROGENASE VARIANTS
G6PD is a cytoplasmic enzyme that is found in all cells of the body. The active
form of the enzyme is either a dimer or a tetramer consisting of identical subunits.
The primary structure of a single subunit polypeptide chain consists of 515 amino
acids (Luzzatto and Mehta, 1989). G6PD B is the enzyme found in most people
worldwide and it is identical to that of the chimpanzee and gorilla (Luzzatto and
Battistuzzi, 1985). In populations outside of tropical and semitropical regions it is
virtually the only G6PD isoenzyme, except for rare private mutations, as no vari-
ants have reached polymorphic frequencies ( > .1%) in populations in these areas (
Luzzatto and Battistuzzi, 1985). Of all human loci, the G6PD locus is the most
polymorphic. Over 300 variants have been fully described (Luzzatto and Battis-
tuzzi, 1985; Beutler, 1990; Luzzatto and Mehta, 1989). In their comprehensive
review, Luzzatto and Mehta indicate that 77 of these variants have reached poly-
morphic frequencies ( > .1%). Eleven of these polymorphic alleles have normal
activity and 66 of the variants have decreased activity and are called deficient
variants (Luzzatto and Mehta, 1989). In a more recent overview Beutler (1992)
states that over 400 variants have now been described, but does not provide an
indication of how many of these variants have reached polymorphic frequencies.
Enzyme variation has traditionally been classified by means of electrophoretic
mobility determined by starch gel electrophoresis and as a function of enzyme
variant activity compared to the activity of G6PD B. The standard for activity is the
rate at which NADP+ is reduced by glucose-6-phosphate with G6PD B as the
catalyst. On this basis, enzyme variants are classified as fast, normal, and slow in
terms of electrophoretic mobility, and as Classes I—V based on enzyme activity
relative to G6PD B (Betke et al., 1967; Luzzatto and Mehta, 1989; Beutler, 1983,
Table 1 shows the classification of enzyme variants by activity level. Enzyme
variants which fall into each activity class are further characterized in terms of
electrophoretic mobility and unique variants are given specific names. The Class I
variants are very rare and code for kinetically unstable enzymes that produce
severe G6PD deficiency with variable degrees of hemolytic anemia (Beutler, 1983).
These variants have not reached polymorphic frequencies and are not relevant to
our discussion. Class II variants are severely deficient variants with less than 10%
of the activity of G6PD B, while Class III variants are more moderately deficient
with 10 to 60% of the activity G6PD B. Forty-four of the 109 Class II variants (40.
4%) and 22 of the 75 Class III variants (29.3%) listed by
G6PD DEFICIENCY AND FALCIPARUM MALARIA
Luzzatto and Mehta (1989) have reached polymorphic frequencies of >.1% in
various human populations and are associated with adaptation to falciparum
malaria (see below).
Some important regional polymorphic variants
G6PD A is a normal variant (Class IV) that has 90% of the activity of G6PD B (
Luzzatto and Mehta, 1989). The allele coding for this variant is probably the most
common variant worldwide as it reaches frequencies of approximately .20 in sub-
Saharan African populations (Beutler, 1990:152). Vulliamy and co-workers (1988)
indicate that this variant derives from a single amino acid substitution of aspartic
acid for asparagine at amino acid number 126, and that this was the result of an
adenine to guanine substitution at nucleotide number 376.
G6PD A (–) is a Class II deficient variant which has 10 to 20% of the activity of
G6PD B and which reaches allele frequencies of about .15 in sub-Saharan African
populations (Luzzatto and Mehta, 1989). It appears that this variant has arisen
from the G6PD A variant as both have aspartic acid substitutions at amino acid
number 126. The G6PD A (–) variant has an additional guanine to adenine sub-
stitution at nucleotide 202 at the genetic level leading to a valine to methionine
substitution at the protein level at amino acid number 67 (Vulliamy et al., 1988).
In actuality, there is some molecular heterogeneity in G6PD A (–), with the nu-
cleotide 202 substitution being the most common, but with alternative substitu-
tions occurring at nucleotides 680 and 968 (Beutler et al., 1989).
G6PD Mediterranean is a Class II deficient variant with enzyme activity that is
less than 10% of the activity of G6PD B; it reaches allele frequencies of between .10
and .25 or higher in many populations in the circum-Mediterranean region and the
Near and Middle East (Luzzatto and Mehta, 1989). This variant is a consequence
of a cytosine to thymine substitution at nucleotide number 563 resulting in a
serine to phenylalanine substitution at amino acid number 188 (Vulliamy et al.,
1988). There are many similar Class II variants that have reached polymorphic
frequencies in the circum-Mediterranean region (Cagliari, Sassari, El Fayoum),
South Asia (Hong Kong, Canton, Mahidol), and elsewhere. Most appear to be the
consequence of point mutations resulting in single amino acid substitutions that
have variable effects on activity and electrophoretic mobility. The characteriza-
tion, classification, and distribution of these variants have been described in detail
in a number of reviews (Luzzatto and Battistuzzi, 1985; Luzzatto and Mehta, 1989;
Beutler, 1990, 1991, 1992).
The effect of red cell age on erythrocyte G6PD activity
G6PD is an age-dependent enzyme. Within normal G6PD B red blood cells the
activity of G6PD declines exponentially, with a half-life of 62 days. However,
despite this loss of enzyme activity older G6PD B red cells contain sufficient G6PD
activity to maintain GSH levels in the face of an oxidant stress and the life span of
the average G6PD B red cell is 100 to 120 days.
In erythrocytes with G6PD A (–) the defect is due to greater enzyme instability.
Newly formed G6PD A (–) red cells have the same enzymatic activity as newly
formed erythrocytes from G6PD B individuals. However, the G6PD activity of these
cells declines rapidly. The half-life of G6PD A (–) erythrocyte is only 13 days, and in
the G6PD A (–) individual the red cell population is composed of a mixture of
erythrocytes of continuously decreasing levels of activity.
In the G6PD Mediterranean individual there is an even greater enzyme insta-
bility as the half-life of these erythrocytes is only about 8 days. Reticulocytes that
are released into the circulation in G6PD Mediterranean individuals already have
reduced levels of G6PD and mature erythrocytes have enzyme levels usually below
1% normal activity (Piomelli et al., 1968; Piomelli, 1986; Luzzatto and Testa, 1978;
Kirkman and Gaetani, 1986).
YEARBOOK OF PHYSICAL ANTHROPOLOGY
[Vol. 36, 1993
TABLE 2. Drugs and chemicals associated with significant hemolysis in
individuals with G6PD deficiency
Definite association
Possible association
Vitamin K analogues
Trinitrotoluene (TNT)
After Luzzatto and Mehta (1989).
Hemolysis in G6PD deficient individuals
Because the G6PD deficient erythrocyte only has a limited ability to deal with an
increased oxidant stress (see above), G6PD deficient individuals may experi-ence
hemolysis (which at times is explosive and severe) under a variety of circum-
stances: when exposed to a number of drugs and chemicals, including several
antimalarial drugs (see Table 2); during infections; during diabetic acidosis; upon
consumption of fava beans (Vicia faba) in G6PD Mediterranean and other Class II
deficient individuals; and as the result of a number of common exposures to envi-
ronmental chemicals (Luzzatto and Mehta, 1989; Beutler, 1983; Belsey, 1973;
Arese et al., 1986; Chevion et al., 1982; Calabrese, 1984). This hemolysis produces a
significant degree of morbidity and mortality in G6PD deficient individuals.
However, the increased vulnerability of the G6PD deficient erythrocyte to oxidant
stress is the mechanism underlying its relative protection against falciparum par-
asitization (see below).
The genetic locus for G6PD in humans and all mammals is located on the telo-
meric region of the long arm of the X-chromosome (band Xq28) distal to the fragile
site Xq27.3. It is closely linked to the loci for color blindness, hemophilia A, and
adrenoleukodystrophy. These linkage groups are very stable and are the same in
all mammals. Interestingly, the G6PD of Drosophila melanogaster is also sex-
linked (Beutler, 1983, 1990; Luzzatto and Battistuzzi, 1985; Luzzatto and Mehta,
1989; WHO Working Group, 1989). Since it is sex-linked there are five possible
genotypes in populations in which the G6PD locus is polymorphic. The frequency
of the deficient condition is higher in males than females since males, being hem-
izygous, only need one copy of the allele to express the full deficient condition while
females need two deficient alleles.
Hemizygous deficient males and homozygous deficient females express the same
degree of enzyme deficiency, while hemizygous normal males and homozygous
normal females also have comparable enzyme levels. Due to the random deacti-
vation of one X-chromosome during embryological development in the female,
heterozygous females actually have two populations of red cells (G6PD normal and
G6PD DEFICIENCY AND FALCIPARUM MALARIA
G6PD deficient) (Lyon Mosaic Effect) with varying total G6PD activity depending on
the relative proportions of these two groups of erythrocytes. Total G6PD activity in
heterozygous females may range from near-normal to near-deficient (Luzzatto and
Battistuzzi, 1985; Beutler, 1983).
Malaria is a febrile illness caused by sporozoa of the genus Plasmodium, four
species of which infect humans: P. falciparum, P. vivax, P. ovale, and P. malariae.
The malarial parasites undergo a developmental cycle in the female anopheline
mosquito, which is the vector. They are transmitted to the human host following a
bite by the mosquito, rapidly enter the liver where they undergo a developmental
phase of varying duration among the four species (pre-erythrocytic phase), and
then enter the red blood cell (intra-erythrocytic phase) where they continue their
multiplicative cycle. The asexual erythrocytic parasite is the stage in the life cycle
that causes disease. This is characterized by fever, chills and sweats (which vary in
periodicity among the different species), anemia, and enlargement of the liver and
spleen (Woodruff, 1978; Miller, 1985; Strickland, 1991).
P. falciparum (malignant tertian) is the most severe form of malaria and causes
the greatest degree of morbidity and mortality. It is found in warm, moist climates
and is distributed over most of tropical Africa, parts of India and Pakistan, South-
east Asia including Indonesia and New Guinea, and Central and South America. P.
vivax (benign tertian) malaria is widespread in the tropics and subtropics and in
some temperate regions. P. ovale (ovale tertian) is uncommon and is irregularly
distributed in Africa and South America. P. malariae (quartan) is also less common
and is found in the tropics of East and West Africa, India, and Central and South
Immunity to malaria is primarily directed against the asexual erythrocytic par-
asite and only develops after prolonged and repeated infections. Immunity usually
does not prevent re-infection, but reduces the severity of the disease or leads to an
asymptomatic infection. Immunity is species-specific, and in P. falciparum is usu-
P. falciparum, P. vivax, and P. ovale invade reticulocytes (immature erythro-
cytes) preferentially, but P. falciparum can invade erythrocytes of all ages. This is a
significant phenomenon since G6PD activity is markedly reduced in older eryth-
rocytes. This renders mature erythrocytes more vulnerable to the oxidant stress
produced by parasitization, and is the mechanism of protection produced by the
G6PD deficient condition (see below). P. malariae tends to infect mature erythro-
cytes (Woodruff, 1978; Miller, 1985; Strickland, 1991).
