Doi:10.1016/j.jcis.2004.08.150
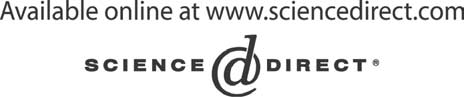
Journal of Colloid and Interface Science 283 (2005) 160–170
Sorption of the antibiotic ofloxacin to mesoporous and nonporous
alumina and silica
Keith W. Goyne Jon Chorover James D. Kubicki Andrew R. Zimmerman
Susan L. Brantley
a Department of Soil, Water and Environmental Science, University of Arizona, 429 Shantz Building, Tucson, AZ 85721-0038, USA
b Department of Geosciences, The Pennsylvania State University, University Park, PA 16802, USA
c Department of Geological Science, University of Florida, Gainesville, FL 32611, USA
Received 23 June 2004; accepted 17 August 2004
Available online 30 November 2004
Mesoporous and nonporous SiO2 and Al2O3 adsorbents were reacted with the fluoroquinolone carboxylic acid ofloxacin over a range
of pH values (2–10) and initial concentrations (0.03–8 mM) to investigate the effects of adsorbent type and intraparticle mesopores onadsorption/desorption. Maximum ofloxacin adsorption to SiO2 surfaces occurs slightly below the pKa2 (pH 8.28) of the antibiotic andsorption diminishes rapidly at pH > pKa2. For Al2O3, maximum sorption is observed at pH values slightly higher than the adsorbent's pointof zero net charge (p.z.n.c.) and less than midway between the pKa values of ofloxacin. The effects of pH on adsorption and ATR–FTIRspectra suggest that the zwitterionic compound adsorbs to SiO2 solids through the protonated N4 in the piperazinyl group and, possibly,a cation bridge; whereas the antibiotic sorbs to Al2O3 solids through the ketone and carboxylate functional groups via a ligand exchangemechanism. Sorption edge and isotherm experiments show that ofloxacin exhibits a higher affinity for mesoporous SiO2 and nonporousAl2O3, relative to their counterparts. It is hypothesized that decreased ofloxacin sorption to mesoporous Al2O3 occurs due to electrostaticrepulsion within pore confines. In contrast, it appears that the environment within SiO2 mesopores promotes sorption by inducing formationof ofloxacin–Ca complexes, thus increasing electrostatic attraction to SiO2 surfaces.
2004 Elsevier Inc. All rights reserved.
Keywords: Ofloxacin; Fluoroquinolone carboxylic acid; Mesoporosity; Sorption edge; Adsorption/desorption isotherms; ATR–FTIR spectroscopy;
Molecular modeling; Mineral–organic interactions
of FQCAs in wastewaters and streams with concentrationstypically reported in the range of ng L−1 to µg L−1
Fluoroquinolone carboxylic acids (FQCAs) are a class
Due to the land application or discharge of wastes to streams
of chemotherapeutic agents with antibacterial activity used
and our limited knowledge of the fate and interactions of
in human and veterinary medicines. Although absorptivity
FQCAs in aquatic and terrestrial environments, these com-
of orally administered FQCAs is high a portion of the
pounds are of significant environmental concern
dose passes through the body into human and animal ex-
Within the large class of FQCAs, ofloxacin is used to treat
crement. Thus, FQCAs have been detected in wastewaters
urinary and respiratory tract infections in humans and ani-
insufficiently treated by sewage treatment plants liq-
mals Although a significant number of studies have in-
uid animal manures and streams Recent studies in
vestigated aqueous ofloxacin–metal complexation reactions
the United States and Europe have documented the presence
much less work has been done on the sorption ofofloxacin to minerals and soil. Djurdjevic et al. de-
* Corresponding author. Fax: +1(520)-621-1647.
termined that sorption of ofloxacin to Al2O3 solids exhib-
E-mail address: (J. Chorover).
ited S-shaped isotherms when experiments were conducted
0021-9797/$ – see front matter 2004 Elsevier Inc. All rights reserved.
doi:10.1016/j.jcis.2004.08.150
K.W. Goyne et al. / Journal of Colloid and Interface Science 283 (2005) 160–170
from 19 to 140 µmol L−1 in neutral and very acidic (pH 1)
In this work, studies were conducted to investigate the
aqueous background solutions, and isotherms were L-shaped
sorption of the FQCA ofloxacin to nonporous and meso-
(Langmuir) in basic solutions (pH 11). The greatest extent
porous Al2O3 and SiO2 mineral sorbents. The objectives
of sorption occurred at neutral pH (0.7 mmol g−1) followed
of this study were (a) to investigate differences in ofloxacin
by sorption at pH 1 (0.5 mmol g−1) and sorption at pH 11
sorption to Al2O3 and SiO2 surfaces as a function of pH and
(0.38 mmol g−1) However, ofloxacin sorption onto
initial concentration, (b) to determine if mesoporosity influ-
Al(OH)3 gel exhibited a C-shaped (linear) isotherm and 21%
ences the amount of ofloxacin sorbed to Al2O3 and SiO2
of adsorbed ofloxacin was released from the mineral surface
solids, and (c) to determine the mechanism(s) through which
during desorption reactions Al dissolution and changes
ofloxacin binds to Al2O3 and SiO2 surfaces.
in solution pH as a function of ofloxacin adsorption werenot measured in either study. Thus, it is unclear to what ex-tent aqueous Al may compete with Al surfaces for ofloxacin
2. Materials and methods
complexation, and the multifunctionality of this compoundmay equate to several possible bonding mechanisms.
2.1. Properties of the adsorbate
Nowara et al. investigated the sorption of several
FQCAs, including levofloxacin (an active optical isomer of
ofloxacin), to soil, soil clay fractions, and soil minerals. This
study reported that adsorption of FQCAs to soil, soil clay
ne-6-carboxylic acid; 98% minimum purity) was purchased
fractions, and layer silicates is very high (95–99% removal
from Sigma–Aldrich Co. (St. Louis, MO) and used as re-
from initial aqueous concentrations ranging from 0.28 to
ceived. The compound was stored at 4 ◦C in the dark to min-
28 µmol L−1) and desorption in 0.01 M CaCl2 is very low
imize photolytically induced degradation Ofloxacin is
(<2.6% of adsorbed amount was released into solution). In-
a zwitterionic compound with acid dissociation constants of
frared spectra and microcalorimetry data were interpreted
6.08 (pKa1) and 8.25 (pKa2) As shown in
by Nowara et al. to suggest that FQCAs are bound to
, the antibiotic is primarily cationic below pKa1 (N4
clays via a cation bridge between charged basal surfaces and
in the piperazinyl group), anionic above pKa2 (3-carboxyl
the carboxylate functional group of FQCAs However,cation bridging is a relatively weak sorption mechanism thatis not associated typically with irreversible adsorption asobserved by Norwara et al. In addition, experimentalpH values were generally equal to or less than the pKa1of the FQCAs, thus cationic forms of the compounds mayhave been adsorbed to mineral surfaces. High Koc values(40,000–71,000) suggest that sorption was also influencedby the amount of organic carbon present in the soil andothers have reported sorption of FQCAs to dissolved humicacids
An additional factor that should be considered when
studying the fate of organic compounds in soils and sedi-ments is substrate surface morphology. Recent studies havedemonstrated that mineral mesoporosity (2–50 nm in porediameter), as occurs in naturally weathered geosorbentscan impact organic compound sorption. Zimmermanet al. observed that nitrogenous organic compoundssmaller than one-half the average mesopore diameter exhib-ited significantly greater surface area-normalized adsorptionto mesoporous alumina and silica, relative to nonporous ana-logues. Sorption of larger compounds was inhibited due tocompound exclusion from the internal mesopore surfaces.
