Microsoft word - bidwell final report.doc
Title: Occurrence of Pharmaceuticals, Hormones, and other Organic Wastewater
Contaminants in Cave Water within the Lower Neosho and Illinois River Basins,
Oklahoma
Start Date: 3/1/2006
End Date: 2/28/2007
Congressional District: District 3 (University), District 2 (Field Sites)
Focus Category: GW, NPP, WQL
Descriptors: Organic wastewater contaminants, cave streams, passive sampler
Principal Investigators: Joseph R. Bidwell, Department of Zoology, Oklahoma State
University
Publications: A manuscript that will be submitted to a peer-reviewed publication is
currently in preparation.
Problem and Research Objectives: The headwaters and upper basins of the Illinois
River and the eastern part of the Lower Neosho River are located in northwestern
Arkansas and northeastern Oklahoma. In Arkansas, this area is experiencing significant
urban development and is one of the most productive poultry producing regions in the
United States (Galloway et al. 2004). While there has been widespread concern about
the health and continued aesthetic quality of these systems due to nutrient input and
associated water quality effects, effluent and/or runoff from livestock production facilities
and municipal wastewater treatment plants may contain a number of organic
wastewater contaminants (OWCs) such as antibiotics, hormone residues, various
pharmaceutical compounds, and other trace organics that are ultimately transferred to
aquatic habitats. The frequency with which these contaminants occur in U.S. surface
waters was clearly indicated by a U.S. Geological Survey (USGS) study that found 80%
of the 139 streams sampled contained detectable levels of OWCs (Kolpin et al. 2002).
Another recent USGS study (Galloway et al. 2004) sampled surface water in Benton
and Washington counties, Arkansas, and similarly reported the presence of selected
OWCs in some streams receiving input from municipal wastewater treatment plants.
In addition to the land use characteristics stated above, the basins of the Illinois and
Lower Neosho Rivers are situated within the Ozark Plateau, an uplifted region with a
karst topography that is characterized by sinkholes, disappearing springs, and caves.
As a result of these features, surface water contaminants may enter the groundwater
and associated cave ecosystems. The presence of contaminants in water flowing
through caves is a concern since these habitats often support a highly specialized
assemblage of organisms which may be particularly susceptible to water-quality impacts
due to their generally low population densities and unique life history requirements
(Graening and Brown, 2003). Several aquatic species that are federally-listed or
considered species of special concern are known to use caves in the Ozark Plateau of
Oklahoma. These species include the Ozark cavefish,
Amblyopsis rosae, federally- and
state-listed as threatened, and the Oklahoma cave crayfish,
Cambarus tartraus, state-
listed as endangered. An unnamed cave crayfish,
C. subterraneus, and the Ozark cave
amphipod,
Stygobromus ozarkensis, both considered critically imperiled by the
Oklahoma Natural Heritage Inventory, also occur in caves of this region (NatureServe,
2005). Environmental contamination has been identified as one of the most significant
threats to cave-dwelling fishes (Proudlove, 2001), and chemical and septic system
pollution has been implicated in the loss of both invertebrate and vertebrate taxa from
cave ecosystems (Aley, 1976; Crunkilton, 1984; Simon and Buikema, 1997).
The prevalence and potential impacts of pharmaceuticals, hormones, and other OWCs
in the Ozark cave habitats of Oklahoma are currently unknown. However, given the
agricultural activities, wastewater discharges, and urban development that are occurring
in the Lower Neosho and Illinois River basins, the karstic nature of these basins, and
the recent detection of OWCs in streams within them (Galloway et al. 2004),
contamination of ground water and associated cave habitats may be occurring. The
presence of OWCs and related compounds could have significant implications for the
long-term management of cave habitats since the potential for effects due to exposure
to these contaminants may result in the need to relocate populations of some organisms
and make it necessary to revise recovery plans for those groups listed as threatened or
endangered. By determining the extent to which OWC residues are present in caves,
this project will provide an important first step toward understanding the risk these
chemicals pose to these sensitive habitats.
The objectives of this study were to 1) determine the presence of selected lipid soluble
and water soluble pharmaceuticals, hormones, and other organic wastewater
contaminants at two surface-water sites and in ground water in six caves in
northeastern Oklahoma and western Arkansas using Semi Permeable Membrane
Devices (SPMD) and Polar Organic Chemical Integrated Samplers (POCIS), and 2)
evaluate the potential for sub-lethal effects associated with exposure to water from the
sampling sites through 7-day bioassays with the fathead minnow,
Pimephales
promelas, a standard US EPA test organism.
Methodology: Site selection: Caves were selected in the Illinois and Lower Neosho
River basins with the guidance of Mr. Steve Hensley and Mr. Richard Stark, US Fish
and Wildlife Service, Tulsa Field Office, and were restricted to sites in which populations
of Ozark cavefish,
A. rosae, were known to have occurred. The caves evaluated
included four systems (Twin, Starr, Mgee and Long, and January-Stansbury) in
Delaware County, OK, and two systems (Logan and Cave Springs) in Benton County,
AK. In addition, sampling devices were deployed at one surface water site in Oklahoma
and one in Arkansas. The Oklahoma site (designated OK-Surface) was located in an
unnamed creek near the town of Jay, OK, with the sampler deployed approximately 250
m downstream from the outfall of a municipal wastewater treatment plant. A previous
study indicated a link between water in this stream and that occurring in Star Cave
(Aley, 2005). The Arkansas surface water site was located in Little Osage Creek
(designated AK-Surface) near Osage Mills, AK.