The following sections present a discussion of the population and in vitro studies
evaluating the relative protection of the G6PD deficient erythrocyte against fal-
ciparum parasitization and the mechanism through which this protection takes
place. This review covers a large sample of the most informative studies, but is not
all-inclusive. Again, the goal of this review is to present the evidence indicating that
all G6PD deficient genotypes are relatively protected against falciparum ma-laria,
and to illustrate some of the difficulties involved in such epidemiologic in-
THE GEOGRAPHICAL DISTRIBUTION OF POLYMORPHIC FREQUENCIES OF G6PD
DEFICIENCY RELATIVE TO THE DISTRIBUTION OF FALCIPARUM MALARIA
The G6PD locus is the most highly polymorphic human genetic locus with over
300 known genetic variants of which at least 77 have reached polymorphic fre-
quencies (Luzzatto and Mehta, 1989; Luzzatto and Battistuzzi, 1985). All of the
polymorphic alleles occur in populations living in areas where falciparum malaria
is or was endemic or in populations derived from these areas, and 66 of the 77
alleles that have reached polymorphic frequencies code for variable degrees of
G6PD deficiency. The large number of G6PD deficient alleles that have reached
polymorphic frequencies in tropical and subtropical environments where malaria
YEARBOOK OF PHYSICAL ANTHROPOLOGY
[Vol. 36, 1993
has been endemic and the total absence of polymorphic frequencies of these alleles
at more extreme latitudes suggest a relationship between G6PD deficiency and
Stronger support for the relationship between G6PD deficiency and adaptation
to falciparum malaria comes from the observation that there is a general covari-
ation in distribution of G6PD deficiency and falciparum malaria worldwide and the
more specific covariation in the distribution of the G6PD A (–) allele and the allele
for hemoglobin S in sub-Saharan Africa (Luzzatto and Battistuzzi, 1985). The
within-population variation in G6PD Mediterranean allele frequency with changes
in altitude and with the historical pattern of malaria distribution in Sardinia (
Siniscalco et al., 1966) lends further support to the view that G6PD deficiency is
associated with adaptation to falciparum malaria, although Brown (1981) has
questioned these relationships as have Gloria-Bottini et al. (1980). However, these
relationships would be more meaningful and precise if they could be viewed within
the context of population differences in consumption of food crops containing
naturally occurring antimalarial substances of plant origin (e.g., fava beans) (see
below). Unfortunately, large data sets that evaluate the relationships between
quantitative measures of historical malarial morbidity (which distinguish between
falciparum and vivax infection), G6PD deficiency as determined by quan-titative
assay, and population differences in the consumption of food crops high in
antimalarial activity do not exist.
Some critiques of the relationship between G6PD deficiency and falciparum
malaria are unfounded and are based solely on the fact that these relationships are
imperfect. An oft-cited article by Kidson and Gorman (1962) is an example. They
questioned the relationship between interpopulation variation in G6PD deficient
allele frequency and falciparum malaria in New Guinea and New Britain based on
the observation that there are somewhat elevated frequencies of G6PD deficiency
in several highland populations where malaria is rare and relatively low frequen-
cies of G6PD deficiency in some coastal populations where malaria is endemic.
However, when the data they presented are pooled for all of their highland popu-
lations and compared to similarly pooled data for all of their lowland populations,
the overall allele frequency for G6PD deficiency is 5.3% in the malarious lowlands
and 0.93% in the non-malarious highlands. This is entirely consistent with the
G6PD deficiency-malaria hypothesis, given the fact that there must be some gene
flow between these adjacent regions.
POPULATION STUDIES
Population studies supporting a protective effect of G6PD deficiency in
hemizygous males and homozygous females
Allison and Clyde (1961). An early field investigation by Allison and Clyde (1961)
was carried out on 532 children from 4 months to 4 years of age in present-day
Tanzania in an area where falciparum malaria was holoendemic. Two-thirds of the
subjects were recruited from village surveys that were done on virtually all of the
age-appropriate children. The remaining one-third of the sample was drawn from
infant welfare and out-patient clinics. Parasite rates and densities were signifi-
cantly lower in G6PD (–) males, but not females, although significant differences
were almost reached in the female group. Parasite rates and densities were ap-
proximately equivalent in G6PD (–) males and subjects with sickle cell trait, and
both were significantly lower than observed in children who were not G6PD defi-
cient or who did not have sickle cell trait.
The strength of this study lies in the fact that the children observed were fairly
young and thus the development of immunity to malaria was not complete (Brown,
1969; Jarra, 1983). The acquisition of relative immunity with age is a factor which
greatly confounds the interpretation of the results of studies of older children and
G6PD DEFICIENCY AND FALCIPARUM MALARIA
adults. A second strength of this study lies in the fact that most of the subjects were
sampled from village surveys rather than from among febrile hospitalized individ-
uals and that the survey sample comprised almost the entire population of age-
appropriate children. Such a study sample is much less likely to show selection
bias than a sample of hospitalized children (see below). Finally, although the rate
of malarial parasitization was found to be elevated in G6PD normal subjects,
perhaps the more significant finding is the increase in parasite densities in the
normals as there is a well-established relationship between parasite density and
mortality from malaria (Allison, 1957; Brown, 1969).
Gilles et al. (1967). Gilles et al. (1967) also reported a protective effect of G6PD
deficiency against falciparum malaria among 100 Nigerian children 4 months to 4
years of age. These children represented a sample of children admitted over a 3-
year period to the pediatric emergency room at University College Hospital, Ibadan,
who were found to have severe falciparum malaria with parasite counts over 100,
000 per mm3. A group of similarly aged children attending the same clinic for
different reasons served as a control group. The frequency of G6PD deficiency
among subjects with severe malaria was significantly lower among both males and
females compared to the frequency of the trait in the control population. The
protective effect of G6PD deficiency was independent of sickle cell trait status,
which was also evaluated and shown to have a protective effect against falciparum
The strengths of this study are that the subjects are young enough so that the
development of immunity is not complete; the degree of falciparum parasitization is
at levels at which mortality normally occurs, and thus differences in the fre-quency
with which G6PD deficient and normal subjects experience these parasite densities
reflect differential exposure to significant selective pressures; and there was no
confounding of the protective effect of G6PD deficiency and sickle cell trait.
Butler (1973). A study by Butler (1973) suggests that G6PD deficiency provides
protection against falciparum malaria among non-immune adults. This work was
carried out on a sample of 277 African-American soldiers in Vietnam. Subjects
represented virtually all African-American patients on a ward or attending a clinic
on a particular study day. "Most subjects" were taking an antimalarial prophylac-
tic regimen of chloroquine and primaquine. Subjects having a history of malaria in
Vietnam were considered malaria patients and parasitological information from the
previous hospitalization was taken from the hospital charts.
The G6PD deficient subjects (13.7%) had a significantly lower incidence of ma-
laria (21%) than the G6PD normal group (39%), although the parasite densities did
not differ. Approximately 80% of cases were falciparum malaria. These findings are
particularly interesting in that they evaluate the relationship between G6PD
deficiency and malaria incidence in a group of adults who have no exposure, and
thus absolutely no degree of immunity, to this disease. This study thus avoids the
possibility of sampling bias that occurs in studies carried out on children who have
lived their entire lives in endemic areas and who are sampled in population sur-
veys or when they present at hospital. In such studies the G6PD normal and
deficient groups may have significantly different degrees of acquired immunity to
falciparum malaria due to chance, despite the fact that they may be closely
matched for age, and this factor could obscure the relationship between G6PD
deficiency and protection against falciparum malaria in a random fashion from
study to study.
That the G6PD deficient subjects showed lower infection rates despite the wide-
spread use of antimalarial prophylaxis is particularly impressive and is consistent
YEARBOOK OF PHYSICAL ANTHROPOLOGY
[Vol. 36, 1993
with the findings of in vitro studies which indicate that divicine and isouramil (the
naturally occurring antimalarial substances in fava beans) have a more profound
antimalarial effect in G6PD deficient than normal erythrocytes (Golenser et al.,
1983, 1988) (see below). These African-American servicemen are relatively
resistant to P. vivax due to their high frequency of Duffy negative phenotype which
conveys almost complete protection against vivax parasitization (Livingstone,
1984). This circumstance removes a confounding factor that occurs in many Asian
studies in which it is difficult to evaluate the relative protection of G6PD deficient
genotypes against falciparum malaria due to their possibly greater vulnerability to
vivax parasitization (see below).
Although these findings are provocative there are several limitations to this
study. One is that the subjects were not randomly recruited into the sample. There-
fore, there may have been selection bias, although 95% of the hospitalized subjects
approached agreed to participate in the study. Also, the author reports that "most
subjects" were taking malaria prophylaxis of chloroquine and primaquine. Such
imprecise assessment of drug use makes it impossible to assess differential anti-
malarial exposure between G6PD deficient and G6PD normal subjects. Neverthe-
less, despite these limitations this study is the only field investigation that has
evaluated the possible protective effect of G6PD deficiency against falciparum
malaria in a population where the immune status of the subjects was not a con-
founding factor.
Kar et al. (1992). Kar et al. (1992) evaluated the relationship between erythrocyte
G6PD status and the prevalence of Plasmodium vivax and P. falciparum parasit-
ization in a "random sample" of 708 Ao Nagas subjects of both sexes from Na-
galand in northeast India. The age of the subjects was not given. Of these individ-
uals, 324 (45.8%) had malarial parasites and constituted the malarial sample and
384 (54.2%) were uninfected and served as a control sample.
Both P. vivax and P. falciparum parasitization rates were significantly greater in
G6PD normal than G6PD deficient males, and parasitization rates with both spe-
cies were also greater among G6PD normal females than heterozygous G6PD
deficient females. There were no significant differences in malarial parasitization
between homozygous G6PD deficient females and G6PD normal subjects, but the
number of homozygous G6PD deficient females was quite small.
The major strength of this study is that the subjects were "randomly selected" by
sex so that there should not be a selection bias, although the authors provide no
information about the source of the subjects and the precise manner in which they
were sampled. Sample size is also quite large.
The main weakness of this study is that there is no indication whatsoever of the
age of the subjects. Since acquired relative immunity is established by about 5
years of age, it is highly preferable that subjects in such studies be younger than
5 (see above). The period prior to the acquisition of immunity is the time during
which a biologic trait like G6PD deficiency, or sickle cell trait, should convey its
maximal relative protection, and when selection against G6PD normal individuals
should be most intense.
One must assume that the sample is composed of adults. If this is the case, then
these are even more important findings since they indicate that both G6PD defi-
cient males and heterozygous deficient females have much lower parasite rates
than G6PD normal subjects in an area where malaria prevalence is extremely high (
45.8%). This finding suggests that the G6PD deficient condition still confers rel-
ative protection in the adult, even after immunity is acquired.