Goyne et al. documented increased adsorption of thepesticide 2,4-D to alumina sorbents with increased meso-porosity. However, it should be noted that porosity, in andof itself, is not always the most important governing fac-
tor. For instance, 2,4-D did not adsorb to mesoporous or
Fig. 1. (a) Ionization of aqueous ofloxacin and (b) distribution of cationic
nonporous silica, presumably because of electrostatic repul-
(ofx+), zwitterionic (ofx0), and anionic (ofx−) ofloxacin in aqueous solu-
tion as a function of pH (pKa1 = 6.08 and pKa2 = 8.25)
K.W. Goyne et al. / Journal of Colloid and Interface Science 283 (2005) 160–170
group), and zwitterionic (net neutral) between pKa1 and
pH range from pH 2 to 10 for sorption edge experiments and
pKa2. Due to solubility issues (Csat =
pH 7.2 for isotherm experiments. Samples were spiked with
ofloxacin stock solutions were not prepared at concentra-
ofloxacin dissolved in 0.02 M CaCl2 to give initial ofloxacin
tions greater than 9 mM The dimensions of ofloxacin as
concentrations ranging from 0.03 to 8 mM for isotherm ex-
determined by measuring interatomic distances and account-
periments or 1 mM for sorption edge experiments. Although
ing for van der Waals radii of the atoms and constrained by
these concentrations are significantly greater than those de-
energy minimization using the COMPASS force field are
tected in natural waters our goal was to compare
1.2 × 0.95 × 0.6 nm.
ofloxacin sorption to mesoporous and nonporous silica andalumina, not to mimic solute concentrations found in im-
2.2. Adsorbent synthesis and characteristics
pacted waters. For the isotherm experiments, the initial pH ofthe ofloxacin stock solution and CaCl2 solution was adjusted
Four mineral adsorbents were used in the present work:
to 7.20. Samples were reacted on an end-over-end shaker
(1) mesoporous Al2O3 (Al-P242), (2) nonporous Al2O3(Al-
(7 rpm) in the dark at 298 K for 30 min. Adsorbent-free
NP37), (3) mesoporous SiO2 (Si-P700), and (4) nonporous
controls (no mineral) were reacted concurrently to measure
SiO2 (Si-NP8), where the subscripts refer to specific surface
compound loss resulting from sorption to centrifuge tube
area in m2 g−1. Al-NP37 and Si-NP8 were purchased from
walls or volatilization. Neither of these was found to be sig-
Alfa Aesar (Ward Hill, MA), Stock Nos. 40007 and 89709,
respectively. Al-NP37 was washed and dried as described in
After reaction, mineral suspensions were centrifuged at
Goyne et al. to remove an N-containing soluble con-
15,290g and 298 K for 45 min. An aliquot of supernatant
stituent associated with synthesis. Adsorbents Al-P242 and
solution was removed by pipet, stored in 4 ml amber vials,
Si-P700 were prepared using a neutral template route
and refrigerated for measurement of ofloxacin concentra-
The synthesis procedure and removal of the templates
tion. The remaining solution was aspirated, filtered through
from the fabricated adsorbents are detailed elsewhere
a 0.02-µm nominal pore size filter, acidified to pH < 2 with
All minerals, except Si-NP8, were ground gently prior
trace metal grade HCl, and refrigerated at 4 ◦C. Concentra-
to characterization and stored in polyethylene bottles prior
tions of Al and Si were determined using a Perkin–Elmer
to use. The physical and chemical characteristics were pub-
Elan DRC II inductively coupled plasma-mass spectrome-
lished previously and a summary is provided in
ter (ICP-MS). The pH of unfiltered and unacidified super-natant solution was measured using a calibrated Orion Ross
2.3. Batch sorption edge and isotherm experiments
semi-micro combination pH electrode attached to a Beck-man 390 pH meter.
Mineral adsorbents were suspended in a 0.02 M CaCl2
Ofloxacin concentrations in solution were determined by
(0.06 M ionic strength) background electrolyte solution to
measuring the concentration of nonpurgable organic carbon
give a sorbent surface area to solution ratio of 2.86 ×
(NPOC) and total nitrogen (TN) present in solutions acidi-
103 m2 L−1 in PTFE centrifuge tubes. Sorption experiments
fied to pH < 2 with trace metal grade HCl (Shimadzu Model
were conducted in the absence of pH buffers to prevent
TOC-VCSH, total organic carbon analyzer, equipped with a
competitive sorption between buffer constituents (e.g., phos-
TNM-1, total nitrogen measuring unit, and an ASI-V au-
phate) and ofloxacin for available sorption sites and to al-
tosampler). Standards were prepared by dissolving ofloxacin
low for measurement of pH shifts often indicative of lig-
in 0.02 M CaCl2. There were no significant differences
and exchange reactions All stock solutions or sam-
between ofloxacin concentrations calculated using NPOC
ples containing ofloxacin were wrapped in aluminum foil
or TN; thus all data shown are based on NPOC measure-
and/or stored in amber glassware to prevent or minimize
ments for simplicity. High-performance liquid chromatog-
raphy (HPLC) was not used, due to decreased column re-
Solutions of 0.06 M HCl or 0.02 M Ca(OH)2 were added
tention of ofloxacin when aluminum was present in solu-
to mineral suspensions (prior to reaction) to achieve a final
tion (i.e., peaks were broadened and decreased in height,
Table 1Physical characteristics and surface charge properties of the adsorbents
SBET (m2 g−1)
Dpore (nm)
3.4 ± 0.4
7.5 ± 0.1
8.2 ± 0.6
Note. See Goyne et al. for detailed methods and data analysis; SBET is the specific surface area ± std. dev. as measured by N2 BET; Dpore is the meanpore diameter ± std. dev. determined by the BJH method on the adsorption isotherm leg; Sip is the intraparticle surface area (within pores 2–20 nm in diameter)as percentage of total determined by BJH method; p.z.n.c. is the point of zero net charge ± 95% CI; values of p.z.n.c. not encountered in the pH range of theexperiment are expressed as the lowest pH values of the experiment; pKa1 and pKa2 are surface acidity intrinsic constants in accordance with the constantcapacitance model, using proton charging data from
K.W. Goyne et al. / Journal of Colloid and Interface Science 283 (2005) 160–170
evidently because of ofloxacin complexation with Al in
where the solver function of Microsoft Excel 2002
the aqueous phase). However, excellent agreement between
is used to vary iteratively the three fitting parameters Nt,
standards analyzed using HPLC and NPOC/TN was ob-
A, and β to maximize the coefficient of determination
served in the absence of aluminum. Mineral blanks (no
(R2 = 1).