Passive sampler deployment: The cave and surface-water sites were sampled using two types of passive,
in situ, samplers; the polar organic chemical integrative sampler or POCIS and the semi-permeable membrane device or SPMD. The POCIS is designed to sample transient water-soluble (polar or hydrophilic) organic chemicals from aquatic environments (USGS, 2004), while SPMDs passively accumulate transient hydrophobic organic compounds, such as PCBs, PAHs, and organochlorine pesticides (Huff, 2005). Both of these samplers have been successfully used to monitor OWCs in surface and groundwater (Jones-Lepp et al. 2004; Vrana et al. 2005). The POCIS and SPMDs were purchased from Environmental Sampling Technologies (EST), St. Joseph, MO, and were sent to Oklahoma State University under argon gas in sealed metal cans. The sampling membranes were held on stainless steel racks and deployed in stainless steel canisters (also obtained from EST, Figure 1 & 2). Each canister included three POCIS and three SPMD samplers, with a single canister placed at each site. During deployment, the samplers were removed from their original metal cans and placed in the deployment canisters as quickly as possible to minimize air exposure. In the caves, the cages were tied off to some object on the shoreline with a length of nylon rope (Figure 2). At the surface water sites, the racks were affixed to a concrete block with lengths of stainless steel cable and submerged. The time taken to transfer the sampler to the cage and then to submerge the cage in water was recorded during each deployment. A set of six trip blanks consisting of metal cans that contained either one of three POSIS or SPMD membranes were opened and exposed to air during the time the water samplers were being deployed as well. The samplers were left on site for approximately 30 days (exact duration is presented in results). During retrieval, the stainless steel canisters were first moved from the water to the shoreline where they were opened. The racks holding the sampling membranes were removed and placed in the original metal cans. As for deployment, trip blanks for both membrane types were exposed to the air from the time the samplers were first removed from the stainless steel canisters until they were placed in the holding cans and the cans sealed by gently tapping their metal lids into place. The sealed cans were placed on ice as soon as possible and transported back to Oklahoma State University where they were held at -20°C until being shipped overnight to Environmental Sampling Technologies (also on ice) for extraction. The membrane extraction procedures followed standard protocols used by Environmental Sampling Technologies for the POCIS and SPMD. The final extract from each sampling cage was a composite of either the three POCIS or SPMD membranes. Similarly, the replicate trip blanks were composited into one extract. The final combined extracts were transferred to 2 ml amber ampules and the ampules sealed in preparation for analyses. In total, the samples were analyzed for 159 different compounds, including 68 common wastewater organics (USGS Schedule 1433), 33 antibiotic and pharmaceutical compounds and 58 additional organics including a number of halogenated forms (Tables 1-3). The analyses were conducted using liquid chromatography-mass spectroscopy (LC-MS) and gas chromatography-mass spectroscopy (GC-MS) at the USGS National Water Quality Laboratory, Denver, Colorado, under the direction of Dr. Steven Zaugg (analytes listed in Table 1 & 3) and at
the Organic Geochemistry Laboratory, USGS Kansas Water Science Center, Lawrence, Kansas, under the direction of Dr. Michael Meyer (antibiotics listed in Table 2). As indicated in Tables 1-3, some of these analyses were conducted on extracts from both samplers, while others were restricted to a particular membrane type. The methods used for analyses of the OWCs and antibiotics listed in Tables 1 &2 followed established protocols (Zaugg et al. 2001; Alvarez et al. 2005) that allowed detection at the microgram (1 x 10-6g) level. The 58 organic compounds listed in Table 3 were quantified at the nanogram (1 x 10-9g) level by a technique that is currently under development and is not yet published (S. Zaugg, USGS, personal communication). The results of chemical analyses are qualitative (presence/absence) or semi-quantitative (relative concentrations) since calculations of water concentrations based on levels sequestered in the sampling devices requires an
in situ sampling rate for each chemical and this value is not known for all analytes (Alvarez et al. 2005).
Field water chemistry and fathead minnow bioassays: Basic water chemistry parameters including specific conductance, pH, temperature and dissolved oxygen were measured at cave and surface-water sites when samplers were deployed and collected using a YSI XL-600 multimeter. These same parameters and alkalinity and hardness were determined for each of the water samples collected for the fathead minnow bioassays. Water for these bioassays was collected in acid-washed, 3-liter plastic containers at each cave and surface water site and placed on ice as soon as possible for transport back to the University. Fathead minnow bioassays were conducted at the Ecotoxicology and Water Quality Research Laboratory, Oklahoma State University. General test protocols (test chamber size, loading rate, water renewal, feeding, etc.) followed methods described in US EPA (2002). Briefly, larval fish (<24 h) were exposed to sample water for 7 days and their survival and growth (as dry weight) were compared to that of fish maintained in laboratory water formulated to have similar hardness as the site water (US EPA 2002). Statistical analyses of growth and survival data were conducted using the Comprehensive Environmental Toxicity Information System (CETIS ver 1.1.1, Tidepool Scientific Software, McKinleyville, CA) and followed the standard US EPA decision tree for chronic toxicity data (US EPA 2002). In cases where a significant difference in growth and/or survival was observed between laboratory reference and field samples, the site was re-sampled and a dilution series of the site water was prepared (using laboratory water as the diluent) to determine if a dose response could be generated.