Another important finding of this study is the fact that the relative protection of
the G6PD deficient condition extends to both P. vivax and P. falciparum, and in
both sexes. P. vivax actually accounts for 56.8% of the cases of malaria, so it is more
common that falciparum in this region and possibly a significant selective vector (
although it is invariably a less severe form of malaria).
G6PD DEFICIENCY AND FALCIPARUM MALARIA
Population studies demonstrating a protective effect of G6PD deficiency in
Luzzatto et al. (1969). Luzzatto et al. (1969) presented an elegant demonstration of
the protective effect of G6PD deficiency against falciparum malaria by evaluating
differential parasitization of deficient and non-deficient red blood cells in heterozy-
gous females. Subjects were 1- to 5-year-old Nigerian children with acute falci-
parum malaria. Due to the Lyon Mosaic Effect, approximately one-half of the
erythrocytes of heterozygous females contain normal levels of G6PD while the other
half are severely deficient. Normal and deficient cells can be distinguished
microscopically using the methemoglobin elution technique. In the 20 heterozy-
gous subjects studied, the rate of parasitization was two to 80 times greater in the
G6PD normal than in the G6PD deficient erythrocytes in the same individual.
Comparison of parasite rates in the G6PD deficient red cells and the small number of
G6PD normal erythrocytes in homozygous females (n = 5) led to similar find-ings.
Between 1 and 15 percent of erythrocytes had normal G6PD activity in the
hemizygous deficient males and homozygous deficient females with falciparum
malaria. These were probably young reticulocytes. In these subjects the parasite
rates in the G6PD normal erythrocytes were between six to 81 times greater than
the rate of parasitization in the G6PD deficient erythrocytes in the same individ-
This study is particularly convincing because it demonstrates differential levels
of parasitism in G6PD deficient and non-deficient red cells in the same individual.
The confounding effect of age differences among subjects and differential immu-
nity is thus neatly avoided as is the possible problem of differences in intake of
antimalarial substances of dietary origin among the different G6PD genotypes (see
Bienzle et al. (1972). In another series of studies in Nigeria, Bienzle, Luzzatto, and
co-workers (Bienzle et al., 1972, 1979; Guggenmoos-Holzmann et al., 1981; Luz-
zatto and Bienzle, 1979) presented evidence suggesting that the G6PD deficient
heterozygous females were relatively protected against falciparum malaria, but not
the more severely deficient hemizygous males and homozygous females. These
studies are discussed more fully below in the section on negative findings for a
protective effect of G6PD deficiency in hemizygous and homozygous deficient sub-
Kar et al. (1992). See above.
Population studies indicating no relative protection of hemizygous and
homozygous G6PD deficient individuals against falciparum malaria
Bienzle et al. (1972). One of the most comprehensive and frequently cited field
studies of the relationship between G6PD deficiency and falciparum malaria is that
of Bienzle et al. (1972) in Nigeria on 702 children aged 9 months to 6 years
presenting at hospital with acute febrile illness and 189 healthy young adults aged
14 to 20 years. Sixty-six percent of the children were found to have falciparum
G6PD genotypes were determined by a combination of quantification of the
enzyme activity, qualitative analysis by starch-gel electrophoresis, and cytochem-
ical localization of enzyme activity in individual cells. The authors reported that
YEARBOOK OF PHYSICAL ANTHROPOLOGY
[Vol. 36, 1993
among the children with malaria, G6PD A males and G6PD A-/G6PD B females
were less frequent compared to the group of children who did not have malaria, but
these differences did not reach statistical significance. These genotypes also
showed a lower frequency of high parasite counts compared to the other genotypes,
but it is unclear from the report whether these findings reached statistical signif-
In a later reanalysis of the same data, Bienzle et al. (1979) reported that the
malaria infection rates in the children up to 6 years of age were highest in hem-
izygous and homozygous G6PD deficient subjects at all ages, with the G6PD nor-
mal subjects having the next highest infection rate, and the G6PD A-/G6PD B
heterozygote females having the lowest infection rates at all ages. Their re-anal-
ysis of these data showed that the G6PD A-/G6PD B subjects had the lowest
median, mean, 90th percentile, and maximum parasite densities compared to the
G6PD normal and the combined hemizygous and homozygous deficient group and
that these differences were significant (P = .025). These data were re-analyzed
further by Guggenmoos-Holzmann et al. (1981) with similar conclusions.
The viewpoint that emerged from the various publications emanating from this
single study is "that there is no protection against malaria in G6PD deficient
hemizygous (Gd A-) boys or homozygous (Gd A-/Gd A-) girls" (Luzzatto and Bienzle,
1979:1183) and that "epidemiologic data indicate strongly that G6PD deficiency
can confer relative resistance against Plasmodium falciparum malaria, and clinical
data indicate that this is confined to heterozygous females" (Luzzatto and Mehta,
1989:2237). This position is reiterated by Luzzatto and Battistuzzi (1985) and
Usanga and Luzzatto (1985).
This field investigation is the most often-cited study in the current literature on
the relationship between G6PD deficiency and malaria, probably in consequence
of: 1) the fairly large sample; 2) the great authority of Bienzle and Luzzatto rela-tive
to the determination of enzyme activity and the precise genotypic character-ization
of the subjects; and 3) Luzzatto's frequent reviews which refer to this in-
vestigation. There are many strengths to the study and the findings of relative
protection of G6PD A-/G6PD B heterozygotes from falciparum malaria seem well-
established. Nevertheless, there are aspects of the study design that suggest the
possibility of significant selection bias which may undermine the investigation's
ability to detect any relative protection afforded to the hemizygous and homozy-
gous deficient genotypes.
The major methodological problem with this study, and all those that are based
on acutely ill subjects seen at hospital, is the fact that hemizygous and homozygous
G6PD deficient individuals may be less likely to become infected with falciparum
malaria (Allison and Clyde, 1961; Gilles et al., 1967; Butler, 1973; Kar et al., 1992)
, but that when they do become infected with malaria they are more likely to be
brought to hospital. This is due to a more profound, malaria-associated, hemol-ysis
as a consequence of the greater vulnerability of the G6PD deficient erythro-cyte to
the oxidant stress of malarial parasitization (see below). Therefore, the question is
whether the malarial parasitization rate in the G6PD deficient subjects in this study
by Bienzle et al. (1972) is representative of the parasitization rate among G6PD
deficient subjects of this age in the community at large, or whether, for the above
reasons, it is a sample of G6PD deficient subjects biased in the direction of greater
parasitization rates because G6PD deficient subjects are more likely to go to
hospital when they have malaria (more hemolysis) than G6PD normal subjects.
It is also possible that when falciparum parasitization does become established
in G6PD deficient children it may present in a more severe form (even though it
may be less frequent) if they have had fewer malarial infections due to the pro-
tection of the G6PD deficient condition and thus have not developed the same
degree of acquired immunity as age-matched G6PD normal subjects.
Under these circumstances, this selection bias could only be avoided with a study
design that samples differential falciparum parasitization among all children in a
G6PD DEFICIENCY AND FALCIPARUM MALARIA
community, not just those who are brought to hospital. This was the design em-
ployed by Allison and Clyde (1961), which is, unfortunately, the only population
study that clearly employed such an approach.
Martin et al. (1979). In another study in Nigeria, Martin et al. (1979) evaluated the
relative frequency of G6PD deficiency in Nigerian children with malaria and without
malaria. Subjects were 30 children with a mean age of 3.6 years who were brought
to hospital with convulsions and who had P. falciparum densities greater than 100,
000/µl and 38 children with a mean age of 3.4 years without parasites in their blood
smears who had been brought to the hospital because of convulsions from causes
other than malaria. The authors reported that 20% of the subjects with malaria were
G6PD deficient compared to 21% of the controls and concluded that G6PD
deficiency does not protect against falciparum malaria.
Unfortunately, there are several methodological problems that greatly limit the
usefulness of these findings. First, there could be significant sampling bias, as
noted in the discussion of Bienzle et al. (1972) above, since G6PD deficient indi-
viduals with malaria hemolyze more readily than G6PD normal subjects and thus
may be brought to hospital more frequently. A second difficulty is that both the
experimental and control groups were chosen from among children presenting at
hospital with convulsions, which is likely to produce a highly biased sample of
subjects. This is a consequence of the well-established fact that G6PD deficient
newborns experience a higher rate of neonatal jaundice, more intense hyperbilir-
ubinemia, and a higher prevalence of kernicterus than do non-deficient subjects (
Piomelli, 1986; Valaes et al., 1985). A greater prevalence of minimal cerebral
dysfunction and various degrees of brain damage is also seen in G6PD deficient
newborns (Meijer, 1984; Piomelli, 1986; Singh, 1986). A group of children sampled
on the basis of having convulsions associated with falciparum malaria is much
more likely to include a disproportionate number of G6PD deficient individuals,
because G6PD deficient children are more likely to have minimal cerebral injury
which will be expressed only under conditions of stress such as malaria. A sample of
children experiencing convulsions unassociated with malaria is more likely to be
composed primarily of individuals with more severe neurological defects that are
not associated with G6PD deficiency. Therefore, a study sampling on the basis of
presentation with convulsions is likely to produce spurious results. Given these
limitations and the very small sample size, this study cannot be given much weight
in evaluating the question of the relative protection of the various G6PD genotypes
against falciparum parasitization.
Kruatrachue et al. (1962). Another negative finding on the protective effect of G6PD
deficiency is a study by Kruatrachue et al. (1962) on 519 boys between 1 and 9 years
of age in Thailand. Among the 203 boys aged 1 to 3 years, falciparum malaria was
more common in G6PD deficient boys than in the G6PD normal subjects (23.7% to
19.4%) as was vivax malaria (39.5% to 21.9%) and the difference between the two
groups for vivax infestation was statistically significant.
It is not clear from the report how the subjects were sampled. The authors only
state that two areas of endemic malaria (32%, 51%) were chosen and state at one
point that "most of our patients who had fever"' (1962:1186), suggesting that these
were hospital or clinic patients. If the sampling took place in a hospital setting,
then this study is open to the same criticism of all such studies (see above) in that
there may be significant selection bias because of the greater likelihood that G6PD
deficient subjects will hemolyze, and thus be brought to hospital, if they contract
If the subjects had been sampled randomly, the findings of this investigation
would have greater authority and would undermine the view that G6PD deficiency
protects against falciparum parasitization. However, this study may help answer
YEARBOOK OF PHYSICAL ANTHROPOLOGY
[Vol. 36, 1993
a part of the puzzle rather than erode the well-supported finding that G6PD defi-
ciency provides relative protection against falciparum infection. The higher rate of
malarial parasitization of G6PD deficient boys in this investigation is a conse-
quence of vivax malaria (39.5% in deficients, 21.9% in G6PD normals) whereas the
falciparum parasitization rates of children 1 to 3 years of age were not significantly
different (23.7% in deficients, 19.4% in G6PD normals).
