Kujawa-roeleveld_2011_removal of pharmaceuticals compounds from concentrated wastewater
018530 - SWITCH
Sustainable Water Management in the City of the Future
Integrated Project Global Change and Ecosystems
Training material
Pharmaceutical compounds in environment
Removal of pharmaceuticals from concentrated
wastewater streams in source oriented sanitation
Prepared by: dr. ir. Katarzyna Kujawa-Roeleveld Wageningen University, Wageningen, The Netherlands LeAF (Lettinga Associates Foundation) Based on deliverables of SWITCH project, other overlapping projects and literature Material to be used with PowerPoint presentations I-VIII
1 Characteristics of human pharmaceuticals
Human pharmaceuticals are consumed in high quantities world wide. The consumption is in the range of tons per year per one pharmaceutical compound depending on the size of a country. The expectations are that these amounts will only keep increasing because of a improving health care system and longer life expectations of people. The diversity of the human pharmaceuticals is large. In the Netherlands, for instance, there are 12000 human pharmaceuticals approved (authorised). There are 850 active compounds in human pharmaceuticals, important fact from environmental point of view (Derksen 2004). Pharmaceuticals administered (it is a medical term, in other words consumed) by humans after required action in the body get excreted with urine and feaces as a parent (original) compound and usually as a number of metabolites. The toilet wastewater (consisting of urine and faeces flushed with clean water; often called black water) is mixed with other wastewater streams forming finally a sewage that enter the municipal sewer. In a sewage treatment plant (STP) effluents many pharmaceuticals compounds do not get removed to a sufficient degree. This is because of the configurations of the current STPs that are not efficient enough to remove these micropolllutants. Consequently they are present in the effluents of STPs, enter the surface water where they may pose effects onto aquatic life (Figure 1.1). There are evidences that they do so.
Figure 1.1: Exposure routes of human pharmaceuticals in the environment
The reason that pharmaceutical compounds in the environment has been recently deserved so much
attention is that they have been developed to perform a specific biological effect in human (and other)
organisms. Next to, they possessed several common features like e.g. polarity or persistence to
prevent their inactivation before they posed a therapeutic effect. This already implicate that these
substances will enter the aquatic and terrestrial ecosystems to bioaccumulate and provoke
environmental effects (Halling-Sorensen 1998).
General characteristics of pharmaceuticals
According to EU definition, a drug (medicinal product, pharmaceutical) is:
- any substance or combination of substances presented as having properties for treating or
preventing disease in human beings; or
- any substance or combination of substances which may be used in or administered to human
beings either with a view to restoring, correcting or modifying physiological functions by exerting a pharmacological, immunological or metabolic action, or to making a medical diagnosis (EU 2004).
According to U.S. Food and Drug Administration Centre for Drug Evaluation and Research (F.D.A. 2004) a drug is defined as:
- A substance recognized by an official pharmacopoeia or formulary. - A substance intended for use in the diagnosis, cure, mitigation, treatment, or prevention of
- A substance (other than food) intended to affect the structure or any function of the body. - A substance intended for use as a component of a medicine but not a device or a component,
part or accessory of a device.
- Biologic products are included within this definition and are generally covered by the same
laws and regulations, but differences exist regarding their manufacturing processes (chemical process vs. biological process.)
Human pharmaceuticals comprise a wide array of chemical structures answering a wide array of medical needs. Classification of pharmaceuticals is complex because different groups have different preferences for the base for classification. The following is taken into account for classification of pharmaceuticals:
-
chemical structure – chemical structure may but usually does not overlap with biological
activity of the compounds;
-
pharmacological activity – based on biological activity therapeutic groups of compounds are
distinguished containing a wide range of chemicals, usually, of different chemical structures;
-
physiological classification – based on the targeted physiological system – like e.g. central
-
receptor interaction – based on specific receptor with which they interact (e.g. beta-
blockers) (Williams 2005).
In order to measure drug use classification system and a unit of measurement were developed.
Norwegian researchers developed a system known as the Anatomical Therapeutic Chemical (ATC)
classification and a technical unit Defined Daily Dosis (DDD) used for the first time in 1976 (WHO
2006). In the ATC classification system, the drugs are divided into different groups according to the
organ or system on which they act and their chemical, pharmacological and therapeutic properties.