ofloxacin) were reacted concurrently for correction.
Surface excess of ofloxacin was calculated as
2.4. Infrared spectroscopy
Γads = Cads,B − Cads,S ,
Attenuated total reflectance (ATR)–Fourier transform in-
frared (FTIR) spectroscopy was employed to document
where Γads is the surface excess of ofloxacin (µmol m−2),
changes in ofloxacin spectra as a function of pH and ionic
Cads,S and Cads,B are the equilibrium ofloxacin concentra-
composition and to investigate the mechanism of adsorp-
tions (µmol kg−1) in supernatant solutions of mineral sus-
tion. For aqueous phase spectra, stock solutions contain-
pensions (S) and for the corresponding blank (B) after the
ing 9.0 mM ofloxacin were prepared in 0.06 M NaCl and
reaction period, and SAS is the suspension concentration of
0.02 M CaCl2 background electrolyte solutions with pH val-
adsorbent (m2 kg−1).
ues ranging from pH 5 to 10. A 3-ml aliquot of solution was
Desorption reactions (isotherm experiments only) were
then transferred into an ATR cell equipped with a 45◦ ZnSe
initiated immediately after the adsorption step by adding a
flat plate crystal (ARK cell, Thermo Spectra-Tech, Inc.), and
mass of 0.02 M CaCl2 (pH 7.20) equivalent to that mass of
spectra were obtained by averaging 400 scans at 2 cm−1 res-
supernatant solution removed. Desorption reaction time was
olution on a Nicolet Magna 560 spectrometer.
equal to that for adsorption (30 min). After the desorption
Infrared spectra of adsorbed ofloxacin were obtained for
period, supernatant solutions were again removed by pipet
samples containing 11.80 g L−1 of mesoporous material re-
and analyzed. Adsorbate retained was calculated from
acted at pH 5.5 and 7.2 for 30 min with initial ofloxacin con-
centrations of 0 and 9.0 mM, as described previously. After
Γdes = Γads −
adsorption, samples were centrifuged and most of the super-natant solution was removed, except ca. 3.0 ml which was
where Γdes is the surface excess of ofloxacin (µmol m−2) af-
left in the suspension to create a slurry. ATR–FTIR slurry
ter the desorption step, Cdes,S is the ofloxacin concentration
samples were immediately transferred into the ATR cell for
in supernatant solution of mineral suspension (S) after the
data collection. Spectra of adsorbed ofloxacin were obtained
desorption reaction (µmol kg−1), Mtot,soln is the total mass
by subtracting those of the ofloxacin-free slurry.
of solution (kg) in the reaction vessel during desorption,Ment is the mass of entrained solution (kg) remaining in the
2.5. Molecular modeling of infrared frequencies
centrifuged adsorbent pellet after aspiration of adsorptionstep supernatant, and SA is the total surface area of adsor-
Gas-phase, infrared frequencies of cationic, anionic,
bent (m2) in the reaction vessel.
zwitterionic ofloxacin, and an Al–ofloxacin complex were
Sorption data were fit to the Langmuir–Freundlich equa-
calculated at the B3LYP/6-31G(d) level using the
tion which has been shown to successfully model a
Gaussian 98 program Frequency values were corrected
number of other organic compounds on heterogeneous sur-
by multiplying calculated values by 0.96 Model struc-
tures of the cationic, zwitterionic, and anionic species of
ofloxacin were modeled with and without explicit hydra-
tion of the polar functional groups. In addition, the species
(OH2)4Al–ofloxacin (in the zwitterionic state) was modeled.
The Al3+ was bonded in a bidentate fashion to one O atom
i is adsorbate surface excess (µmol m−2), Nt is the
total number of binding sites, A is a parameter related to
of the carboxylate group and to the O atom of the adjacent
the binding affinity (K
ketone group. The output files were then used to view an-
0; K0 = A1/β ), ci is the equilibrium
aqueous concentration of ofloxacin (µmol L−1), and β is a
imated vibrational motions in Molden Version 3.9 for
fitting parameter When β = 1, the Langmuir–
band assignment.
Freundlich equation reduces to the Langmuir equation
qi = NtAci
(β = 1),
3. Results and discussion
whereas, if ci or A approach zero, it equation reduces to the
3.1. Ofloxacin adsorption as a function of pH
Freundlich equation
The effects of pH on ofloxacin adsorption to SiO2 sur-
qi = Ac
(ci or A → 0).
faces are shown in Above pH 5.0, Si-P700 adsorbs
The Langmuir–Freundlich equation was fit to the experi-
significantly more ofloxacin than does Si-NP8. Maximum
mental data using the method outlined in Umpleby et al.
ofloxacin sorption to these minerals (80.3 and 67.2% for
K.W. Goyne et al. / Journal of Colloid and Interface Science 283 (2005) 160–170
Fig. 3. Ofloxacin sorbed on (a) Si-NP
Fig. 2. Ofloxacin adsorbed (Γ
8 and Si-P700 or (b) Al-NP37 and
ads) on (a) Si-NP8 and Si-P700 or (b) Al-NP37
242 after 30 min of adsorption (Γads ) or desorption (Γdes) reaction
242 as a function of pH after 30 min of reaction time (duplicate
time (duplicate means are shown and error bars, where visible, represent
means are shown and error bars, where visible, represent 95% CI). Sur-
95% CI). Surface excess is expressed as micromoles per square meter and
face excess is expressed as micromoles per square meter and molecules per
molecules per square nanometer.
square nanometer.