As the authors of this report note, P. vivax tends to parasitize preferentially
young red blood cells which exist in elevated numbers in G6PD deficient subjects
due to recurrent self-limited hemolysis and some associated reticulocytosis. If
G6PD deficient subjects are more susceptible to vivax malaria this probably is not
a major selective disadvantage as the infection is likely to be confined to the small
number of reticulocytes, and P. vivax is self-limited and generally not life-threat-
In areas where both vivax and falciparum malaria are endemic, such as South
Asia, a greater susceptibility of G6PD deficient individuals to a mild vivax infec-
tion that is limited to reticulocytes may actually be advantageous if it decreases the
likelihood that those same G6PD-rich erythrocytes will be invaded by the more
virulent P. falciparum. Following this logic, the lower reticulocyte levels in G6PD
normal individuals would render them less susceptible to vivax and thus more open
to falciparum infestation. Although these data of Kruatrachue et al. (1962) from
Thailand show a higher degree of vivax parasitization of G6PD deficient subjects,
they do not demonstrate a concomitantly lower level of falciparum parasitization in
these individuals. However, there may be seasonal variability in such a phe-
nomenon with the protective effect occurring only during very peak periods of vivax
endemicity. Under these circumstances, the relative advantage of the G6PD
deficient genotypes could only be assessed by evaluating infection rates for both
species in all genotypes throughout an annual cycle.
Vivax malaria does not exist in sub-Saharan Africa because sub-Saharan Afri-
can populations have virtually 100% (1.0) frequency of the Duffy negative allele
which conveys almost total protection against vivax parasitization (Livingstone
1984). Therefore, it should be quite a bit more straightforward to evaluate the
relative protection of G6PD deficient genotypes against falciparum malaria in
studies carried out in this region.
Powell and Brewer (1965). Powell and Brewer (1965) carried out an experimental
study of susceptibility to induced malaria in 16 volunteer subjects who were
African-American males in prison. Eight subjects were G6PD deficient with a mean
age of 32 years and eight had normal G6PD activity and a mean age of 33 years.
None of the subjects had a prior history of malaria, or a disease suggesting
malaria, or had visited an area where malaria was common. Body weights were
comparable in the two groups and none of the subjects had hemoglobin variants.
The subjects were exposed to Anopheles stephensi mosquitos infected with the
McLendon strain of P. falciparum and all subjects except one G6PD normal indi-
vidual developed patent falciparum infections. Mean length of the prepatent period
and mean levels of parasitemia did not differ between the two groups and chloro-
quine antimalarial therapy was initiated when parasite levels first exceeded 5,000
per mm3. Therefore, this investigation appears to provide strong experimental
evidence indicating that G6PD deficiency does not protect against falciparum in-
However, the major weakness of this study is that it is experimental rather than
naturalistic. Subjects were bitten by ten falciparum-infected Anopheles stephensi
mosquitos resulting in a massive parasite dose that is uncommon or non-existent
under natural conditions. Livingstone (1958:536) states that in West Africa "the
average number of infective bites per person per year is always greater than about
G6PD DEFICIENCY AND FALCIPARUM MALARIA
five, and in some areas ranges up to 100 or more." It is likely that the biochemical
advantage of the G6PD deficient condition evolved in response to something like
this modest infection rate and not as an adaptation to massive artificial levels of
parasitization that would result as a consequence of an individual being exposed to
ten infected mosquitos simultaneously. Also, in vitro studies have suggested that
maximal antimalarial protection is achieved when G6PD deficient erythrocytes are
treated with naturally occurring antimalarial substances from food sources (
Golenser et al., 1988). Such data suggest that experimental studies like the one
carried out by Powell and Brewer do not reproduce the natural conditions under
which selection has taken place (natural levels of exposure and concomitant con-
sumption of traditional dietary items), and provide further support for the view
that community studies represent the optimal study design for evaluating the
relative susceptibility of the various G6PD genotypes to malarial parasitization. An
experiment such as the one carried out by Powell and Brewer is somewhat akin to
an hypothetical study in which eight lightly pigmented and eight darkly pig-
mented individuals would be exposed to intense artificial ultraviolet radiation
resulting in skin cancer in all of the subjects, with the conclusion that darkly
pigmented skin does not provide relative protection against the carcinogenic ef-
fects of ultraviolet radiation.
IN VITRO STUDIES OF MALARIAL PARASITE GROWTH IN G6PD
DEFICIENT ERYTHROCYTES
With the development of techniques for the continuous culture of human
malarial parasites (Trager and Jensen, 1976) it became possible to experimentally
evaluate whether there is a protective effect of G6PD deficient erythrocytes against
P. falciparum parasitization. Clark et al. (1989) point out that it took decades to
develop these techniques largely because it was not realized that the parasites
only tolerated low levels of oxygen in vitro. Thus, from early on there were clues
indicating that malarial parasites were vulnerable to oxidant stress. These
observations suggested that genetic factors, such as G6PD deficiency, which
increase the likelihood of uncompensated oxidant stress to the red cell, might
provide some degree of antimalarial protection for the deficient erythrocytes. This
led to a series of in vitro investigations which illuminated how malarial parasites
are vulnerable to oxidant stress and how this phenomenon contributes to the
protective effect provided by G6PD deficient red cells against falciparum malaria.
As noted above, certain aspects of the life cycle of the erythrocyte also have an
influence on malarial parasitization. Since it lacks a nucleus and mitochondria,
the normal erythrocyte has a life span of about 120 days. The level of activity of
G6PD in erythrocytes declines with age, and the half-life of normal G6PD B is
about 62 days. However, G6PD in the erythrocytes of deficient individuals is much
less stable and the activity level decreases dramatically. Thus, the half-life of
G6PD A (–) is only 13 days and that of G6PD Mediterranean is just 8.5 days (
Luzzatto and Testa, 1978). Therefore, older G6PD deficient erythrocytes have very
low G6PD levels and are extremely vulnerable to oxidant stress, while reticulo-
cytes and young erythrocytes have much higher G6PD activities and are far less
susceptible to oxidant damage.
In early field investigations it was observed that in the normal course of para-
sitization P. falciparum and P. vivax both preferentially invade younger red cells
that are rich in G6PD (Allison and Clyde, 1961; Kruatrachue et al., 1962). That
observation raised the possibility that the low G6PD levels of G6PD deficient red
cells may be protective against Plasmodium parasitization. These observations
had informed the direction of the field studies evaluating the possible protective
effect of the G6PD genotypes against falciparum parasitization, and they also
informed the direction of the in vitro studies that were carried out beginning in the
latter half of the 1970s.
YEARBOOK OF PHYSICAL ANTHROPOLOGY
[Vol. 36, 1993
In vitro studies demonstrating a protective effect of G6PD deficiency against
Eckman and Eaton (1979) Eckman and Eaton (1979) observed a twofold increase
in GSH level in erythrocytes of Swiss white mice that had been infected with
Plasmodium berghei. In a series of experiments they demonstrated that this GSH
was largely parasitic in origin, rather than having been derived from the infected
red cells, and that the parasites were oxidizing red cell NADPH to maintain par-
asitic glutathione in the reduced form (GSH). From these observations on eryth-
rocytes in G6PD normal mice the authors then hypothesized that G6PD deficient
erythrocytes would protect against fulminant malaria infection because utilization of
NADPH by both the host erythrocyte and malaria parasite would overwhelm the
limited ability of the G6PD deficient red cells to regenerate NADPH and the
resultant decrement in GSH would lead to oxidant-induced hemolysis. They also
suggested that the accumulation of oxidized glutathione (GSSG) in the parasitized
G6PD deficient erythrocyte would inhibit parasite protein synthesis, an idea that
had received some experimental support in an earlier study by Kosower and Ko-
sower (1970).
Friedman (1979) cultured P. falciparum in normal and G6PD A (–) deficient
erythrocytes in conditions which either enhanced or inhibited oxidant damage.
Culture conditions under an atmosphere of 25% 02 increased oxidant stress. This
did not lead to a decrease in parasite multiplication in G6PD normal cells, but
produced a 50% decrease in multiplication in G6PD deficient cells. Addition of the
antioxidant compound vitamin E to the G6PD deficient culture mixture mitigated
the oxidant stress somewhat so there was only a 30% decrease in parasite multi-
plication. Addition of DTT (dithiothreitol), a thiol reducing agent, to the G6PD
deficient culture medium further mitigated the effect of the stress so that there
was no decrease in falciparum multiplication and thus a total abolition of the
protective effect of the G6PD deficient erythrocyte against falciparum parasitiza-
tion. In order to approximate more closely in vivo conditions, the GSH that was a
normal constituent of the culture medium was removed and under these circum-
stances P. falciparum multiplication was reduced by 62% in G6PD A (–) deficient
erythrocytes at 17% 02. In sum, this study demonstrated that P. falciparum mul-
tiplication was greater in G6PD normal than G6PD A (–) erythrocytes when an
additional oxidant stress was applied to the system, and the author suggested that
oxidants found in some foods could contribute to such an effect in vivo.
Roth et al. (1983)
A series of experiments by Roth et al. (1983) provided the first unequivocal
evidence that P. falciparum multiplication is retarded in erythrocytes from G6PD
deficient individuals under normal culture conditions. These studies were carried
out on blood samples drawn from G6PD normal subjects, G6PD hemizygous defi-
cient males, and G6PD heterozygous deficient females from Sardinia. None of the
subjects had hemoglobin abnormalities and the hemoglobin F content of all sub-
jects was less than 1%. Culture conditions were at 17% oxygen.
Five days after inoculation of P. falciparum into the culture the G6PD deficient
hemizygous and heterozygous cultures both showed levels of parasitemia about
one-third of that of the G6PD normal controls. There was a similar reduction in the
parasitemias in the two cultures despite the fact that the cultures from the het-
erozygotes contained 30 to 40% histochemically G6PD normal erythrocytes com-
pared to the hemizygous cultures which contained close to 100% G6PD deficient
The authors suggested that falciparum parasite growth inhibition was success-
ful in their experiment under normal culture conditions because the G6PD defi-
G6PD DEFICIENCY AND FALCIPARUM MALARIA
cient erythrocytes were from Sardinian subjects who were more severely G6PD
deficient, while the erythrocytes used by Friedman (1979) were from an individual
with the less severely deficient G6PD A (–) variant and thus required the aid of an
additional oxidant stress (25% 02 culture conditions) to show a protective effect.
Luzzatto et al. (1983)
Luzzatto et al. (1983) inoculated synchronized cultures of P. falciparum in par-
allel into G6PD Mediterranean deficient and G6PD normal erythrocytes and found
that the two types of cells were infected at about the same rate, but that there was
approximately a 40% decreased rate of parasite growth in the G6PD (–) erythro-
cytes by the second schizogonic cycle. Similar findings were observed with G6PD
Mahidol (–) and G6PD A (–). However, when they grew the Plasmodium parasites
through several passages in the G6PD Mediterranean (–) erythrocytes and eval-
uated their ability to invade G6PD ( + ) and G6PD (–) erythrocytes they found that
the parasitization rates were almost the same. It thus appeared that the surviving
parasites had adapted to the G6PD (–) host cells and were capable of invading
subsequent G6PD (–) erythrocytes almost as successfully as they were able to
invade G6PD ( + ) erythrocytes.