Drugs are classified in groups at five different levels as presented in Table 1.1.
Table 1.1: Five levels of drug classification to illustrate the structure of the code based on example drug
ibuprofen
Group/level
Musculo-skeletal system
Antiinflammatory and antirheumatic products
Antiinflammatory and antirheumatic products,
Propionic acid derivatives
Chemical substance
M01AE01 Ibuprofen
The DDD is the assumed average maintenance dose per day for a drug used for its main indication in adults. A DDD is only assigned for drugs that already have an ATC code. The DDD does not necessarily reflect the recommended or prescribed daily dose. Doses for individual patients will often
differ from the DDD and will necessary have to be based on individual characteristics (e.g. age, weight) and pharmacokinetic considerations (WHO 2006). Each pharmaceutical consists of an active pharmacological compound (usually in small quantity) and a number of help compounds to allow for medicine handling and dosing. From environmental points of view only active compounds are important.
Pharmaceutical metabolism and excretion
Drug metabolism is the metabolism of drugs, their biochemical modification or degradation, usually
through specialized enzymatic systems. Drug metabolism often converts
lipophilic chemical compounds into
more readily excreted polar products. Its rate is an important determinant of the
duration and intensity of the pharmacological action of drugs.
Drug metabolism can result in toxication or detoxication - the activation or deactivation of the
chemical. While both occur, the major metabolites of most drugs are detoxication products.
Drugs are almost all xenobiotics.
Phase I and Phase II reactions are biotransformations of chemicals that occur during drug
metabolism. Phase I metabolism usually precedes Phase II, though not necessarily (Figure 1.2 and
1.3). During these reactions, polar bodies are either introduced or unmasked, which results in (more)
polar metabolites of the original chemicals. Phase I reactions may occur by oxidation, reduction or
hydrolysis reactions. If the metabolites of phase I reactions are sufficiently polar, they may be readily
excreted at this point. However, many phase I products are not eliminated rapidly and undergo a
subsequent reaction in which an endogenous substrate combines with the newly incorporated
functional group to form a highly polar conjugate. Phase II reactions — usually known as conjugation
reactions (e.g., with glucuronic acid, sulfonates (commonly known as sulfation), glutathione or amino
acids) — are usually detoxication in nature, and involve the interactions of the polar functional groups
of phase I metabolites (Wikipedia).
Introduction of new functional groups
Conjugation reactions involving
Or modification/unmasking
Addition of functional groups
existing functional group
(acetyl, sulfate, glucuronic acid,
(oxidation, hydroxylation, reduction,
glutathione or some aminoacids)
Increasing polarity and excretability
Figure 1.2: Two phases of drug metabolism; all drugs undergo both phases; conjugation reactions can be
reversed.
Figure 1.3.: Metabolism of pharmaceutical compounds ; solid line transformation into a more water soluble
compound; doted line – reactivation of the phase II metabolites
Most of the pharmaceutical substances are metabolised to phase I or II metabolites before being
excreted. Products of phase I are often more toxic than the parent drug. Conjugates from phase II are
normally inactive. Both phases change the chemical-physical behaviour of substance; metabolites are
more soluble than parent compounds (Halling-Sorensen 1998). Attention needs to be paid therefore in
any studies on both, parent compound and metabolites.
Pharmaceuticals undergo a number of enzymatic transformations (metabolism) in human tissues including liver, intestine, kidney and lung. The main part of metabolism occurs in liver. Every drug is metabolised to different degree resulting in more polar metabolites with loss of some or all pharmacological activity of the parent substance (Williams 2005). More polar character of transformed pharmaceuticals enables their excretion, although unmetabolised compounds leave also human body. Urine and feaces are two excretion routes of pharmaceuticals. Feaces contains usually unabsorbed drugs (oral administration) or drugs metabolites excreted in the bile (Williams 2005). In Figure 1.4 a distribution of excreted 40 pharmaceutical compounds between urine and faeces is shown (Moffat et al, 2004). It can be stated generally that 30% of the compounds are excreted in faeces and 70% in the urine.
Figure 1.4: Fraction of excreted pharmaceuticals (parent compounds and metabolites) in urine and faeces for
selected compounds (Moffat et al., 2004).