In contrast, indicates that ofloxacin sorption to
Si-P700 and Si-NP8, respectively) occurs slightly below the
mesoporous Al2O3 (Al-P242) was consistently lower than
pKa2 (pH 8.28) of the antibiotic and diminishes rapidly at
sorption to nonporous Al2O3 (Al-NP37) over the full pH
pH > pKa2. Thus, we presume that cationic and zwitterionic
range investigated. Sorption of the antibiotic to Al2O3 solids
ofloxacin are adsorbed to the negatively charged silica sur-
increases significantly above pH 5.0, concurrent with in-
faces (i.e., ≡SiO− functional groups) via the protonated N4
creased aqueous concentrations of zwitterionic ofloxacin. In
in the piperazinyl group. At the pH of maximum measured
this case, Γ max (88.2 and 73.3% for Al-NP37 and Al-P242,
sorption, only 45 and 9% of the dissociated silanol groups
respectively) occurs at pH slightly less than midway be-
on the surface of Si-P700 and Si-NP8 respectively, are
tween the pKa values of ofloxacin (pH 7.16) and slightly
occupied by the compound, suggesting that sorption was not
higher than the adsorbent point of zero net charge (p.z.n.c.;
limited by the availability of ≡SiO− sites.
At pH greater than that of Γmax, adsorption dimin-
The fact that Si-NP8 adsorbs more ofloxacin below pH 5
ishes as alumina surfaces become increasingly negatively
than does Si-P700 can be attributed to 50% higher density
charged, thus repelling zwitterionic and anionic ofloxacin
of dissociated surface silanol groups on Si-NP8 at pH 3–5
from the surface. These data lead us to hypothesize that
according to surface charge data reported previously
ofloxacin sorption to ≡AlOH+ surface sites via the dissoci-
However, at this pH, Si-P700 has a greater fraction of disso-
ated 3-carboxyl group (COO−) of the zwitterion is initiated
ciated silanol groups occupied by ofloxacin. For instance,
between pH 4.5 and 5.5. Initiation likely occurs closer to pH
ofloxacin sorbed onto Si-P700 at pH 3.32 occupies 62%
5.5 based on . However, the same mechanism of sorp-
of the ≡SiO− groups, whereas at pH 3.40 ofloxacin occu-
tion may not be applicable below pH 5.0.
pies only 34% of the ≡SiO− groups on Si-NP8. Overall, it
Ofloxacin adsorption to Al2O3 decreases from pH 2 to
appears that the presence of intraparticle mesoporosity in-
4.5, and the same reproducible trend appears in the data
creases ofloxacin sorption to SiO2 surfaces.
sets for both alumina solids We are unable to ex-
K.W. Goyne et al. / Journal of Colloid and Interface Science 283 (2005) 160–170
Table 2Langmuir–Freundlich parameters for SiO2 and Al2O3 isotherms
Nt (µmol m−2)
A (µM−1)
K0 (µM−1)
R2 (n)
4.6 × 10−5
1.3 × 10−3
5.7 × 10−5
1.8 × 10−3
1.2 × 10−3
8.2 × 10−4
4.8 × 10−4
1.1 × 10−3
1.3 × 10−3
2.3 × 10−3
7.0 × 10−3
4.5 × 10−4
9.5 × 10−4
1.1 × 10−3
5.6 × 10−4
4.1 × 10−3
Note. Nt is adsorption capacity; A is a parameter related to mean binding affinity (K0); β is an exponent related to the heterogeneity of binding site energydistribution; K0 is the mean binding affinity; R2 is the coefficient of determination, and n is the number of sample points utilized to calculate the parameters.
plain the cause of this occurrence, other than to suggest that
measurement of proton or hydroxide production, but varied
the mechanism of ofloxacin adsorption is likely different
among the four adsorbents.
than above pH 5.0. Below pH 5, the fraction of zwitterionic
Equilibrium pH values for SiO2 samples were relatively
ofloxacin is very low and surface complexation via
constant (pH 7.20–7.40) except for those reacted at the high-
COO− is likely insignificant. It is possible that dissolution
est initial concentration of ofloxacin (pH 6.77 and 6.65 for
of Al at acidic pH promotes the formation of Al-bridged
Si-NP8 and Si-P700, respectively). This proton production
dimers whose adsorption could be enhanced relative to
likely decreased the quantity of ofloxacin sorbed to these
monomeric ofloxacin. Irrespective of the mechanism(s) of
samples, relative to that adsorbed at pH 7.20 (see
adsorption, it is clear that intraparticle porosity does not in-
and may slightly skew the isotherm shapes shown in
crease sorption of ofloxacin to alumina surfaces. This is de-
However, this shift to more acidic pH is indicative of proton
spite the fact that ofloxacin is smaller (1.2 × 0.95 × 0.6 nm)
displacement from ≡SiOH surface functional groups. This
than the nominal pore size of Al-P242 (8.2 nm) and that these
is verified by comparing the moles of ofloxacin adsorbed
alumina minerals have nearly identical surface charge prop-
to the predicted density of ≡SiO− present at equilibrium
pH for samples with the highest initial ofloxacin concentra-tion. At pH 6.78, 0.79 µmol m−2 of ofloxacin is adsorbed
3.2. Ofloxacin adsorption/desorption isotherms
to Si-NP8 and the predicted density of ≡SiO− at this pHis 0.74 µmol m−2. However, 1.15 µmol m−2 of ofloxacin
Adsorption/desorption experiments were conducted to in-
is adsorbed to Si-P700 with a predicted dissociated silanol
vestigate ofloxacin sorption and retention as a function of
site density of 0.34 µmol m−2 at pH 6.65. Thus cationic
initial sorptive concentration at a target equilibrium pH 7.20
ofloxacin is displacing adsorbed protons upon adsorption:
The isotherm data agree with findings from sorp-
≡SiOH + ofx+ ≡SiO–ofx + H+.
tion edge experiments in that mesoporous SiO2 consis-tently sorbs more ofloxacin than nonporous SiO
Calculations show that for Si-NP
8 the stoichiometry of this
whereas, the opposite is true for Al
cation exchange reaction is 1:1 as shown in Eq. For Si-
The Langmuir–Freundlich isotherm results in in-
P700, the measured release of H+ is somewhat lower than the
dicate that N
moles of ofloxacin adsorbed in excess of negatively charged
t (sorption maximum) and A (measure of bind-
ing affinity) for the two minerals adsorbing greater amounts
sites, suggesting the possibility of additional sorption mech-
of ofloxacin are very similar and higher than the lower affin-
anisms (e.g., cation bridging).