Usanga and Luzzatto (1985)
Usanga and Luzzatto (1985) conducted a series of experiments designed to ex-
tend their previous findings. They reported that when P. falciparum parasites were
grown through four successive cycles in G6PD A (–) red cells (adapted parasites) a "
slight, not significant, difference was observed" in G6PD A (–) infection rates
compared to G6PD A ( + ) infection rates, and that "in four separate experiments,
multiplication of these adapted parasites, measured in a fifth cycle in G6PD-defi-
cient (A-) RBC, was 79 ± 5% of the multiplication observed in transfer back to
G6PD-normal RBC" (1985:794).
The investigators then evaluated the hypothesis that adaptation to the G6PD
deficient erythrocytes was a consequence of induction of parasite G6PD enzyme
activity in the G6PD deficient environment. When they examined G6PD activity in
parasitized, compared to non-parasitized, G6PD (–) cells they found that G6PD
activity was two to five times higher in the parasitized cells. When they examined
G6PD extracts from parasitized G6PD A (–) erythrocytes they observed two bands of
electrophoretically different G6PD activity, one of which was comparable to host cell
enzyme while the other appeared to represent G6PD synthesized by P. falci-parum.
This process of induction did not occur when the parasites were grown through
four cycles of G6PD A (+ ), an enzyme variant with normal activity, suggesting that
this process of adaptation does not take place when the parasites invade
erythrocytes with normal levels of G6PD activity.
Usanga and Luzzatto (1985:795) concluded that this observation explains why
field studies have shown that "hemizygous G6PD-deficient males are not protected
against infection and also explains the protection of heterozygous females." They
argue that under in vivo conditions the Plasmodium parasites will adapt to the
G6PD deficient erythrocytes in hemizygous and homozygous deficient individuals
after a few cycles, thus overcoming the initial protection of the G6PD deficient
condition. However, they maintain that in the heterozygote this process of adap-
tation will not take place as the parasites emerging from the G6PD normal eryth-
rocytes will face an approximately 50% probability of entering a G6PD deficient
erythrocyte to which they are not adapted and they imply that this should limit the
parasitization. In another publication derived from this work Luzzatto et al. (1986)
state that "However, in heterozygous females parasite adaptation will be persis-
tently frustrated by the co-existence of G6PD normal and G6PD deficient red cells.
. . As a result, the susceptibility to malaria mortality of the Gd + /Gd-genotype is
These are interesting findings which suggest that there is some degree of adap-
tation on the part of the P. falciparum parasite to the G6PD deficient host envi-
YEARBOOK OF PHYSICAL ANTHROPOLOGY
[Vol. 36, 1993
ronment; however, such an observation in itself does not prove that "hemizygous
G6PD-deficient males are not protected against infection" (Usanga and Luzzatto,
1985:795), which has been the conclusion of their own field studies (Bienzle et al.,
1972; Luzzatto and Bienzle, 1979; Guggenmoos-Holzmann et al., 1981).
There are several objections to these findings. First, Usanga and Luzzatto (1985:
794) report that parasites adapted through four cycles of G6PD (–) cells, when "
measured in a fifth cycle in G6PD-deficient (A–) RBC, was 79 ± 5% of the
multiplication observed on transfer back to G6PD-normal RBC." Although this
decrease in parasitic growth rate is not as dramatic as occurs in P. falciparum that
have not been adapted to G6PD (–) erythrocytes, it is still substantial and it can be
argued that such a reduction is likely to slow the rate of development of the
parasitemia to a biologically significant degree. Second, these studies should be
carried out over a much larger number of parasite cycles in order to develop a
better idea of the course of parasitization in G6PD deficient and G6PD normal
erythrocytes. Third, it is difficult to see how the G6PD deficient heterozygote
condition can be the most protective against falciparum multiplication. Approxi-
mately half of the erythrocytes in the heterozygote have normal G6PD activity and
Luzzatto's own work has demonstrated that these erythrocytes have parasitization
rates of two to 80 times those of the G6PD deficient erythrocytes in the same
individuals under natural conditions (Luzzatto et al., 1969). Nothing is protecting
these G6PD ( + ) red cells from parasitization, and if parasites that have adapted to
the G6PD deficient condition then enter the G6PD normal red cells, there should
be no impediment to their development.
In heterozygotes, parasites entering G6PD (–) cells after having cycled through
G6PD ( + ) cells would have a growth rate of about 45% of the rate they would have
had in G6PD ( + ) red cells (Usanga and Luzzatto 1985). However, this moderate
diminution in growth in approximately 50% of the erythrocytes in heterozygous
individuals should not necessarily convey greater antimalarial protection than a
20% diminution in parasite growth rate in all erythrocytes among parasites that
have adapted to G6PD (–) red cells in hemizygous and homozygous individuals.
Further, if dietary oxidants potentiate the protective effect of G6PD (–) geno-
types against falciparum parasitization (see below), then a far larger proportion of
the erythrocytes will benefit from this phenomenon in G6PD deficient hemizygotes
and homozygotes than in the heterozygotes. Therefore, the evidence provided by
Luzzatto and co-workers in these in vitro studies to support the findings of their
single field investigation (Bienzle et al., 1972) is not convincing. It does not support
the view that there is only relative antimalarial protection in G6PD deficient
heterozygotes and no protection against falciparum parasitization in hemizygous
and homozygous G6PD deficient individuals.
Roth and Schulman (1988)
Roth and Schulman (1988) further evaluated adaptation of P. falciparum para-
sites to G6PD deficient erythrocytes. They noted that it had been demonstrated
that P. falciparum produced its own G6PD enzyme after several cycles of growth in
G6PD (–) erythrocytes. In several experiments they investigated whether this
parasite enzyme can enable the G6PD deficient host cell to resist oxidant stress as
well as G6PD normal cells resist such stress. In these experiments they cultured P.
falciparum in G6PD normal erythrocytes, G6PD deficient erythrocytes for one
growth cycle only, and G6PD deficient erythrocytes for a minimum of five growth
cycles. (G6PD deficient erythrocytes were G6PD Mediterranean with less than 1%
of normal G6PD activity and G6PD A (–) with 12 to 15% activity.) All cultures were
then challenged with acetylphenylhydrazine (APH), a powerful oxidant.
It was found that the unadapted malarial parasites added to the G6PD Medi-
terranean red cell medium showed very poor growth for 4 days (two cycles) after
which growth approximated that of parasites in G6PD normal cells. However,
falciparum parasites adapted through growth in G6PD Mediterranean red cells for
five growth cycles and then added to a G6PD Mediterranean red cell medium
G6PD DEFICIENCY AND FALCIPARUM MALARIA
showed near normal growth. These findings replicated the work of Usanga and
Luzzatto (1985) demonstrating the ability of P. falciparum to adapt to G6PD defi-
cient erythrocytes after several cycles.
The investigators then evaluated the sensitivity of the three different parasite-red
cell systems to the inhibitory effects of the oxidant stress of APH over a dose range
of 10 to 500 µg/ml. These experiments demonstrated that the unadapted parasites
in G6PD Mediterranean erythrocytes were eight times more susceptible to the
inhibitory effects of APH than parasites in G6PD normal systems. Compared to this
baseline, adapted parasites in G6PD Mediterranean red cells were four times more
sensitive to APH than parasites in G6PD normal erythrocytes. The parasite G6PD
observed by Usanga and Luzzatto (1985) in P. falciparum adapted to G6PD (–) cells
increased the ability of the parasites to multiply at near-normal rates in G6PD
Mediterranean red cells that were not under external oxidant stress. However, the
adapted parasites in G6PD Mediterranean cell systems were still four times more
vulnerable to additional oxidant stress than were parasites in G6PD normal cell
The results with parasites grown in G6PD A (–) cells were somewhat different.
Unadapted parasites began to grow without a lag period but growth was signifi-
cantly reduced for the first two growth cycles, while parasites adapted to grow in
G6PD A (–) red cells grew at a rate equivalent to those in G6PD normal eryth-
rocytes. When APH was applied to parasite-G6PD A (–) cell systems the results
were markedly different from those in the G6PD Mediterranean system. Both
unadapted and adapted parasite-G6PD A (–) systems showed only slightly greater
parasite sensitivity to the inhibitory effects of APH compared to parasite-G6PD
normal cell systems.
It seems clear from these data that adaptation of P. falciparum to G6PD Medi-
terranean red cells is minimal, as the parasites in these parasite-red cell systems
were still four times more sensitive to oxidant stress than parasites in control
parasite-G6PD ( + ) red cell systems. However, both unadapted and adapted para-
sites in G6PD A (–) erythrocytes were only about 30 to 40% more vulnerable to an
oxidant stress than were parasites in G6PD normal cells. Although this greater
sensitivity to oxidant stress is modest and was not statistically significant in this
study, it is still probably biologically significant in protecting G6PD A (–) eryth-
rocytes against falciparum parasitization.
IN VITRO STUDIES OF THE EFFECT OF OXIDANT STRESS FROM NATURALLY
OCCURRING ANTIMALARIAL SUBSTANCES OF PLANT ORIGIN ON PLASMODIUM
GROWTH IN HUMAN ERYTHROCYTES
The chemistry of favism-inducing compounds
While parasitologists were carrying out in vitro investigations of the possible
protective effects of G6PD deficient erythrocytes against P. falciparum infection (see
above), several nutritional biochemistry groups were exploring the nature of the
hemolysis-producing compounds in fava beans (Beutler, 1970; Chevion et al.,
1982, 1983; Jamalian, 1978; Jamalian et al., 1977; Higazi and Read, 1974). Since
prophylactic administration of aminoquinoline antimalarials had been identified as
the cause of hemolysis in G6PD A (–) subjects in early studies (Beutler et al., 1955;
Beutler, 1957; Carson et al., 1956), the chemical nature of the favism-induc-ing
compounds was of interest. This was not only from a public health point of view,
but also in terms of whether these compounds might provide antimalarial
protection. This would be particularly important for an understanding of how
natural selection operates at the G6PD locus; if these compounds inhibit falci-
parum development, it would be essential to ascertain whether they act differen-
tially on the various G6PD genotypes to produce variation in vulnerability to
falciparum. parasitization among the different G6PD genotypes in human popula-
A number of studies subsequently identified the source of the favism-producing
compounds as the B-glycosides vicine and convicine, which constitute approxi-
YEARBOOK OF PHYSICAL ANTHROPOLOGY
Vol. 36, 1993
mately 0.5% of the wet weight of the Vicia faba seed. These compounds are hy-
drolyzed in the gastrointestinal tract to form their unstable pyrimidine aglycones
divicine and isouramil (Chevion et al., 1982; Chevion et al., 1983; Marquardt,
1989). The oxidized forms of these compounds have been shown to lower red cell
reduced glutathione, and G6PD deficient erythrocytes, with their limited ability to
regenerate reduced glutathione, hemolyze readily in the presence of these sub-
stances (Mager et al., 1965; Clark et al., 1989). These aglycones also generate
hydrogen peroxide and free radical species (Chevion et al., 1982; Winterbourn et
It was thus clear from the chemical characterization of these substances that
they place an oxidant stress on the red blood cell that is similar to that of the
aminoquinoline antimalarials and that these compounds may have antimalarial
effects (Etkin, 1979; Katz and Schall, 1979, 1986; Friedman, 1979).