Commonly, glucuronide and sulphate conjugates of the parent drugs are the major excreted
metabolites. It is supposed that glucuronide and sulphate conjugates may be at least partially
hydrolysed in sewage, thus effectively increasing the excreted contribution to sewage concentrations
of the parent drugs (Ternes 1998).
Sources of emission of pharmaceutical compounds
Emission routes of pharmaceuticals to water environment have a very diffuse character:
- production (cleaning processes in pharmaceutical industry) - consumption in household or hospital - not-consumed pharmaceuticals flushed in toilet - effluent of a WWTP
1.4.1 Patients excretion
It is generally accepted that the principal source of human pharmaceuticals detected in the aquatic
environment is patient excretion. The most important is on-house consumption. Humans excrete
pharmaceuticals via urine and faeces. Pharmaceuticals are often excreted only slightly transformed or
even unchanged mostly conjugated to polar molecules (e.g. as glucoronides. These conjugates can be
easily cleaved during sewage treatment and the original PhAC will then be released into the aquatic
environment (Heberer 2002).
The ‘typical' wastewater from a residential area contains, conform domestic consumption, pain
killers, beta-blockers, cholesterol lowering agents and anti-epileptics in concentrations up to tens of
µg/L. Antibiotics, anastatics and X-ray contrast media were also detected but in much lower
concentrations. Detected concentrations in the effluents from pharmaceutical industry and hospitals
differ a lot concerning a type of pharmaceutical as well as its concentration. In general wastewater
from hospital contains high concentrations of X-ray contrast media (in mg/L) and antibiotics, different
than those used in a household (µg/L).
Disposal from pre-patient supply and unused pharmaceuticals
Handling pharmaceutical products to be disposed, because of, e.g., the expiration date has passed, is controlled. Expired products are commonly returned to the manufacturing company. Expired products are then usually destructed via incineration. In this way disposal of undistributed or outdated products is unlikely to be a source of pharmaceuticals detected in the environment (Williams 2005). Patient disposal of unused, outdated or sold over-the-counter pharmaceuticals can be into either domestic wastewater or solid waste. Only limited data is available on the magnitude of this emission source. According to (Kummerer, 2004 ) between 25% (in Germany) and 33% (Austria) sold drugs are disposed to household waste(water) streams. Disposal to wastewater is believed to be an emission form but is not dominant (Heberer 2002), (Williams 2005). Disposed pharmaceuticals are not modified by human metabolism prior entering wastewater. Disposal to solid waste. Residential solid waste is either incinerated or disposed via landfill. It is not clear whether biodegradation of pharmaceutical compounds occurs in bioreactive landfills. Excess leachate from landfills that may contain pharmaceuticals may be disposed to WWTP.
1.4.3 Pharmaceutical industry
Discharges from manufacturing facilities are not believed to contribute significantly to the overall
emission. Discharge of active pharmaceutical ingredient via waste stream is generally avoided since it
constitutes a valuable product. A common practice in pharmaceutical industry is recovery and reuse of
active ingredients, otherwise treatment and disposal via incineration is applied. A number of
manufacturing sites is relatively small in the world and they are usually concentrated in specific
regions. Also there are very few facilities for a specific active ingredient. When active ingredients are
blended with some help substances (starch, lactose) some solid waste stream may be produced, that is
commonly incinerated (Williams 2005).
Variety of pharmaceutical compounds
There are 14 main groups of human pharmaceutical substances as shown in Table 1.2.
Table 1.2: Main groups of human pharmaceuticals (WHO 2006)
Number of pharmacological therapeutic
A Alimentary tract and metabolism
B Blood and blood forming organs
5 (B01-B06) no B04
C Cardiovascular system
9 (C01-C10) no C06
D Dermatologicals
G Genito urinary system and sex hormones
Excl. sex hormones and
H Systematic hormonal preparations
J Antiinfectives for systematic use
6 (J01-J07) no J03
L Antineoplastic and immunomodulating
agents M Musculo-skeletal system
6 (M01-M09) no M6,7,8
N Nervous system
P Antiparasitic agents, insecticides,
repellents R Respiratory system
6 (R01-R07) no R4
S Sensory organs
9 (V01, 03,04,06,07-09,10,20)
Some of important groups, those used in highest quantities, are described to more detail in paragraphs from 1.5.1. to 1.5.7.