ity sorbents. In addition, values of N
In contrast, pH values for Al
t indicate that whereas
2O3 reacted samples show
the sorption maximum was reached by Si-NP
an increase in pH with increased ofloxacin adsorption, re-
to the other minerals is below the predicted maximum. No
gardless of whether acid or base was added to reach the
other discernible trends are apparent within the Langmuir–
target equilibrium pH of 7.20. The range of Al2O3 sam-
Freundlich isotherm parameters.
ple pH values (pH 6.45–7.58) are located very near maxi-
As mentioned under Section isotherm experiments
mum adsorption (see and the shape of the Al2O3
were conducted in the absence of pH buffers. Thus, ofloxacin
isotherms should be very similar to that of isotherms where
stock solution and 0.02 M CaCl
pH = 7.20 for all samples. These results are suggestive of
2 background electrolyte so-
lution were adjusted with base (0.02 M Ca(OH)
ligand exchange reactions between ≡AlOH+ and the COO−
7.20, and additional acid (0.06 M HCl) or base was added at
the beginning of each experiment to offset any pH changes
≡AlOH + ofx0 ≡Al–ofx+ + OH−.
resulting from buffering of the minerals themselves The amount of acid/base added to reaction vessels was
This could explain the slight hysteresis between the Al2O3
constant for the full isotherm of each adsorbent to permit
adsorption/desorption isotherms and adsorption
K.W. Goyne et al. / Journal of Colloid and Interface Science 283 (2005) 160–170
via the COO− functional group agrees with the sorp-
background electrolyte solutions at a particular pH from
tion mechanism inferred from sorption edge experiments
their counterparts containing dissolved ofloxacin.) Ofloxacin
). Dissolution data for Al adsorbents are very simi-
dissolved in the different background electrolyte solutions
lar and show elevated Al concentrations in solution (582 and
were compared because it is known that ofloxacin forms
593 µM for Al-P242 and Al-NP37, respectively) for samples
strong bonds with divalent cations possi-
reacted with the highest initial concentration of ofloxacin,
bly through interaction between the carboxylic and ketone
relative to mineral controls (<6.4 µM) and solubility data
published previously for these materials (<1.4 µM)
In we observe that the C=O stretch of COOH
This is also consistent with adsorption via a ligand exchange
(1710 cm−1) is lost as pH increases. Subse-
reaction, which would tend to promote Al dissolution
quently, intensity of the asymmetric (1585 cm−1) andsymmetric (1340 cm−1) stretch of COO− increases
3.3. Infrared spectra of dissolved ofloxacin and
with increasing pH. There is also an increase in the inten-
sity of the wavenumber that we assign as vibrations as-sociated with protonation of N4 in the piperazinyl group
Although several studies have used infrared spectroscopy
(1400 cm−1) Assignments of the remaining vibrations
to investigate the interaction of ofloxacin with polyva-
observed in are as follows: C=O stretch of ketone
lent cations these studies have not documented
group (1620 cm−1) C=C aromatic stretching
changes in aqueous phase spectra as a function of pH (i.e.,
(1530 cm−1) and C–O–C stretching of the ether group
functional group protonation) and cation composition (i.e.,
aqueous phase complexation). Thus, as noted by Macias
Molecular orbital models of ofloxacin calculations on
et al. assigning functional groups appropriately to vi-
the explicitly solvated models generally produce similar fre-
brations observed in powder IR spectra of ofloxacin–metal
quencies (±35 cm−1; for the main peaks of inter-
complexes can be challenging. In order to help interpret
est. The C=O stretch of the carboxylic acid for explicitly
ATR–FTIR spectra of ofloxacin adsorbed to the mineral sur-
solvated cationic ofloxacin is an exception because it is cal-
faces, we first collected ATR–FTIR difference spectra of
culated to occur at 80 cm−1 lower than the experimental
ofloxacin (9 mM) dissolved in 0.06 M NaCl and 0.02 M
observation. The main reason for this discrepancy is the ap-
CaCl2 from pH 5 to 10 as shown in (Dif-
proximate representation of the electron correlation in the
ference spectra were obtained by subtracting spectra of the
Fig. 4. Attenuated total reflectance–Fourier transform infrared (ATR–FTIR)
Fig. 5. Attenuated total reflectance–Fourier transform infrared (ATR–FTIR)
difference spectra of ofloxacin in 0.06 M NaCl from pH 5 to 10. Difference
difference spectra of ofloxacin in 0.02 M CaCl2 from pH 5 to 10. Differ-
spectra were obtained by subtracting spectrum of 0.06 M NaCl at a partic-
ence spectra were obtained by subtracting spectrum of 0.02 M CaCl2 at a
ular pH from spectrum of ofloxacin dissolved in 0.06 M NaCl at the same
particular pH from spectrum of ofloxacin dissolved in 0.02 M CaCl2 at the
K.W. Goyne et al. / Journal of Colloid and Interface Science 283 (2005) 160–170
Fig. 7. Attenuated total reflectance–Fourier transform infrared (ATR–FTIR)
Fig. 6. Attenuated total reflectance–Fourier transform infrared (ATR–FTIR)
difference spectra of (a) ofloxacin in 0.02 M CaCl2 at pH 7.2 and
difference spectra of (a) ofloxacin in 0.02 M CaCl2 at pH 5.5 and
ofloxacin-adsorbent slurry at pH 7.2 for (b) Al-P242 and (c) Si-P700. Dif-
ofloxacin-adsorbent slurry at pH 5.5 for (b) Al-P242 and (c) Si-P700. Dif-
ference spectra were obtained by subtracting spectrum of 0.02 M CaCl2
ference spectra were obtained by subtracting spectrum of 0.02 M CaCl2
from ofloxacin dissolved in 0.02 M CaCl2 (a) and by subtracting 0.02 M
from ofloxacin dissolved in 0.02 M CaCl2 (a) and by subtracting 0.02 M
CaCl2-adsorbent slurry from ofloxacin–0.02 M CaCl2-adsorbent slurry
CaCl2-adsorbent slurry from ofloxacin–0.02 M CaCl2-adsorbent slurry
molecule. Although the LYP gradient-corrected correlation
Difference spectra of ofloxacin and ofloxacin–mineral
functional is adequate for most of the bonds in the mole-
complexes formed at pH 5.5 and 7.2 are shown in
cule, C=O bonds depend more strongly on electron corre-
respectively. (Difference spectra were obtained by
lation so this calculated mode ends up with a larger
subtracting a spectrum of mineral suspended in 0.02 M
discrepancy with experiment. A more accurate method for
electron correlation could possibly decrease this error, but
2 at a specific pH from corresponding samples re-
acted with ofloxacin.) The spectra indicate that sorption of
methods such as (MP2, Møller Plesset second-order pertur-
ofloxacin occurs via similar mechanisms for a given min-
bation) require far more computational time and are not
eral type, irrespective of pH. For example, ofloxacin sorbed
practical for a model of this size. However, without explicitsolvation, COO− stretching was predicted to occur in the
to Al2O3 at pH 5.5 and 7.2 shows a dra-
1725–1710 cm−1 region, an error of 100 cm−1 or more. In
matic decrease in the intensity of the ketone (1620 cm−1)
addition, linear regression of calculated versus predominant
and asymmetric COO− (1590 cm−1) vibrations. However,
experimental frequencies (n 16) for the three explicitly
the same spectra show a large increase in the intensity of
solvated molecules yielded slopes of 1 (±0.03) and R2 val-
peaks at 1530 and 1275 cm−1.