Studies evaluating the antimalarial effects of divicine and isouramil
Golenser et al. (1983). Golenser et al. (1983) carried out a series of studies on
erythrocytes from a G6PD Mediterranean hemizygous male (<2% normal activ-ity),
two G6PD Mediterranean heterozygous females (40% and 50% normal activ-ity),
and two G6PD normal males. In each experiment there were two treatments of the
erythrocytes from each subject: 1) they were bathed in a control solution; or 2) they
were bathed in a solution of isouramil. All of the erythrocyte treatment conditions
were then inoculated with the P. falciparum cultures.
The development of P. falciparum in the G6PD normal erythrocytes was not
affected by the pretreatment of the red cells with isouramil. In both the control and
the isouramil treated erythrocytes, 10% of the red cells were parasitized by the
third day. Parasitemias reached similar levels in the G6PD deficient red cells that
had been bathed in control solution, but in contrast, parasite growth was totally
unsupported in the G6PD deficient erythrocytes that had been bathed in isouramil.
When evaluated as a function of the G6PD activity of the host erythrocytes,
isouramil pretreatment totally suppressed parasite development in the G6PD
hemizygous deficient (<2% activity) suspension, had an intermediate effect in the
heterozygous suspensions (40 to 50% activity), and only had a very minor effect in
the G6PD normal red cells. Young parasite stages (ring forms) were much less
sensitive to the effect of isouramil than trophozoites and schizonts. The investiga-
tors concluded that G6PD normal individuals may be partially protected and G6PD
deficient genotypes more fully protected against falciparum malaria. As a
consequence of the ingestion of isouramil in fava beans.
Golenser et al. (1988). In this series of experiments Golenser et al. (1988) again
examined P. falciparum parasite development in cultures of untreated G6PD nor-
mal and G6PD Mediterranean erythrocytes. They also studied parasite develop-
ment in cultures of G6PD normal and G6PD Mediterranean parasitized erythro-
cytes that were exposed to crisis form factor (CFF; a normal blood constituent
associated with the development of immunity to malaria), isouramil, and diamide.
In the first set of experiments with untreated G6PD Mediterranean erythrocytes
they found that the total number of parasites in the deficient red cells was not
lower than in normal erythrocytes. However, on closer examination they noted a
profound retardation in the stage distribution of parasites in G6PD deficient red
cells—with a predominance of less mature forms in the G6PD deficient red cells at
comparable times in the parasitemia compared to the normal erythrocytes.
When parasites in the G6PD deficient and G6PD normal erythrocytes were
exposed to CFF, the P. falciparum within the G6PD deficient erythrocytes were
significantly more sensitive to the inhibitory activity of CFF, with a high percent-
age of ring forms failing to develop within these erythrocytes.
G6PD DEFICIENCY AND FALCIPARUM MALARIA
Exposure of parasitized G6PD deficient erythrocytes to the oxidant isouramil,
derived from fava beans, and also to diamide, a thiol oxidizing agent, did not have
a greater effect on parasite morphology than in parasitized G6PD normal eryth-
rocytes. However, measures of parasite incorporation of [3H]-isoleucine and [ H]-
hypoxanthine showed that mature parasites in G6PD normal erythrocytes were
more sensitive to the oxidant effect of isouramil than were the ring forms. Further, in
G6PD deficient erythrocytes both ring forms and mature stages were more
sensitive to oxidant stress than the corresponding stages in G6PD normal eryth-
In summary, taken together these two studies (Golenser et al., 1983, 1988)
indicate that G6PD Mediterranean erythrocytes are resistant to falciparum para-
sitization on their own and that this resistance is greatly potentiated by exposure
to CFF and isouramil, a dietary oxidant derived from fava beans. In addition,
isouramil also had a modest suppressant effect on mature parasite forms in G6PD
Clark et al. (1984). Clark et al. (1984) studied the effect of intravenous adminis-
tration of divicine into mice that had been infected with Plasmodium vinckei, a
mouse malarial parasite. Six days after infection with P. vinckei, after para-
sitemias were between 30 and 60%, the mice (n = 22) received an intravenous
injection of divicine. By 1 hour after intravenous injection of the divicine micro-
scopic examination indicated that 38% of the parasites had degenerated within the
circulating erythrocytes. Further, the micrographs indicated that parasite mem-
branes were damaged while the host erythrocyte membranes were intact. At 1
hour, the total parasitemia, counting intact and damaged parasites, had fallen by
25%, and 45% of the parasites observed by light microscope had shrunken with
fragmented and pyknotic nuclei. Within 2 hours of injecting divicine the urine of
the mice had changed to a dark black color and hemolysis was confirmed by he-
matocrit and plasma hemoglobin values, while unparasitized mice did not hemo-
lyze under these conditions. Within 2 hours of the divicine injection parasite levels
had fallen by approximately 65%, and the parasitemia in the mice was usually
cleared by a single dose of divicine.
Intraperitoneal desferrioxamine (an iron chelator) blocked the antimalarial ac-
tion of the divicine, while iron-saturated desferrioxamine did not limit the anti-
malarial effect. These data support the view that iron-catalyzed free radical pro-
duction from divicine was responsible for the hemolysis and for the direct parasite
killing effect.
These data provide strong evidence indicating that divicine has a powerful an-
timalarial effect in vivo. Although a mouse model with a P. vinckei parasitemia may
be somewhat different than human falciparum malaria, the differences are likely to
be minor. Also, human consumption of fava beans and other foods con-taining
naturally occurring antimalarial substances is likely to result in a more gradual
dose than that achieved with a massive intravenous injection. Therefore, these
experimental findings probably differ somewhat from the manner in which these
substances function in natural circumstances in human populations. Never-theless,
it is particularly noteworthy that this fava bean-derived substance pro-duced an
antimalarial effect in presumably G6PD normal mice, although, again, probably at
doses greater than occur under natural circumstances in human pop-ulations.
These data suggest that if divicine has antimalarial activity in vivo in G6PD normal
erythrocytes, which are well-buffered against oxidant stress, then it is likely to do
the same at much lower doses in G6PD deficient individuals, as the G6PD (–)
erythrocytes are much more sensitive to the oxidant stress created by this
YEARBOOK OF PHYSICAL ANTHROPOLOGY
CO-EVOLUTION OF THE G6PD POLYMORPHISM AND QUININE TASTE SENSITIVITY:
As noted above, there is strong evidence that G6PD deficient erythrocytes
achieve optimal antimalarial protection when they are placed under an additional
oxidant stress due to the consumption of oxidant dietary substances such as divi-
cine and isouramil in fava beans (Golenser et al., 1983, 1988; Clark et al., 1984,
1989), and probably from other oxidants in a variety of food crops (Etkin, 1979,
1986; Xiao, 1981). However, if this oxidant stress is excessive, G6PD deficient
individuals, especially those with the severely deficient Class II enzyme variants
like the Mediterranean variant, are at risk to experience an acute hemolytic epi-
sode which may be life-threatening (Beutler, 1983; Luzzatto and Mehta, 1989;
Kattamis, 1986; Arese et al., 1986; De Flora et al., 1986; Belsey, 1973). Therefore, on
theoretical grounds, human population adaptation would be enhanced if there
were some mechanism whereby the dietary intake of these naturally occurring
antimalarial substances was regulated so that dietary intake was adequate for
antimalarial protection, but not so excessive as to produce hemolysis in G6PD
deficient individuals. Adaptation would be further enhanced if dietary intake of
these substances were maximized in G6PD normal individuals so as to provide
optimal antimalarial benefits.
We have suggested that another genetic trait called "quinine" taste sensitivity (
Fischer et al., 1961; Fischer, 1967; Smith and Davies, 1973) actually functions to
regulate the intake of bitter-tasting naturally occurring antimalarial substances of
plant origin, with sensitive tasters of quinine consuming more limited quanti-ties
of these compounds and individuals who are less sensitive tasters of quinine
consuming greater amounts, or more concentrated doses, of these dietary sub-
stances (Greene et al., 1993). Further, although the genetic locus of quinine taste
sensitivity is not known, we have postulated that it is on the X-chromosome and
that the G6PD and "quinine" taste loci are closely linked, producing four possible
haplotypes in the hemizygous individual: G6PD-/Q+; G6PD-/Q-; G6PD +/Q+ ; G6PD+/Q-. We argue that there has been co-evolution of the G6PD polymorphism and "
quinine" taste sensitivity in populations living in areas where falciparum malaria
has been endemic and where naturally occurring antimalarial substances of plant
origin are consumed in the diet, in that there is a relative increase in the G6PD-
We illustrate our argument by using the hemizygous deficient condition as an
example and Q to denote acute taste sensitivity to quinine and Q- to denote an
insensitivity to the taste of quinine. In our convention the Q does not represent a
single allele, but a polygenically acting group of alleles on the X-chromosome. The
alleles act additively, resulting in codominance. We suggest that the G6PD-/Q+
haplotype will have greater fitness than the G6PD-/Q- haplotype in areas where
falciparum malaria is endemic and bitter-tasting oxidants are consumed in the
diet, because of a propensity to avoid or limit the intake of these substances. The
two haplotypes have equivalent antimalarial protection from their G6PD deficient
condition, but the G6PD-/Q+ individuals should have a diminished hemolytic risk if
they modulate their intake of these bitter-tasting, oxidant-containing foods. In a
similar fashion, we suggest that the G6PD+/Q- haplotype would have greater
fitness than the G6PD +/Q+ haplotype. Due to their taste insensitivity, there is a
greater likelihood that individuals with this haplotype (who do not have the bio-
logic protection against falciparum malaria of the G6PD deficient condition) would
consume more bitter-tasting dietary oxidants and thus would have the continuing
antimalarial benefits of this diet-mediated prophylaxis (see also Greene, 1974).
This hypothesis of a co-evolution of the G6PD polymorphism and "quinine" taste
sensitivity was tested in a preliminary study of 17 G6PD deficient and 25 G6PD
normal African-American subjects 15 to 40 years of age (Greene et al., 1993). The
G6PD deficient subjects showed greater taste sensitivity to quinine sulfate, but not
to sodium chloride (a control comparison) than did the G6PD normal subjects.