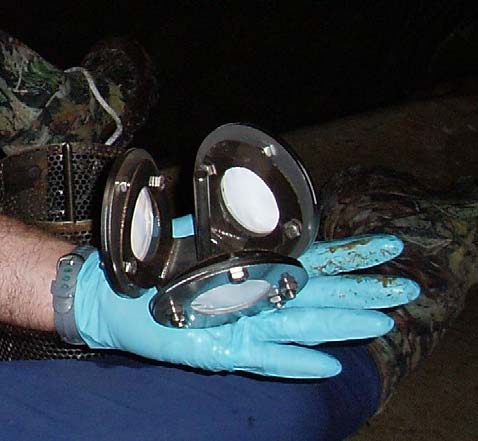
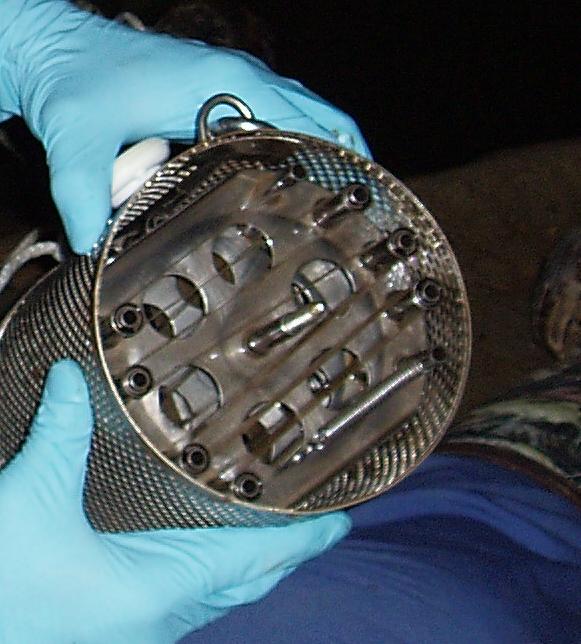
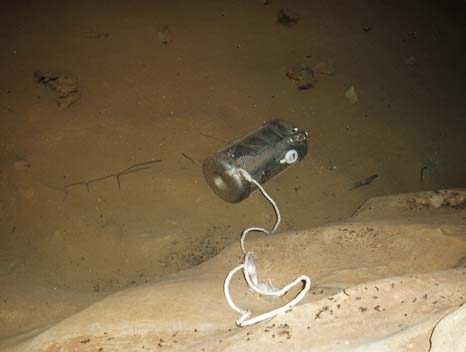
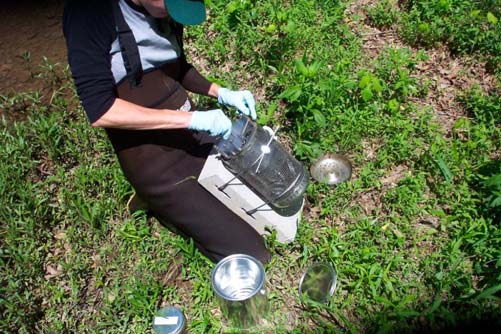
Figure 1. POCIS (left) and SPMD (right) sampling membranes deployed at the
cave and surface water sites.
Figure 2. Deployment of a stainless steel canister in a cave (left) and at one of the
surface sites (right).
Table 1. Organic wastewater compounds that were targeted in analyses of
the extracts from both the POCIS and SPMD passive sampling devices.
Descriptions of chemicals and associated laboratory reporting levels were
taken from Galloway et al. (2004) and Alvarez et al. (2005). *-Compound
analyzed in extracts from SPMD only
Laboratory
Reporting Level
Compound
Description
1,4-Dichlorobenzene
Moth repellant, fumigant,
deodorant Component of
1-Methylnapthalene
gasoline/diesel/crude oil
2,6-Dimethylnapthalene
Component of diesel and
2-Methylnapthalene
gasoline/diesel/crude oil
Herbicide intermediate
3-beta-Coprostanol
Carnivore fecal indicator
Nonionic detergent
Estrogen replacement
therapy and metabolite
Nonionic detergent
4-tert-Octylphenol
Nonionic detergent
Antifreeze component,
Fragrance in soap,
detergent, tobacco; flavor in
Wood preservative,
tar/diesel/crude
Used in Manufacture of
Polyaromatic hydrocarbon,
by-product of combustion
Fixative for perfumes and
Generally a plant sterol
Manufacture of resins;
Herbicide- non-crop
grass/brush control
By-product of wastewater
Stimulant 0.5 Flavor, odorant, in
Table 1. Continued
Laboratory
Reporting Level
Compound
Description
Manufacture of dyes,
explosives, and lubricants
Organophosphorous
Fecal indicator, also a plant
Primary nicotine metabolite
Manuf phenol/acetone;
component of fuels/paint
thinner Organophosphorous
Organophosphorous
Diethylhexylphthalate
Diethylphthalate
Antimicrobial antiviral;
fragrance in aerosols
Estrone*
Cosmetic component
Component of coal
Galaxolide (HHCB)*
Solvent for lacquers,
plastics, oils, silicon, resins
Manuf phenol/acetone;
component of fuels/paint
thinner Cigarettes, cough drops,
liniment, mouthwash
Fungicide 0.5 Liniment, food, beverage,
Methylsalicylate
UV-absorbing lotions
N,N-diethyltoluamide (DEET)
Insect repellant
Nonylphenol di-ethoxylates (total)
Nonionic detergent
metabolite Nonionic detergent
Octylphenol di-ethoxylates (total)
Octylphenol monoethoxylates
Nonionic detergent
metabolite termite control
Wood preservative
Table 1. Continued
Laboratory
Reporting Level
Compound
Description
Para-nonylphenol (total)
Nonionic detergent
Pentachlorophenol
tar/diesel/crude
Disinfectant 0.5
common in coal tar/asphalt
s3-Methyl-1(H)-indole (skatol) Odor in feces and coal tar
Generally a plant sterol
Solvent, degreaser;
Tetrachloroethylene
Veterinary: anthelminic
Tonalide (AHTN)*
Antimicrobial in soaps
Plasticizer and flame
Tributylphosphate
Antifoaming agent and
Triphenylphosphate
Plasticizer, resins, waxes,
finishes, roofing paper,
Table 2. Antibiotics and other pharmaceutical compounds that
were additionally targeted in analyses of the extracts from the
POCIS samplers.