ues of 0.99. Based on this good correlation, we conclude that
We attribute the change at 1530 cm−1 to a downward
the model calculations produce realistic vibrational frequen-
shift in frequency of the ketone and/or asymmetric COO−
cies for ofloxacin and can be used to help interpret spectra
stretch upon innersphere complexation with an Al center on
of unknown structures such as surface complexes.
the mineral surface. Molecular modeling of an ofloxacin–Al
Ofloxacin spectra collected in 0.02 M CaCl
complex, whereby Al is bound via bidentate complexation
show trends similar to those in as pH increases, but vi-
to the ketone and carboxylate functional groups
brations associated with the ketone and asymmetric stretch
indicates that the downward shift is attributable to
of COO− are more intense and broadened in the presence of
the ketone vibration (predicted at 1525 cm−1; ). Al-
Ca2+. We propose, as have others that this is indica-
though others have suggested that the ketone group vibration
tive of a weak Ca–ofloxacin complex that forms between the
may decrease in frequency upon strong complexation with
ketone and carboxylate functional groups. Apparently, simi-
a metal ion our experimental data and calculations
lar complexes are not formed in the presence of monovalent
demonstrate this occurrence, as do calculations performed
ions with a large hydrated radius (e.g., Na+;
by Sagdinc and Bayarı Perhaps more importantly, the
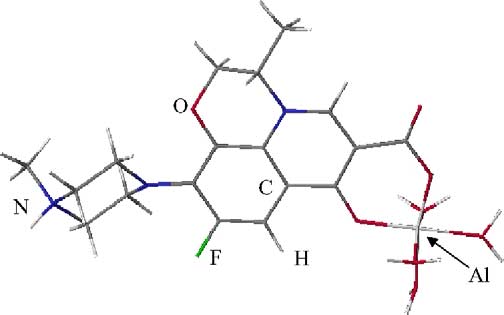
K.W. Goyne et al. / Journal of Colloid and Interface Science 283 (2005) 160–170
Table 3Selected experimental and calculated vibrational frequencies for ofloxacin
Experimental (cm−1) Calculated (cm−1)
C=O stretch of carboxylic acid 1705
C=O stretch of ketone group
COO− asymmetric stretching
COO− symmetric stretching
C–O–C stretching of ether group 1058
Fig. 8. Molecular structure of (OH2)4Al–ofloxacin (zwitterionic) complex.
C=O stretch of ketone group
This complex has the same basic orientation as the ofloxacin molecules
COO− asymmetric stretching
COO− symmetric stretching
C–O–C stretching of ether group 1054
ketone frequency (1620 cm−1). This suggests that ofloxacin
may be bonded to SiO2 surfaces via a cation bridge atpH 5.5, although the same occurrence is not observed at
C=O stretch of ketone group
COO− asymmetric stretching
pH 7.2 This supports our contention that ofloxacin
sorbs to SiO2 through the protonated piperazinyl group, via
COO− symmetric stretching
weaker electrostatic interaction, and through a cation bridge.
C–O–C stretching of ether group 1053
Note. Experimental assignments are reported for ofloxacin in 0.06 M NaCl;
3.4. Mechanisms for enhanced or reduced ofloxacin
N.C.V. is no calculated vibration. However, the calculated results where
sorption to mesoporous adsorbents
N.V.C. is reported are reasonable. This is due to the presence of COOH,only, in the modeled cationic molecule; whereas, the experimental data con-
Enhanced ofloxacin sorption to mesoporous SiO
tain both cationic (dominant) and zwitterionic (minor) species in solution.
pothesized to occur due to higher concentration of Ca2+([Ca2+]) within the confines of a pore. Our calculations
IR data strongly support our hypothesis that ofloxacin sorbs
indicate that electric double layer (EDL) thickness extend-
to Al2O3 surfaces via a ligand exchange process
ing from a planar solid–water interface in 0.02 M CaCl2 is
In contrast, IR spectra of ofloxacin sorbed to SiO2
1.2 nm Given that the mean pore diameter of Si-P700
are similar to those of dissolved ofloxacin,
is 3.4 nm, EDLs within pore confines would not quite over-
despite the fact that Si-P700 adsorbed 2–3 times more
lap near the center of this circular pore. However, the pres-
ofloxacin per unit mass than did Al-P242
ence of EDLs extending from pore walls into the pore cen-
However, the spectra of the ofloxacin–SiO2 complex col-
ter should increase [Ca2+] and decrease [Cl−] in the pore,
lected at pH 5.5 shows an increase and broadening of the
relative to concentrations of these ions near surfaces exter-
Table 4Correlation of calculated vibrations and assignments of an Al–ofloxacin complex to experimental vibrations
Calculated (cm−1)
Experimental (cm−1)
C=O stretch of carboxylate–Al complex
1593 (m), 1572 (m)
C=O stretch of ketone group
1488 (s), 1468 (m)
1415 (m), 1413 (s)
1378 (m), 1372 (m)
1325 (m), 1322 (m)
1106 (w), 1075 (m)
C–O–C stretch of ether group
Note. Vibration assignments are based on model results and experimental vibrations are reported for ofloxacin–Al-P242 slurry spectra collected at pH 7.2
K.W. Goyne et al. / Journal of Colloid and Interface Science 283 (2005) 160–170
nal to pores The higher [Ca2+] within pores would
sion from specific, but as yet undetermined, aluminol sites
promote ofloxacin–Ca2+ complexation via the carboxylate
within pore cavities. Overall, the data indicate that intraparti-
group and effectively increase the amount of pos-
cle mesopores can enhance ofloxacin sorption to adsorbent
itive charge on an ofloxacin ion. Therefore, a zwitterionic
minerals, but the degree of enhancement may be diminished
ofloxacin molecule could bind to the surface via the proto-
or even reversed by other mitigating factors (e.g., surface
nated N4 of the piperazinyl group and, at the same time, bind
charge properties).
via a cation bridge as others have observed This ap-pears to be a reasonable explanation for increased ofloxacinsorption to mesoporous Si-P700 and it agrees with our other
data that suggest ofloxacin binds to Si-P700 surfaces by morethan one mechanism.