Group A: alimentary tract and metabolism
This group comprises the largest number of the subgroups: A01 - stomatological preparations; A02 Drugs for acid related disorders, A03 Drugs for functional gastrointestinal disorders, A04 Antiemetics and antinauseants, A05 Bile and liver therapy, A06 Laxatives , A07 Antidiarrheals, intestinal anti-inflammatory/anti-infective agents, A08 Antiobesity preparations, excluding diet products, A09 Digestives, including enzymes, A10 Drugs used in diabetes, A11 Vitamins, A12 Mineral supplements, A13 Tonics, A13A tonics, A14 Anabolic agents for systemic use, A15 Appetite stimulants, A16 Other alimentary tract and metabolism products. Pharmaceuticals belonging to this ATC group will be further not subjected to analysis within this part of the project.
Group B: blood and blood forming organs
Medicines from this group are applied to fight against diseases of blood and blood forming organs, like iron deficiency anemias, other deficiency anemias, hereditary hemolytic anemias, acquired hemolytic anemias, aplastic anemia, other and unspecified anemias, coagulation defects, purpura and other hemorrhagic conditions, diseases of white blood cells, other diseases of blood and blood-forming organs. The pharmaceuticals from this group will be further not investigated within this project.
Group C: cardiovascular system
Beta blockers (β-blockers) are a class of drugs used in the highest quantities within ATC group C
used for various indications, but particularly for the management of cardiac arrhythmias and
cardioprotection after myocardial infarction. Beta blockers are pharmaceuticals designed to block the
β1–receptor from stimulating the higher hart rate and the cardiac output in humans with mainly
cardiovascular diseases, like hypertension and angina pectoris, but also some other diseases like
migraine, thyrotoxicoses and the control of tremors. Some beta blockers have a high first pass
metabolism, while others are excreted unchanged in the urine. Most of the generic names for beta
blockers end with
"olol"(e.g. sotalol, timolol, esmolol, carteolol, carvedilol, nadolol, propranolol,
propranolol, betaxolol, penbutolol, metoprolol, acebutolol, atenolol, metoprolol, labetalol, pindolol,
bisoprolol, Table 1.3).
Table 1.3: Examples of selective and non-selective beta-blockers and their metabolism
Selective beta blockers
Metoprolol
Extensively metabolised in the liver, so that only 5 % is excreted by the kidney as the parent compound. The plasma half live is 3-4 hours and to prolong the plasma half-life, extended release tablets are developed. It has a first pass effect of 50 %. In the liver metoprolol is metabolised by the cytochrome P450 isoenzyme cyp2D6. It also undergoes oxidative deamination, O-dealkylation followed by oxidation and aliphatic hydroxylation. The metabolites are also excreted in urine.
Atenolol
Metabolism in the body is different than of metoprolol. Only 50 % is absorbed in the intestine and with food this decreases with 20 %. From the 50 %, which is absorbed only 10 % is metabolised in the liver. The drug is excreted in the urine. It has a plasma half life of 7-8 hours. And the maximum plasma concentration is reached after 2-4 hours.
Bisprolol
After oral intake it is absorbed in the gastro intestinal tract and approximately 90 % is bio available in the body. It is metabolised in the liver. 50 % is excreted as parent compound in the urine and the other 50 % as inactive metabolites.
Selective beta blockers
Propranolol
It binds to both α- and β-adrenoreceptors. It has a high first pass metabolism and is subject to hepatic tissue binding. The maximum concentration in the plasma is reached after 1-2 hours. In the blood 80-90 % is bound to plasma proteins, so only 10-20 % of the absorbed and metabolised drug can cause effects. The plasma half live is 3-6 hours . Propranolol has high lipophilic solubility and passes the blood-brain barrier, placenta and is distributed in milk (EU 2004). Less than 1 % is excreted as the parent compound and 90 % is excreted as a metabolite in urine. Three primary pathways of metabolism of propranolol are described. 41 % is metabolised through a side-chain oxidation, 17 % through glucuronic acid conjugation and the other route is ring oxidation. Another route of metabolism, which is of little importance, is the O-dealkylation. Recently it was discovered that the cytochrome P450 isoenzymes, CYP1A2 catalyses the oxidative metabolism of propranolol. 4-hydroxypropranolol is a ring hydroxylated metabolite and is biologically active. 4-hydroxypropranolol and propranolol are formed in the same amount in the liver after oral administration, but this metabolite isn't excreted in urine and has a lower plasma half live as propranolol.