Laboratory
Reporting Level
Compound
Description
Azithromycin Antibiotic
Carbamazapine Anticonvulsant
Chloramphenicol Antibiotic
Chlorotetracycline Antibiotic
Ciproflaxacin Antibiotic
Doxycycline Antibiotic
Enrofloxacin Antibiotic
Epi-chlorotetracycline Antibiotic 0.01 Epi-iso-chlorotetracycline Antibiotic
Epi-oxytetracycline Antibiotic
Epi-tetracycline Antibiotic
Erythromycin Antibiotic
Erythromycin-H2O Antibiotic
Ibuprofen Analgesic
Table 2. Continued
Laboratory
Reporting Level
Compound
Description
Iso-chlorotetracycline Antibiotic 0.010 Lincomycin Antibiotic
Lomefloxacin Antibiotic
Norfloxacin Antibiotic
Ofloxacin Antibiotic
Ormetoprim Antibiotic
Oxytetracycline Antibiotic
Roxithromycin Antibiotic
Sarafloxacin Antibiotic
Sulfachloropyridazine Antibiotic 0.005 Sulfadiazine Antibiotic
Sulfadimethoxine Antibiotic
Sulfamethazine Antibiotic
Sulfamethoxazole Antibiotic
Sulfathiazole Antibiotic
Tetracycline Antibiotic
Trimethoprim Antibiotic
Tylosin Antibiotic
Virginiamycin Antibiotic
Table 3. Additional organic compounds that were targeted in analyses of
the extracts from the SPMD samplers only. Analyses were conducted
with a method that allowed lower detection limits.
Laboratory
Reporting Level
Compound
Description
BDE100 (Brominated di-phenyl ether)
Organophosphorous
Table 3. Continued
Laboratory
Reporting Level
Compound
Description
Insecticide 0.2 Organochlorine,
Cyfluthrin Pyrethroid
Cyhalothrin Pyrethroid
DCPA Phthalate/herbicide
desulfnylFipronil
Insecticide 0.2 Organochlorine,
Insecticide 0.2 Organochlorine,
Insecticide 0.2 Organochlorine,
Firemaster Flame
Octachlorostyrene Organochlorine
breakdown product
Oxyfluorfen Herbicide 10
Dye intermediates, agricultural chemicals
PCA (p-chloroaniline)
and pharmaceuticals
Organochlorine, formerly used in hydraulic oils and some other industrial
PCB101 (Polychlorinated biphenyl) applications 2 PCB110
Table 3. Continued
Laboratory
Reporting Level
Compound
Description
Pentabromotoluene Flame
Pentachloronitrobenzene
treatment/fungicide
Tefluthrin Pyrethroid
Tetradifon Acaricide 0.5
Antimicrobial in soaps
Methoxytriclosan
Antiseptic, metabolite of
Trifluralin Herbicide
Principal Findings and Significance: The deployment time for the samplers ranged
from 28 to 35 days (Table 4). The first deployment at Star Cave in May – June 2006
failed because the cave stream went dry before the end of the exposure time. A second
successful deployment was conducted in June –August 2006 in which the sampler was
placed in more permanent water farther into the cave. Due to the possible connection
between the OK surface water site and this cave, a second sampler was deployed at
the OK surface site in conjunction with the second deployment at Star Cave.
Table 4. Deployment and retrieval dates and durations of exposure for the
POCIS and SPMD samplers at each cave and surface water site.
Exposure time
Deploy date
Retrieve date
OK-Surface-1st Deployment*
OK-Surface-2nd Deployment*
Cave Springs (AK)
January-Stansbury (OK)
Mgee and Long (OK)
Star (OK)- 1st Deployment*
Star – 2nd Deployment*
*= The stream in Star Cave went dry during the first deployment and a new sampler had to be redeployed. A second sampler was also placed at the OK-Surface because of the potential link between it and Star Cave.