The authors thank Mary Kay Amistadi for laboratory as-
It is unclear why adsorption to Al-NP
sistance, Sridhar Komarneni, Bharat Newalkar, and Stephen
37 exceeds that of
Stout for mineral synthesis and preparation, and Chad Trout
242. Ofloxacin sorption to Al-P242 is not reduced due
to exclusion of the antibiotic from the pores, differences in
for assistance with molecular modeling. Financial support
surface charge properties, or increased dissolution of Al-
was provided by the Penn State Biogeochemical Research
Initiative for Education (BRIE) sponsored by NSF (IGERT)
242 resulting in aqueous and solid phase Al competition for
ofloxacin. In addition, ATR–FTIR and molecular orbital cal-
Grant DGE-9972759 and by the Penn State Materials Re-
culations indicate that ofloxacin forms a strong innersphere
search Science and Engineering Center (MRSEC) spon-
complex with surface aluminum molecules. Thus, we con-
sored by NSF Grant DMR-0080019. Andrew Zimmerman
clude that the coexistence of positive- and negative-charged
acknowledges donors to the American Chemical Society
Petroleum Research Fund for partial support of this re-
2O3 may inhibit sorption of ofloxacin within
the pore confines, relative to external surfaces. If ofloxacin
search, and James Kubicki acknowledges the support of
sorbs to Al centers via the ketone and carboxylate groups,
Stony Brook-BNL collaboration to establish a Center for
then over a wide range of pH the protonated N
Environmental Molecular Sciences (CEMS). Computation
azinyl group will be positioned toward the pore center. This
was supported, in part, by the Materials Simulation Center,
may, depending on the local surface charge properties, re-
a Penn State MRSEC and MRI facility.
sult in an energy barrier that prohibits or hinders ofloxacinsorption to particular aluminol sites. In other words, repul-sive forces between the protonated N
4 of the piperanzinyl
groups and ≡AlOH+ surface functional groups may inhibit
[1] A.K. Sharma, R. Khosla, A.K. Keland, V.L. Mehta, Indian J. Pharm.
ofloxacin sorption within pore confines.
26 (1994) 249.
[2] E.M. Golet, A.C. Alder, W. Giger, Environ. Sci. Technol. 36 (2002)
[3] C.G. Daughton, T.A. Ternes, Environ. Health Perspect. 107 (1999)
[4] J. Tolls, Environ. Sci. Technol. 35 (2001) 3397.
Intraparticle mesoporosity in SiO2 solids was found to re-
[5] D.W. Kolpin, E.T. Furlong, M.T. Meyer, E.M. Thurman, S.D. Zaugg,
sult in increased uptake of ofloxacin when adsorption was
L.B. Barber, H.T. Buxton, Environ. Sci. Technol. 36 (2002) 1202.
normalized to sorbent surface area. Relative to the non-
[6] A. Hartmann, E.M. Golet, S. Gartiser, A.C. Alder, T. Koller, R.M.
porous solid, the presence of intraparticle porosity resulted
Widmer, Arch. Environ. Contam. Toxicol. 36 (1999) 115.
[7] E.R. Campagnolo, K.R. Johnson, A. Karpati, C.S. Rubin, D.W. Ko-
in a statistically significant sorption enhancement through-
plin, M.T. Meyer, J.E. Eseeban, R.W. Currier, K. Smith, K.M. Thu,
out the isotherms and over most of the sorption edge for the
M. McGeehin, Sci. Total Environ. 299 (2002) 89.
porous silica adsorbent (Si-P700). Observations of proton re-
[8] S.E. Jørgensen, B. Halling-Sørensen, Chemosphere 40 (2000) 691.
lease in association with ofloxacin sorption and sorption in
[9] P.T. Djurdjevic, M. Jelikic-Stankov, J. Pharm. Biomed. Anal. 19
excess of the surface site density of ≡SiO− groups (mea-
[10] M. Sakai, A. Hara, S. Anjo, M. Nakamura, J. Pharm. Biomed. Anal. 18
sured in absence of ofloxacin) indicate that the compound
(1999) 1057.
is capable of displacing protons complexed with silanol
[11] D.L. Ross, C.M. Riley, Int. J. Pharm. 93 (1993) 121.
groups as it sorbs to SiO2 surfaces via the protonated N4
[12] H.-R. Park, K.-Y. Chung, H.-C. Lee, J.-K. Lee, K.-M. Bark, Bull. Ko-
of the piperazinyl group. Conversely, ofloxacin adsorption
rean Chem. Soc. 21 (2000) 849.
[13] P.T. Djurdjevic, M. Jelikic-Stankov, I. Lazarevic, Bull. Chem. Soc.
2O3 was significantly higher than that to
Jpn. 74 (2001) 1261.
mesoporous Al2O3 in both sorption edge and isotherm ex-
[14] B. Macías, M.V. Villa, I. Rubio, A. Castiñeiras, J. Borrás, J. Inorg.
periments. The observed hydroxyl release concurrent with
Biochem. 84 (2001) 163.
ofloxacin adsorption and shifts in the frequency of ketone
[15] M. Tanaka, T. Kurata, C. Fujisawa, Y. Ohshima, H. Aoki, O. Okazaki,
and increased intensity of carboxylate stretching vibrations
H. Hakusui, Antimicrob. Agents Chemother. 37 (1993) 2173.
[16] A. Nowara, J. Burhenne, M. Spiteller, J. Agric. Food Chem. 45 (1997)
are indicative of ligand exchange between ofloxacin and
≡AlOH or ≡AlOH+ surface sites. Decreased adsorption on
[17] H.-C.H. Lützhøft, W.H.J. Vaes, A.P. Freidig, B. Halling-Sørensen,
porous Al2O3 is postulated to result from electrostatic repul-
J.L.M. Hermens, Environ. Sci. Technol. 34 (2000) 4989.
K.W. Goyne et al. / Journal of Colloid and Interface Science 283 (2005) 160–170
[18] Ph. Schmitt-Kopplin, J. Burhenne, D. Freitag, M. Spiteller, A. Kettrup,
J.V. Ortiz, B.B. Stefanov, G. Liu, A. Liashenko, P. Piskorz, I. Ko-
J. Chromatogr. A 837 (1999) 253.
maromi, R. Gomperts, R.L. Martin, D.J. Fox, T. Keith, M.A. Al-
[19] L.M. Mayer, Chem. Geol. 114 (1994) 347.