G6PD DEFICIENCY AND FALCIPARUM MALARIA
However, due to the small sample size these differences were not significant (
ANOVA, P < .10). This study was limited by our inability to locate and recruit the
proposed number of G6PD deficient subjects. Based on our data on G6PD activity
in this population, a fair test of the hypothesis would require a sample of approx-
imately 75 G6PD deficient and 75 G6PD normal subjects.
Although this hypothesis has not yet received empirical support, we believe that it
is testable. If confirmed, it would significantly enhance the biocultural perspec-tive
presented above.
Fava bean consumption is widespread in the circum-Mediterranean region, the
Near and Middle East, and southwest Asia, and malaria is common in all of these
areas (Katz and Schall, 1986). Malaria is also common in the southern portion of
China, the largest producer of fava beans in the world (Mikhael, 1986). However,
the fava bean is only the best studied food crop with clear antimalarial activity.
Etkin (1979, 1986) has provided evidence indicating that a number of plants used
in traditional African medicine and diet have antimalarial activity. Xiao (1981) has
provided similar evidence for several plants used in China. Therefore, it ap-pears
that in human populations there is widespread dietary use of plants with clear or
suspected antimalarial activity.
SUMMARY AND CONCLUSIONS
This article has presented support for the view that all G6PD deficient genotypes
are relatively protected against falciparum malaria, and that this protective effect is
potentiated by the consumption of naturally occurring antimalarial substances of
plant origin. Fava beans are the best known and best described source of these
dietary oxidants, but not the only source. It also presents an hypothesis and a
summary of preliminary data suggesting that what has been called "quinine" taste
sensitivity functions to regulate the dietary intake of these substances and that
this trait has co-evolved with the G6PD polymorphism in human populations.
Although it appears clear that the G6PD deficient genotypes are relatively pro-
tected against falciparum and perhaps vivax malaria, it is also certain that there is
strong selection against the hemizygous and homozygous G6PD deficient geno-
types as a result of diet-, drug-, chemical-, and infection-related hemolysis and
neonatal jaundice (Beutler, 1983; Belsey, 1973; Luzzatto and Mehta, 1989; Arese et
al., 1986; Chevion et al., 1982; Calabrese, 1984). It is thus likely that the heterozy-
gous G6PD deficient female is the most fit genotype in areas where malaria is
endemic. Further, the G6PD-/Q+ G6PD /Q- heterozygote should be the most fit
genetic constitution in regions where malaria is endemic. These individuals would
benefit from a moderate intake of dietary antimalarials due to their intermediate
quinine taste sensitivity, and would have a limited risk of experiencing hemolysis.
Since G6PD occurs in all cells of the body (Luzzatto and Battistuzzi, 1985; Luz-
zatto and Mehta, 1989), G6PD deficiency may also be involved in cellular defense
against other parasitic diseases and infections. This would be one possible expla-
nation of why alleles for G6PD deficiency are at polymorphic frequencies in some
populations in areas where malaria has apparently not been endemic (Livingstone,
1985). The plethora of G6PD deficient variants that have reached polymorphic
frequencies also suggests that there may be unique relationships between each
variant and particular dietary oxidants in regional cuisines, which would be an
important area of future investigation.
Part of this article was written during the 1991-92 academic year when the
author was a visiting scholar at the University of Cambridge. I would like to thank
Dr. C. G. N. Mascie-Taylor, Head, Department of Anthropology, University of
Cambridge, Professor A. N. Broers, Master of Churchill College, and the Fellows of
Churchill College for their kind hospitality during that period of time.
YEARBOOK OF PHYSICAL ANTHROPOLOGY
Allison AC (1957) Malaria in carriers of sickle-cell
(1956) Enzymatic deficiency in primaquine sen-
trait and in newborn children. Exp. Parasitol. 6:
sitive erythrocytes. Science 124:484.
Chevion M, Navok T, Glaser G, and Mager J (
Allison AC and Clyde DF (1961) Malaria in Afri-
1982) The chemistry of favism-inducing com-
can children with deficient erythrocyte glucose-
pounds. Eur. J. Biochem. 127:405–409.
6-phosphate dehydrogenase. Br. Med. J. 1.1346–
Chevion M, Mager J, and Glaser G (1983) Natu-
rally occurring food toxicants: Favism-producing
Arese P, Mannuzzu L, Turrini F, Galiano S, and
agents. In M Rechcigl Jr (ed.): Handbook of Nat-
Gaetani GF (1986) Etiological aspects of favism.
urally Occurring Food Toxicants. Boca Raton, FL:
In A Yoshida and E Beutler (eds.): Glucose-6-
CRC Press, pp. 63–79.
Phosphate Dehydrogenase. Orlando, FL: Aca-
Clark IA, Cowden WB, Hunt NH, Maxwell LE,
demic Press, pp. 45–75.
and Mackie EJ (1984) Activity of divicine in Plas-
Belsey MA (1973) The epidemiology of favism.
modium vinckei–infected mice has implications
Bull. WHO 48:1–13.
for treatment of favism and epidemiology of G-6-
Betke K, Brewer GJ, Kirkman HN, Luzzatto L,
PD deficiency. Br. J. Haematol. 57:479–487.
Motulsky AC, Ramot B, and Siniscalco M (1967)
Clark IA, Chaudhri G, and Cowden WB (1989)
Standardization of procedures for the study of
Some roles of free radicals in malaria. Free Radic.
glucose-6-phosphate
Biol. Med. 6:315–321.
Health Organization Technical Report Series No.
De Flora A, Benatti U, Guida L, and Zocchi E (
366. Geneva: WHO.
1986) Divicine and G6PD-deficient erythrocytes:
Beutler E (1957) The glutathione instability of
An integrated model of cytotoxicity in favism. In
drug sensitive red cells: A new method for the in
A Yoshida and E Beutler (eds.): Glucose-6-Phos-
vitro detection of drug sensitivity. J. Lab. Clin.
phate Dehydrogenase. Orlando, FL: Academic
Press, pp. 77–93.
Beutler E (1970) L-dopa and favism. Blood 36:
Eckman JR and Eaton JW (1979) Dependence of
plasmodial glutathione metabolism on the host
Beutler E (1983) Glucose-6-phosphate dehydroge-
cell. Nature 278:754–756.
nase deficiency. In JB Stanbury, J Wyngaarden,
Etkin NL (1979) Indigenous medicine among the
DS Fredrickson, and J Goldstein (eds.): The Met-
Hausa of northern Nigeria: Laboratory evalua-
abolic Basis of Inherited Disease. Fifth edition.
tion for potential therapeutic efficiency of anti-
New York: McGraw-Hill, pp. 1629-1653.
malarial plant medicinals. Med. Anthropol. 3:
Beutler E (1990) The genetics of glucose-6-phos-
phate dehydrogenase deficiency. Semin. Hema-
Etkin NL (1986) Multidisciplinary perspectives in
tol. 27:137–164.
the interpretation of plants used in indigenous
Beutler E (1991) Glucose-6-phosphate dehydroge-
medicine and diet. In NL Etkin (ed.): Plants in
nase deficiency. New Engl. J. Med. 324:169-174.
Indigenous Medicine and Diet. Bedford Hills, NY:
Beutler E (1992) The molecular biology of G6PD
Redgrave Press, pp. 2–29.
variants and other red cell enzyme defects. Annu.
Fischer R (1967) Genetics and gustatory chemore-
Rev. Med. 43:47–59.
ception in man and other primates. In MR Kare
Beutler E, Dern RJ, and Alving AS (1955) The
and 0 Maller (eds.): The Chemical Senses and
hemolytic effect of primaquine. VI. An in vitro
Nutrition. Baltimore: Johns Hopkins University
test for sensitivity of erythrocytes to primaquine.
J. Lab. Clin. Med. 45:40.
Fischer R, Griffin F, England S, and Garn SM (
Beutler E, Kuhl W, Vives-Corrons JL, and Prehal
1961) Taste thresholds and food dislikes. Nature
JT (1989) Molecular heterogeneity of glucose-6-
phosphate dehydrogenase A-. Blood 74:2550–
Friedman MJ (1979) Oxidant damage mediates
variant red cell resistance to malaria. Nature
Bienzle U, Ayeni 0, Lucas A, and Luzzatto L (
1972) Glucose-6-phosphate dehydrogenase and
Gilles NH, Hendrickse RG, Linder R, Reddy S, and
malaria. Lancet 1:107–110.
Allan N (1967) Glucose-6-phosphate dehydroge-
Bienzle U, Effiong CE, Aimaku VE, and Luzzatto
nase deficiency, sickling, and malaria in African
L (1976) Erythrocyte enzymes in neonatal jaun-
children in southwestern Nigeria. Lancet 1:138–
dice. Acta Haematol. (Basel) 55:10–20.
Bienzle U, Guggenmoos-Holzmann I, and Luzzatto
Gloria-Bottini F, Falsi AM, Mortera J, and Bottini
L (1979) Malaria and erythrocyte glucose-6-phos-
E (1980) The relations between G-6-PD,
phate dehydrogenase variants in West Africa.
thalassemia and malaria. Further analysis of
Am. J. Trop. Med. Hyg. 28:619–621.
data from Sardinia and the Po valley. Experien-
Brown IN (1969) Immunological aspects of ma-
tia 36:541-543.
laria infection. Adv. Immunol. 11:267–349.
Golenser J, Miller J, Spira DT, Navok T, and
Brown PJ (1981) New considerations on the distri
Chevion M (1983) Inhibitory effect of a fava bean
-bution of malaria, thalassemia, and glucose-6-
component in the in vitro development of Plas-
phosphate dehydrogenase deficiency in Sardinia.
modium falciparum in normal and glucose-6-
Hum. Biol. 53:367–382.
phosphate deficient erythrocytes. Blood 61:507–
Butler T (1973) G-6-PD deficiency and malaria in
black Americans in Vietnam. Milit. Med. 138:
Golenser J, Miller J, Spira DT, Kosower NS,
Vande waa JA, and Jensen JB (1988) Inhibition
Calabrese EJ (1984) Ecogenetics. New York: John
of the intraerythrocytic development of Plasmo-
Wiley & Sons, pp. 17–39.
dium falciparum in glucose-6-phosphate deficient
G6PD DEFICIENCY AND FALCIPARUM MALARIA
erythrocytes is enhanced by oxidants and by cri-
tions of sickle cell gene distribution in West Af-
sis form factor. Trop. Med. Parasitol. 39:272-276.
rica. Am. Anthropol. 60:533-562.
Greene LS (1974) Physical growth and develop-
Livingstone FB (1984) The Duffy blood groups,
ment, neurological maturation and behavioral
vivax malaria, and malaria selection in human
functioning in two Ecuadorean Andean commu-
populations. A review. Hum. Biol. 56:413-425.
nities where goiter is endemic. II. PTC taste sen-
Livingstone FB (1985) Frequencies of Hemoglobin
sitivity and neurological maturation. Am. J.
Variants. Oxford: Oxford University Press.
Phys. Anthropol. 41:139-151.