On-site water chemistry: The water chemistry values measured at each of the sites are presented in Table 5. Due to instrument malfunctions, values were not available for all sites on all visits. The measured temperature at the cave sites ranged from 13-15 °C, pH was near neutral and dissolved oxygen was near saturation. Conductivity levels for the Oklahoma caves were higher than those for the Arkansas caves. The temperature values at the Oklahoma surface water site were approximately 10°C higher than that in the caves, and dissolved oxygen was below saturation.
Table 5. Water chemistry values at surface water and cave sites during deployment/retrieval of the samplers. Due to
instrument malfunctions, data for some of the sites were not available (NA).
OK-Surface
January-
Stansbury
Temperature (°C)
NA 21.61/23.52 14.9/NA 13.5/14.2 14.3/NA
Dissolved
NA 7.21/5.62 8.8/NA
Oxygen (mg/L)
NA 6.91/6.52 6.9/NA 7.0/7.0 7.1/NA
NA 4941/6022 269/NA 207/293 293/NA NA/258 4563/NA 3984
1-Value from 7 June 2006 retrieval of sampler
2-Value from 27 June 2006 deployment of sampler
3-Value from 27 June 2006 deployment of sampler
4-Value from 3 December 2005 reconnaissance of site
Detection of target compounds in passive sampler extracts: Lists of the compounds detected in the extracts from the POCIS and SPMD samplers are presented in Tables 6-9. These tables are differentiated based on analytical technique used and compounds analyzed. The data presented in Tables 6 & 7 were derived from the analyses for standard wastewater compounds (e.g. Zaugg et al. 2001), the data in Table 8 summarizes the antibiotic residues detected, while that in Table 9 summarizes the results of analyses for chlorinated and other organics using the experimental, unpublished analytical technique which provides lower detection limits. An additional summary of all detections is presented in Figure 3. Regardless of the analytical technique used, more compounds were detected in the surface water sites than in caves and more were detected in the OK-Surface site than in the AK-Surface site. This is not a surprising result given that the OK samplers were placed directly downstream from the outfall of a municipal wastewater treatment plant. A total of 27 different organic wastewater compounds were detected in the POCIS and SPMD extracts from the surface water and cave sites, with the majority of these found in the extracts from the POCIS samplers (Tables 6 & 7). Of these 27 compounds, 11 OWCs were detected in the caves, and Star Cave had the greatest number of detects, followed by Cave Springs Cave. Cholesterol and diethylexylphthalate were the most commonly detected compounds in the POCIS extracts, while no consistent trend in compound detection was apparent for the SPMD extracts. Measurable levels of antibiotics/pharmaceuticals were only found in the extracts from samplers at the OK surface water site and in Star Cave, with 8 compounds detected in the surface water and 2, carbamazapine and sulfamethoxazole, detected in the cave (Table 8). In most cases, the level of antibiotic measured in these extracts was 5 times the detection limit or higher. As would be expected, the majority of compound detections were observed in the SPMD extracts that were analyzed with the experimental method allowing for lower detection limits (Table 9). A total of 44 compounds were measured using this method, with this number including those with estimated levels below the laboratory reporting limit (LRL). Since this analytical technique is still being developed, a more conservative approach was taken when interpreting the data. Specifically, a "detect" was considered to have occurred only if the level of compound in the extract was at least 2X the LRL or level measured in the blanks. With this approach, 32 detections were observed, with 23 of these occurring in extracts from the cave samplers. In the OK surface site, the most commonly encountered residues were selected BDE and PCB congeners, organochlorine pesticides, and the common wastewater contaminants triclosan and methoxytriclosan. For the caves, the most common residues were BDEs and other selected flame retardants, organochlorine pesticides and triclosan and methoxytriclosan. Most of these residues were observed in the samples from January-Stansbury and Logan caves, although Star cave also had a number of detects at lower (<2X LRL) levels. The compounds triclosan and chlorpyrifos were also targeted as part of the OWC analyses (Table 1), although chlorpyrifos was only measured using the method with nanogram detection and triclosan was detected at more sites using this more sensitive method (Table 9).
Table 6. Compounds detected in extracts from the POCIS samplers. (D)=Detection at less than the laboratory reporting limit
(LRL); D=Detection above, but less than 2X, LRL or average blank concentration; D-2X=Detection at or above 2X, but less
than 5X, LRL or average blank concentration, D-5X=Detection at or above 5X LRL or average blank concentration.
Stansbury
Long Cave
4-tert-octylphenol ND
(D) (D) ND ND ND ND
(D)
(D)
(D)
ND ND ND ND D
(D)
(D)
D-2X D-2X D D-2X D
Diethylhexylphthalate
D D-5X (D)
(D) (D) D-5X D-2X
Diethylphthalate ND
ND ND ND ND D-5X
Ethanol,2-butoxy-,phosphate ND
Methylsalicylate ND
(D)
(D)
(D)
ND ND ND D
(D)
Octylphenol monoethoxylates (total) ND
(D)
ND ND ND ND D
(D)
ND ND ND ND ND D
(D)
tri(2-Chloroethyl)phosphate ND
tri(Dichlorisopropyl)phosphate ND
(D)
Detections
Table 7. Compounds detected in extracts from the SPMD samplers. (D)=Detection at less than the laboratory reporting limit
(LRL); D=Detection above, but less than 2X, LRL or average blank concentration; D-2X=Detection at or above 2X, but less
than 5X, LRL or average blank concentration, D-5X=Detection at or above 5X LRL or average blank concentration.