Laham, C.Y. Peng, A. Nanayakkara, C. Gonzalez, M. Challacombe,
[20] A.R. Zimmerman, K.W. Goyne, J. Chorover, S. Komarneni, S.L. Bran-
P.M.W. Gill, B. Johnson, W. Chen, M.W. Wong, J.L. Andres, C. Gon-
tely, Org. Geochem. 35 (2004) 355.
zalez, M. Head-Gordon, E.S. Replogle, J.A. Pople, Gaussian 98 (Re-
[21] K.W. Goyne, J. Chorover, A.R. Zimmerman, S. Komarneni, S.L.
vision A.10), Gaussian, Pittsburgh, PA, 2001.
Brantley, J. Colloid Interface Sci. 272 (2004) 10.
[36] M.W. Wong, Chem. Phys. Lett. 256 (1996) 391.
[22] E. Fasani, A. Profumo, A. Albini, Photochem. Photobiol. 68 (1998)
[37] G. Schaftenaar, J.H. Noordik, J. Comput. Aided Mol. Design 14
[23] D.L. Ross, C.M. Riley, Int. J. Pharm. 63 (1990) 237.
[38] G. Sposito, The Chemistry of Soils, Oxford Univ. Press, New York,
[24] K.W. Goyne, A.R. Zimmerman, B.L. Newalkar, S. Komarneni, S.L.
Brantley, J. Chorover, J. Porous Mater. 9 (2002) 243.
[39] B. Macías, M.V. Villa, M. Sastre, A. Castiñeiras, J. Borras, J. Pharm.
[25] S. Komarneni, R. Pidugu, V.C. Menon, J. Porous Mater. 3 (1996) 99.
Sci. 91 (2002) 2416.
[26] P.T. Tanev, T.J. Pinnavaia, Science 267 (1995) 865.
[40] B.M. Sánchez, M.M. Cabarga, A.S. Navarro, A.D.G. Hurlé, Int. J.
[27] W. Zhang, T.R. Pauly, T.J. Pinnavaia, Chem. Mater. 9 (1997) 2491.
Pharm. 106 (1994) 229.
[28] W. Stumm, Chemistry of the Solid–Water Interface, Wiley, New York,
[41] S. Sagdinc, S. Bayari, J. Mol. Struct. 691 (2004) 107.
[42] R.M. Silverstein, G.C. Bassler, T.C. Morrill, Spectrometric Identifica-
[29] R.J. Umpleby, S.C. Baxter, Y. Chen, R.N. Shah, K.D. Shimizu, Anal.
tion of Organic Compounds, fifth ed., Wiley, New York, 1991.
Chem. 73 (2001) 4584.
[43] A.L. Mattioda, D.M. Hudgins, C.W. Bauschlicher Jr., M. Rosi, L.J.
[30] J.-Y. Yoon, H.-Y. Park, J.-H. Kim, W.-S. Kim, J. Colloid Interface
Allamandola, J. Phys. Chem. A 107 (2003) 1486.
Sci. 177 (1996) 613.
[44] J. Gauss, J. Chem. Phys. 99 (1993) 3629.
[31] J.-Y. Yoon, J.-H. Kim, W.-S. Kim, Colloids Surf. A 153 (1999) 413.
[45] C. Møller, M.S. Plesset, Phys. Rev. 46 (1934) 618.
[32] A.D. Becke, J. Chem. Phys. 98 (1993) 5648.
[46] B. Gu, J. Schmitt, Z. Chen, L. Liang, J.F. McCarthy, Geochim. Cos-
[33] C.T. Lee, W.T. Yang, R.G. Parr, Phys. Rev. B 37 (1988) 785.
mochim. Acta 59 (1995) 219.
[34] W.J. Hehre, R. Ditchfield, J.A. Pople, J. Chem. Phys. 56 (1972) 2257.
[47] J. Chorover, M.K. Amistadi, Geochim. Cosmochim. Acta 65 (2001)
[35] M.J. Frisch, G.W. Trucks, H.B. Schlegel, G.E. Scuseria, M.A. Robb,
J.R. Cheeseman, V.G. Zakrzewski, J.A. Montgomery Jr., R.E. Strat-
[48] K. Vermöhlen, H. Lewandowski, H.-D. Narres, E. Koglin, Colloids
mann, J.C. Burant, S. Dapprich, J.M. Millam, A.D. Daniels, K.N.
Surf. A 170 (2000) 181.
Kudin, M.C. Strain, O. Farkas, J. Tomasi, V. Barone, M. Cossi,
[49] D.L. Sparks, Environmental Soil Chemistry, Academic Press, San
R. Cammi, B. Mennucci, C. Pomelli, C. Adamo, S. Clifford, J. Ochter-
Diego, 1995.
ski, G.A. Petersson, P.Y. Ayala, Q. Cui, K. Morokuma, D.K. Mal-
[50] Y. Wang, C. Bryan, H. Xu, P. Pohl, Y. Yang, C.J. Brinker, J. Colloid
ick, A.D. Rabuck, K. Raghavachari, J.B. Foresman, J. Cioslowski,
Interface Sci. 254 (2002) 23.
Source: http://swes.cals.arizona.edu/chorover_lab/pdf_papers/Goyne_et_al_2005.pdf
Observational evidence favors a static universe David F. CrawfordSydney Institute for Astronomy,School of Physics, University of Sydney.Correspondence: 44 Market St, Naremburn, 2065,NSW, Australiaemail: [email protected] The common attribute of all Big Bang cosmologies is that they are based on the assumption that the universe is expanding. However exam-ination of the evidence for this expansion clearly favors a static universe.The major topics considered are: Tolman surface brightness, angular size,type 1a supernovae, gamma ray bursts, galaxy distributions, quasar dis-tributions, X-ray background radiation, cosmic microwave background ra-diation, radio source counts, quasar variability and the Butcher–Oemlereffect. An analysis of the best raw data for these topics shows that theyare consistent with expansion only if there is evolution that cancels theeffects of expansion. An alternate cosmology, curvature cosmology, is atired-light cosmology that predicts a well defined static and stable uni-verse and is fully described. It not only predicts accurate values for theHubble constant and the temperature of cosmic microwave backgroundradiation but shows good agreement with most of the topics considered.Curvature cosmology also predicts the deficiency in solar neutrino pro-duction rate and can explain the anomalous acceleration of Pioneer 10.
Oregon State University The Linus Pauling From the Director Balz Frei, Ph.D. LPI Director and Endowed Chair Distinguished Professor of An Interview with Biochemistry and Biophysics Sharon Krueger, Ph.D. Joan H. Facey LPI Professor Assistant Professor (Senior Research) I n my last column I told you about the strategic