It has low lipid solubility, but is absorbed almost for 100 % in the intestine. A very little amount is metabolised and all is excreted unchanged in the urine. It is given in racemic mixtures of two stereoisomers
d-sobatol and
l-sobatol. Unlike the
disomers of the other beta blocking drugs,
d-sotalol has arrhythmic properties. The plasma half life is 10-20 hours.
Incompletely absorbed in the gastro-intestinal system after oral administration. It does appear not to be metabolised and is excreted in urine. It has a plasma half-life of 12-24 h. It has a low lipid solubility.
Lipid-lowering drugs reduce serum cholesterol levels by inhibiting a key enzyme involved in the
biosynthesis of cholesterol; examples:
- Resins cholestyramine (Cholybar, Questran) colestipol (Colestid) - HMG CoA Reductase Inhibitors lovastatin (Mevacor) pravastatin (Pravochol) simvastatin
- Fibric Acid Derivatives gemfibrozil (Lobid) clofibrate (Atromid-S) - Miscellaneous nicotinic acid (Niacin) probucol (Lorelco)
Group J: antibiotics
Antibiotic are widely used to treat many bacterial infections. Bacteria are classified as either Gram-positive or Gram-negative. They differ in several respects, especially in the structure of the cell wall, which has implication for the action of the antibiotics. The cell wall of the Gram-positive bacteria is a relatively simple structure, while the cell wall of Gram-negative organisms is more complex. Some of the antibiotic classes, such as macrolides, show difficulty in penetrating the complex outer layer of Gram-negative bacteria. There are three proven targets for the main antibacterial drugs: (1) bacterial cell-wall biosynthesis; (2) bacterial protein synthesis; and (3) bacterial DNA replication and repair. Phenoxymethylpenicillin and amoxicillin inhibits the cell wall synthesis; Tetracycline, erythromycin and clarithromycin inhibits the protein synthesis and trimetoprim and ciprofloxacin inhibits the nuclei acid synthesis. The antibiotic compounds will be further not subject of this study.
Group N: nervous system
Sedatives, tranquilizers, depressants, anxiolytics, soporifics, sleeping pills, downers, or sedative-hypnotics these are different names for substances, which depress the central nervous system (CNS), resulting in calmness, relaxation, reduction of anxiety, sleepiness, slowed breathing, slurred speech, staggering gait, poor judgment, and slow, uncertain reflexes. At high doses or when they are abused, many of these drugs can cause unconsciousness and death (Wikipedia). A depressant is a chemical agent that diminishes the function or activity of a specific part of the body. The term is used in particular with regard to the CNS. In that case these chemicals are known as neurotransmitters. Depressants intended to act on the CNS do so by increasing the activity of a particular neurotransmitter known as gamma-aminobutyric acid (GABA). GABA's task is to calm the CNS and to promote sleep. Drugs that stimulate the production of this amino acid produce slowed brain activity and a drowsy or calm feeling, and so depressants are generally prescribed to relieve symptoms of anxiety or insomnia. Internal systems regulate the body's production of GABA, but when medication is taken to stimulate GABA production, it is possible to induce hazardously high levels, which can dangerously slow breathing and heart rates, and may result in death.
An
antidepressant is a medication designed to treat or alleviate the symptoms of clinical depression.
Some antidepressants are also used to help one sleep, to treat anxiety, and to relieve certain types of
pain. Other antidepressants, notably the tricyclics, are commonly used off-label in the treatment of
neuropathic pain, whether or not the patient is depressed. Smaller doses are generally used for this
purpose, and they often take effect more quickly. Many antidepressants also are used for the treatment
of anxiety disorders and tricyclic antidepressants are used in the treatment of chronic pain disorders
such as chronic functional abdominal pain (CFAP), myofascial pain syndrome, and post-herpetic
neuralgia.