Stansbury
Compound
Long Cave
2,2',4,4'-Tetrabromodiphenylether ND D ND ND ND ND ND
Diethylhexylphthalate ND
Diethylphthalate
Tetrachloroethylene ND
(D)
Number of Detections
Table 8. Antibiotic and other pharmaceutical compounds detected in extracts from the POCIS samplers. (D)=Detection at less
than the laboratory reporting limit (LRL); D=Detection above, but less than 2X, LRL or average blank concentration; D-
2X=Detection at or above 2X, but less than 5X, LRL or average blank concentration, D-5X=Detection at or above 5X LRL or
average blank concentration.
Stansbury
Compound
Carbamazapine ND
Erythromycin-H2O ND
Sulfamethoxazole ND
Detections 0 8 0 0 0 0 2 0
Table 9. Chlorinated and other compounds detected in extracts from the SPMD samplers using the experimental analytical
method with lower detection limits. (D)=Detection at less than the laboratory reporting limit (LRL); D=Detection above, but
less than 2X, LRL or average blank concentration; D-2X=Detection at or above 2X, but less than 5X, LRL or average blank
concentration, D-5X=Detection at or above 5X LRL or average blank concentration.
Stansbury
Compound
Long Cave
D-2X D-5X D-2X D-2X D-2X D
D-5X D-2X D-2X D-2X D-2X ND
D D-5X (D)
D-2X D-2X D-2X D
D-2X D-2X ND
ND ND D-2X
D D-5X D-2X D-2X D-2X D-2X D
D-2X D-2X D
D D-5X D D-2X
D-2X D-5X ND ND ND ND D
D-2X D-5X D-5X D-2X D-2X D
D-5X D-5X D-5X D-2X D-2X ND
desulfnylFipronil ND
(D)
D-2X D-5X D-2X ND ND ND D-2X
FipronilSulfide ND
D-2X D-2X ND ND ND
D-5X D-5X ND
D-2X D-2X ND
D D-5X D ND ND ND ND ND
D-2X D-5X D-2X ND
D-5X D-5X D-2X ND ND ND D
D-5X D D-2X ND ND D D
D D D D ND ND
Table 9. Continued
Stansbury
Compound
Long Cave
ND ND ND ND (D) ND ND ND
(D) ND ND ND
D-2X D D-2X D ND ND ND
ND ND ND (D)
(D) ND ND D
ND ND ND ND ND ND D
Pentachloronitrobenzene ND
D-2X D-2X D-2X
D-2X D-5X D-5X D-2X D-2X D-2X D-2X D-2X
D-5X D-5X D-5X D-2X D-5X D-2X D-2X D-2X
(D)
D-5X D-2X D D-2X D-2X
Methoxytriclosan
D-5X D-5X ND
D-5X D-5X D-5X D-5X D-5X
Number of Detections
1 1 1 0 3 0 0 1
4 4 5 6 2 5 12 8
7 6 7 18 13 7 6 7
6 23 4 2 3 1 1 1
OWCs (POCIS and SPMD)
Chlorinated and other organics (SPMD)
ns 24
tio 22
f D 16
er o 14
mb 12
Nu 108
Figure 3. Summary of compound detections in extracts from the POCIS and SPMD samplers. OWCs (including
antibiotics) were analyzed in the μg range while the chlorinated and other organics were analyzed in the ηg range
using an experimental method that is under development.
Fathead minnow bioassay results: The water chemistry results derived from the fathead minnow bioassays were consistent with the data that were available from the field sampling (Table 5 & Table 10). The hardness of the water from the sites ranged between 76-190 mg/L as CaCO3, and a moderately hard laboratory water (80 -100 mg/L as CaCO3, USEPA 2002) was used as the reference water for the bioassays. For the majority of tests conducted, there were no significant differences between survival and growth of fish in laboratory versus cave water (Table 11). In Test 1 (3 May 2006), survival of fish exposed to water from Cave Springs Cave was reduced, although the difference was not statistically significant from that in the laboratory reference water. A follow-up bioassay with diluted Cave Springs water was conducted (Test 4, 7 June), with no effects observed. Similarly in Test 5 (9 June), a significant reduction in survival of fish exposed to Logan Cave water was observed, but a follow-up bioassay (Test 6, 20 June) indicated no effects. These data may suggest the presence of transitory stressors in the cave water that may be associated with run off events (Cave Springs Cave water was turbid with high flow on the day the sample was collected for Test 1), but consistent chronic effects were not indicated by the limited number of bioassays that were conducted for this study.
Table 10. Water chemistry ranges for all fathead minnow bioassays conducted with the surface and cave water
samples.
January-
Stansbury
6.8-7.1 6.8-7.5 6.4-7.3 6.4-7.3 6.5-7.0 6.7-7.2 6.3-7.0 6.7-7.2
375-379 472-549 274-357 209-280 272-393 227-303 335-436 343-363
Dissolved
8.3-9.0 7.1-7.9 8.0-8.7 8.5-10.0 8.4-9.7 8.0-8.9 8.2-8.3 8.5-8.6
Oxygen (mg/L)
Alkalinity
110-124 64-92 110-140 72-120 114-140
102-118 74-106 78-96
(mg/L CaCO3)
Hardness
120-146 104-114 120-190 76-116 132-154 106-132 90-156 96-122
(mg/L CaCO3)
Table 11. Results from bioassays with fathead minnows (Pimephales promelas)
exposed to water from the caves and surface water sites evaluated in the study.