Luzzatto L and Battistuzzi G (1985) Glucose-6-
Greene LS, McMahon L, and dilorio J (1993) Co-
phosphate dehydrogenase. Adv. Med. Genet. 14:
evolution of glucose-6-phosphate dehydrogenase
deficiency and quinine taste sensitivity. Ann.
Luzzatto L and Bienzle U (1979) The malaria/G.-
Hum. Biol. 20:497-500.
6-P.D. hypothesis. Lancet 1:1183-1184.
Guggenmoos-Holzmann I, Bienzle U, and Luzzatto
Luzzatto L and Mehta A (1989) Glucose-6-phos-
L (1981) Plasmodium falciparum malaria and
phate dehydrogenase deficiency. In CR Scriver,
human red cells. II. Red cell genetic traits and
AL Baudet, WS Sly, and D Valle (eds.): The Met-
resistance against malaria. Int. J. Epidemiol. 10:
abolic of Inherited Disease. Sixth edition. New
York: McGraw-Hill, pp. 2237-2265.
Higazi ML and Read WW (1974) Methods for de-
Luzzatto L and Testa U (1978) Human erythrocyte
termination of vicine in plant material and blood.
glucose-6-phosphate dehydrogenase: Structure
J. Agric. Food Chem. 22:570.
and function in normal and mutant subjects.
Jamalian J (1978) Favism-inducing toxins in
Curr. Top. Hematol. 1:1-70.
broad beans (Vicia faba). Determination of the
Luzzatto L, Usanga E, and Reddy S (1969) Glu-
vicine content and the investigation of other non-
cose-6-phosphate dehydrogenase deficient red
protein nitrogenous compounds in different broad
cells: Resistance to infection by malarial para-
bean cultivars. J. Sci. Food Agric. 29:136-140.
sites. Science 164:839-841.
Jamalain J, Aylward F, and Hudson B (1977)
Luzzatto L, Sodeinde 0, and Martini G (1983) Ge-
Favism-inducing toxins in broad bean extracts in
netic variation in the host and adaptive phenom-
favism sensitive subjects. Plant Foods for Human
ena in Plasmodium falciparum infection. In
Malaria and the Red Cell. Ciba Foundation Sym-
Jarra W (1983) Protective immunity to malaria
posium No. 94. London: Pitman, pp. 159-173.
and antierythrocytic autoimmunity. In Malaria
Luzzatto L, O'Brien S, Usanga E, and Wanachi-
and the Red Cell. Ciba Foundation Symposium
wanawin W (1986) Origin of G6PD polymor-
No. 94. London: Pitman, pp. 137-158.
phism: Malaria and G6PD deficiency. In A
Johnson GJ, Allan DW, and Flynn TP (1986) Ox-
Yoshida and E Beutler (eds.): Glucose-6-Phos-
idant-induced membrane damage in G-6-PD de-
phate Dehydrogenase. Orlando, FL: Academic
ficient red blood cells. In A Yoshida and E Beutler
Press, pp. 181-193.
(eds.): Glucose-6-Phosphate Dehydrogenase. Or-
Mager J, Glaser G, Razin A, Izak G, Bien S, and
lando, FL: Academic Press, pp. 153-177.
Noam J (1965) Metabolic effects of pyrimidines
Kar S, Seth S, and Seth PK (1992) Prevalence of
derived from fava bean glycosides on human
malaria in Ao Nagas and its association with
erythrocytes deficient in glucose-6-phosphate de-
G6PD and HbE. Hum. Biol. 64:187-197.
hydrogenase. Biochem. Biophys. Res. Commun.
Kattamis C (1986) Favism: Epidemiological and
clinical aspects. In A Yoshida and E Beutler (eds.
Marquardt RR (1989) Vicine, convicine, and their
): Glucose-6-Phosphate Dehydrogenase. Or-
aglycones—divicine and isouramil. In PR Cheeke (
lando, FL: Academic Press, pp. 25-43.
ed.): Toxicants of Plant Origin. Volume 2. Boca
Katz SH and Schall J (1979) Fava bean consump-
Raton, FL: CRC Press, pp. 161-200.
tion and biocultural evolution. Med. Anthropol.
Martin SK, Miller LH, Alling D, Okoye VC, Esan
GJF, Osunkoya BO, and Deane M (1979) Severe
Katz SH and Schall J (1986) Favism and malaria:
malaria and glucose-6-phosphate dehydrogenase
A model of nutrition and biocultual evolution. In
deficiency: A reappraisal of the malaria/G.-6-P. D.
N Etkin (ed.): Plants in Indigenous Medicine and
hypothesis. Lancet 1:524-526.
Diet. Bedford Hills, NY: Redgrave Press, pp. 211-
Meijer A (1984) Psychiatric problems of children
with glucose-6-phosphate dehydrogenase defi-
Kidson C and Gorman JG (1962) A challenge to
ciency. Int. J. Psychiat. Med. 14:207-214.
the concept of selection by malaria in glucose-6-
Mikhael NA (1986) Toxic Substances in Local Va-
phosphate dehydrogenase deficiency. Nature
rieties of Faba Beans. Ph.D. Dissertation in
Chemistry, University of Cairo, Cairo, Egypt.
Kirkman HN and Gaetani GF (1986) Regulation of
Miller LH (1985) Malaria. In JB Wyngaarden and
glucose-6-phosphate dehydrogenase in normal
LH Smith, Jr. (eds.): Cecil Textbook of Medicine.
and variant red blood cells. In A Yoshida and E
17th edition. Philadelphia: W. B. Saunders, pp.
Beutler (eds.): Glucose-6-Phosphate Dehydroge-
nase. Orlando, FL: Academic Press, pp. 109-132.
Newsholme EA and Leech AR (1983) Biochemistry
Kosower NS and Kosower EM (1970) Molecular
for the Medical Sciences. New York: John Wiley &
basis for selective advantage of glucose-6-phos-
phate dehydrogenase deficient individuals ex-
Piomelli S (1986) G6PD-related neonatal jaundice.
posed to malaria. Lancet 2:1343-1344.
In A Yoshida and E Beutler (eds.): Glucose-6-
Kruatrachue M, Charoenlarp P, Chongsopha-
Phosphate Dehydrogenase. Orlando, FL: Aca-
jaisiddhi T, and Harinasuta C (1962) Erythrocyte
demic Press, pp. 95-108.
glucose-6-phosphate dehydrogenase and malaria
Piomelli S, Carash LM, Davenport DD, Miraglia J,
in Thailand. Lancet 2:1183-1186.
and Amorosi EL (1968) In vivo lability of G6PD
YEARBOOK OF PHYSICAL ANTHROPOLOGY
in GdA- and GdMed'terraRed" deficiency. J. Clin. In-
tion of parasite-encoded enzyme. Nature 313:
vest. 47:940.
Powell RD and Brewer GJ (1965) Glucose-6-phos-
Valaes T, Karaklis A, and Doxiadis S (1985) Gene
phate dehydrogenase deficiency and falciparum
loss due to neonatal jaundice in glucose-6-phos-
malaria. Am. J. Trop. Med. Hyg. 14:358-362.
phate dehydrogenase deficiency. In L Stern, M
Roth E and Schulman S (1988) The adaptation of
Xanthou, and B Friis-Hansen (eds.): Physiologic
Plasmodium falciparum to oxidative stress in
Foundations of Perinatal Care. New York: Prae-
G6PD deficient human erythrocytes. Br. J. Hae-
matol. 70:363-367.
Vulliamy TJ, D'Urso M, Battistuzzi G, Estrada M,
Roth EF, Raventos-Suarez C, Rinaldi A, and Nagel
Foulkes NS, Martini G, Calabro V, Poggi V, Gior-
RL (1983) Glucose-6-phosphate dehydrogenase
dano R, Town M, Luzzatto L, and Perisco MG (
deficiency inhibits in vitro growth of Plasmodium
1988) Diverse point mutations in the human glu-
falciparum. Proc. Natl. Acad. Sci. USA 80:298-
cose-6-phosphate dehydrogenase gene cause en-
zyme deficiency and mild or severe hemolytic
Singh H (1986) Glucose-6-phosphate dehydroge-
anemia. Proc. Natl. Acad. Sci. USA 85:5171-5175.
nase deficiency. A preventable cause of mental
WHO Working Group (1989) Glucose-6-phosphate
retardation. Br. Med. J. 292:397-398.
dehydrogenase deficiency. Bull. WHO 67:601-
Siniscalco M, Berini L, Filippi G, Latte B, Meera
Khan P, Piomelli S, and Rattazzi M (1966) Pop-
Winterbourn CC, Benatti U, and De Flora A (
ulation genetics of hemoglobin variants, thalas-
1986) Contributions of superoxide, hydrogen
semia and glucose-6-phosphate dehydrogenase
peroxide, and transition metal ions to auto-oxi-
deficiency, with particular reference to the ma-
dation of the favism-inducing pyrimidine agly-
laria hypothesis. Bull. WHO 34:379-393.
cone, divicine, and its reaction with haemoglobin.
Smith SE and Davies PDO (1973) Quinine taste
Biochem. Pharmacol. 35:2009-2015.
thresholds: A family study and a twin study.
Woodruff AW (1978) Malaria. In Sir RB Scott (ed.):
Ann. Hum. Genet. 37:227-232.
Price's Textbook of the Practice of Medicine. 12th
Strickland GT (1991) Malaria. In GT Strickland (
edition. Oxford: Oxford University Press, pp. 178-
ed.): Hunter's Tropical Medicine. Seventh edi-
tion. Philadelphia: W. B. Saunders, pp. 586-615.
Xiao P (1981) Traditional experience of Chinese
Trager W and Jensen JB (1976) Human malaria
herb medicine. Its application in drug research
parasites in continuous culture. Science 193:
and new drug searching. In JL Beal and E Rein-
hard (eds.): Natural Products as Medicinal
Usanga EA and Luzzatto L (1985) Adaptation of
Agents. Stuttgart: Hippokrates Verlag, pp. 351-
Plasmodium falciparum to glucose-6-phosphate
dehydrogenase-deficient host red cells by produc-
Source: http://www.anthropology.ua.edu/bindon/ant475/Readings/r11.pdf
Permitted in or as carry on baggage Permitted in or as checked baggage Permitted on one´s person Approval of Icelandair is required Pilot in Command must be informed of the location Disabling devices such as mace, pepper spray, etc. containing an irritant or incapacitating NO NO NO N/A N/A substance are provided on the person, in checked and carry-on baggage. Electro shock weapons (e.g. Tasers) containing dangerous goods such as explosive,
Journal of Magnetism and Magnetic Materials 225 (2001) 226} 234 Signal transduction of erythrocytes after speci"c binding of ecdysterone and cholesterol immobilized on nanodispersed O.M. Mykhaylyk *, A.V. Kotzuruba, O.M. Buchanevich, A.M. Korduban, E.F. Meged, N.M. Gulaya Institute for Applied Problems in Physics and Biophysics, National Academy of Sciences, Ukraine, P.O. Box 355, 255001 Kyiv, Ukraine