Test 1, Date: 3 May 2006-10 May 2006
% Survival
Av. Dry Biomass (mg)
Range (mg)
Laboratory water
Cave Springs Cave
January-Stansbury
Mgee and Long Cave
Test 2, Date: 9 May -16 May 2006.
Site
% Survival
Av. Dry Biomass (mg)
Range (mg)
Laboratory water
AK-Surface 98 0.436
OK-Surface 94 0.439
Test 3, Date: 2 June 2006-9 June 2006.
Site
% Survival
Av. Dry Biomass (mg)
Range (mg)
Laboratory water
January-Stansbury
100 0.328 0.306-0.351
Cave Mgee and Long Cave
Test 4, Date: 7 June 2006-14 June 2006. Results of bioassays with fathead
minnows exposed to water from Cave Spring Cave.
Site
% Survival
Av. Dry Biomass (mg)
Range (mg)
Laboratory water
Test 5, Date: 9 June 2006-16 June 2006. *- Survival significantly different from that
in laboratory water at α=0.05.
Site
% Survival
Av. Dry Biomass (mg)
Range (mg)
Laboratory water
Table 11. Continued.
Test 6, Date: 20 June 2006-27 June 2006. Results of bioassays with fathead
minnows exposed to water from Logan Cave.
Site
% Survival
Av. Dry Biomass (mg)
Range (mg)
Laboratory water
12.5% Cave water
Test 7, Date: 29 June 2006-6 July 2006.
Site
% Survival
Av. Dry Biomass (mg)
Range (mg)
Laboratory water
Test 8, Date: 2 August 2006-9 August 2006.
Site
% Survival
Av. Dry Biomass (mg)
Range (mg)
Laboratory water
Test 9, Date: 27 October 2006-3 November 2006.
Site
% Survival
Av. Dry Biomass (mg)
Range (mg)
Laboratory water
January-Stansbury
Mgee and Long Cave
Signiicance of Results: Links between land use activities and residues in surface water and cave sites: A key objective in the management of both surface and cave water habitats is to understand the linkage between land use activities and water quality. In this study, a greater number of analytes were measured in the surface waters than in caves which is expected given the potential for aerial deposition and the larger drainage area available to influence surface water quality. As previously mentioned, the higher number of detections at the Oklahoma surface water site was expected given the proximity to a wastewater treatment outfall, and the number of detections is consistent with previous studies that analyzed either water samples or used passive sampling devices to evaluate the presence of OWCs downstream from WWTP outfalls (Galloway et al. 2004; Alvarez et al. 2005). Given the preliminary nature of this study, it is not possible to make any definitive conclusions regarding land use activities and the compounds that were detected in the caves, although some of the results are compelling. For example, antibiotic residues were only found at the OK surface site and Star Cave, the two sites known to have a hydrologic link (Aley 2005). Star Cave also
ranked higher than others in the number of organic wastewater contaminants that were
detected in the sampler extracts from that site. The presence of the antibacterial
compounds triclosan and methoxytriclosan at most of the cave sites may suggest a
wastewater influence at these sites as well.
The higher number of chlorinated organic residues that were detected at January-
Stansbury, Logan, and to some extent Cave Springs caves is also intriguing. Both
Logan and Cave Springs caves occur in drainage areas where there is increasing urban
development, but January-Stansbury Cave is in a relatively undeveloped area. One
common attribute of these sites is that the sampling canisters were placed relatively
close to the cave entrance (versus Star and Twin caves in which a significant
penetration of the system was required to reach permanent water). The water at these
sites may therefore be more influenced by aerial deposition of dusts that contain these
persistent organochlorine residues.
The significance of this study is that it did indicate the presence of a range of organic
wastewater contaminants and other organic compounds in water of the caves
examined. While the levels of these compounds are quite low (mostly in the 1 x 10-9g
range), their presence in these systems is a concern since so little is known about how
contaminants may influence cave stream fauna. Additional studies that further quantify
OWC levels in these habitats and investigate links between land use activities and cave
water quality are critical to understand the risk these chemicals pose to cave habitats.
Acknowledgement
This study would not have been possible if not for the efforts of a number of individuals
who gave their time and input toward project design and deploying the sampling
devices. Carol Becker of the USGS Oklahoma Water Science Center was instrumental
in the inception of the project and assisting with sampling. Steve Hensley and Richard
Stark of the US Fish and Wildlife Service, Tulsa Field Office, arranged access to the
caves and also helped with sample collection. Dr. Steven Zaugg of the USGS National
Water Quality Laboratory, Denver, Colorado, and Dr. Michael Meyer of the Organic
Geochemistry Laboratory, USGS Kansas Water Science Center analyzed the extracts
from the passive samplers for the target compounds.
References Cited
Aley, T.J. 1976. Hydrology and surface management. In: The National Cave
Management Symposium Proceedings. Speleo-books, Albuquerque, NM, pp. 44-45.
Aley, T.J. 2005. Groundwater recharge area delineation and vulnerability mapping of
Star cave and nearby hydrologically associated springs, Delaware County, Oklahoma: Report submitted to the U.S. Fish and Wildlife Service, October, 2005.
Alvarez, D.A., P.E. Stackelberg, J.D. Petty, J.N. Huckins, E.T. Furlong, S.D. Zaugg and
M.T. Meyer. 2005. Comparison of a novel passive sampler to standard water-
column sampling for organic contaminants associated with wastewater effluents enetering a New Jersey stream. Chemsophere, 61:610-622.
Crunkilton, R. 1984. Subterranean contamination of Meramec Spring by ammonium
nitrate and urea fertilizer and its implication on rare cave biota. Proceedings of the 1984 National Cave Management Symposium. Journal of the Missouri Speological Society, 25:151-158.
Galloway, J.M., B.E. Haggard, M.T. Meyers and W.R. Green. 2004. Occurrence of
pharmaceuticals and other organic wastewater constituents in selected streams in Northern Arkansas. U.S. Geological Survey, Scientific Investigations Report 2005-5140, 25p. Online at http://pubs.usgs.gov/sir/2005/5140/SIR2005-5140.pdf, accessed October 15, 2005.
Graening, G. and A. Brown. 2003. Ecosystem dynamics of an Ozark cave stream.
Journal of the American Water Resources Association, 39:1497-1507.
Huff, T. 2005. Overview of semipermeable membrane devices. Online at
http://wwwaux.cerc.cr.usgs.gov/spmd/spmd_overview.htm, accessed 21 October 2005.
Jones-Lepp, T.L., D.A. Alvarez, J.D. Petty and J.N. Huckins. 2004. Ploar organic
chemical integrative sampling and liquid chromatography-electroscopy/ion-trap mass spectroscopy for assessing selected prescription and illicit drugs in treated sewage effluents. Archive of Environmental Contamination and Toxicology, 47:427-439.
Kolpin, D.W., E.T. Furlong, M.T. Meyer, E.M. Thurman, S.D. Zaugg, L.B. Barber and
H.T. Buxton. 2002. Pharmaceuticals, hormones, and other organic wastewater contaminants in U.S. streams, 1999-2000: A national reconnaissance. Environmental Science and Technology, 36:202-1211.
NatureServe. 2005. NatureServe Explorer: An online encyclopedia of life [web
application]. Version 4.5. NatureServe, Arlington, Virginia. Online at http://www.natureserve.org/explorer, accessed 28 September 2005.
Proudlove, G. S. 2001. The conservation status of hypogean fishes. Environmental
Biology of Fishes, 62:201–213.
Simon , K. S. and A. L. Buikema, Jr. 1997. Organic Pollution of an Appalachian cave:
changes in macroinvertebtrates Populations and food. American Midland Naturalist, 138:387-401.
U.S. Environmental Protection Agency. 2002. Short-term Methods for Estimating the
Chronic Toxicity of Effluents and Receiving Waters to Freshwater Organisms, Fourth Edition, EPA-821-R-02-013
U.S. Geological Survey, 2004, Polar organic chemical integrative sampler (POCIS),
Online at http://www.cerc.usgs.gov/pubs/center/pdfDocs/POCIS.pdf, accessed 24 September 2005.
Vrana, B., H. Paschke, A. Paschke, P. Popp and G. Schuurmann. 2005. Performance of
semipermeable memberane devices for sampling of organic contaminants in groundwater. Journal of Environmental Monitoring, 7:500-508.
Zaugg, S.D., S.G. Smith, M.P. Schroeder, L.B. Barber, and M.R. Burkhardt,2001.
Methods of analysis by the U.S. Geological Survey National Water Quality Laboratory – Determination of wastewater compounds by polystyrene-divinylbenzene solid-phase extraction and capillary-column gas chromatography/mass spectrometry: U.S. Geological Survey Water Resources Investigations Report 01-4186.
Notable Achievements and Awards: The notable achievement is the detection of
these compounds in the cave water. This establishes the basis/need for additional study
of these cave systems.
Student Support: A summary of the number of students, their degree level and
discipline supported by the project in the following table:
Student Status
Disciplines
Source: http://water.okstate.edu/library/reports/project-reports/2006-projects/2006_bidwell_final.pdf
Published by Utah State University Extension and Utah Plant Pest Diagnostic Laboratory June 2008 Arthropod Diagnostician Extension Entomology Specialist What You Should Know • Very few insects are considered pests, and even fewer are actual y parasites of humans. • If you believe your body is infested with insects or other parasites, consult a physician immediately.
Psychotherapy Research 12(1) 1–21, 2002© 2002 Society for Psychotherapy Research HERMENEUTIC SINGLE-CASE EFFICACY DESIGN Robert ElliottUniversity of Toledo In this article, I outline hermeneutic single-case efficacy design (HSCED),an interpretive approach to evaluating treatment causality in single therapycases. This approach uses a mixture of quantitative and qualitative methodsto create a network of evidence that first identifies direct demonstrationsof causal links between therapy process and outcome and then evaluatesplausible nontherapy explanations for apparent change in therapy. I illus-trate the method with data from a depressed client who presented withunresolved loss and anger issues.