Like many psychiatric drugs, antidepressants were discovered by accident. The first useful
antidepressants belonged to a group called MAOIs (MonoAmine Oxidase Inhibitors) and were
discovered in the early 1950s. The original member of this group was iproniazid, which was originally
developed to treat tuberculosis. The next group were the tricyclic antidepressants. The first was
imipramine. They were effective and safer than the MAOI but still quite dangerous in overdose. They
are still used today but have been largely replaced by another group: SSRIs (Selective Serotonin
Reuptake Inhibitors). The first SSRI was fluoxetine (better known as Prozac). Drugs from all three
groups have been found to improve the mood of depressed patients. The SSRI antidepressants were
early examples of rational drug design.
TCA's have increasingly been replaced by selective serotonin reuptake inhibitors (SSRI's), serotonin
and noradrenaline reuptake inhibitors (SNRI's) and other newer antidepressants. SSRIs are prescribed
for anxiety disorders, obsessive-compulsive disorder, and eating disorders. They are also sometimes
prescribed to treat irritable bowel syndrome. SNRI's are used in the treatment of depression and other
affective disorders. They are also sometimes used to treat anxiety disorders, obsessive-compulsive
disorder, attention deficit hyperactivity disorder (ADHD) and chronic neuropathic pain. Some ‘well-
known' antidepressants examples are given in Table 1.4:
Table 1.4: Examples of anti-depressants
Compound
Brand names
Prozac, Sarafem, Fluctin, Fontex, Prodep, Fludep, Lovan
Zoloft, Lustral, Apo-Sertral, Asentra, Gladem, Serlift, Stimuloton
Celexa, Cipramil, Talohexane
Paxil, Seroxat, Aropax
Lexapro, Cipralex
and Wellbutrin, Zyban
Dothiepin (Dosulepin)
Prothiaden, Dothapax
Depressants generally fall into two classes, barbiturates and benzodiazepines, but also include narcotics (or opioids) and sedative-hypnotics. Also there are tranquilizers. Barbiturates are effective in relieving the conditions they are designed to address; they are also readily abused, and when, in the late 1960s, it became clear that the social cost of barbiturates was beginning to outweigh the medical benefit, a serious search began for a replacement drug. Most people still using barbiturates do so to prevent seizures.
Benzodiazepines mediate the same symptoms as barbiturates, but without the same degree of toxic
hazard. This is not to say they are not without their own risks; where barbiturates pose a greater
"front-end" risk in that overdose or drug/alcohol interactions may result in fatality, benzodiazepines
pose a greater "back-end" risk in the possibility of addiction and serious physical and psychological
withdrawal symptoms. Even so, any suggestion that it is safe to consume alcohol while using
benzodiazepines, or to attempt to stop barbiturate use "cold turkey" is foolish in the extreme.
Barbiturates are drugs that act as central nervous system (CNS) depressants, and by virtue of this they
produce a wide spectrum of effects, from mild sedation to anesthesia. Some are also used as
anticonvulsants. Barbiturates are believed to be GABA (gamma-aminobutyric acid) agonists, acting
on the GABA-A receptor. GABA is the principal inhibitory neurotransmitter in the mammalian CNS.
Barbiturates are derivatives of barbituric acid.
The benzodiazepines as minor tranquilizers are a class of drugs with sedative, hypnotic, anxiolytic,
anticonvulsant, amnestic and muscle relaxant properties. Benzodiazepines are often used for short-
term relief of severe, disabling anxiety or insomnia. Long-term use can be problematic due to the
development of tolerance and dependency. They are believed to act on the GABA receptor GABAA,
the activation of which dampens higher neuronal activity. They began to be widely prescribed for
stress-related ailments in the 1960s and 1970s. Their chemical structure is based upon diazepine and
phenyl groups. Examples: Alprazolam, Bromazepam, Chlordiazepoxide, Cinolazepam, Clonazepam
Clorazepate Diazepam Flunitrazepam Flurazepam Halazepam Ketazolam Loprazolam Lorazepam
Lormetazepam Medazepam Nobrium Midazolam Nitrazepam Mogadon Nordazepam Oxazepam
Prazepam Quazepam Temazepam Tetrazepam Triazolam DMCM.
The term
antipsychotic is applied to a group of drugs used to treat psychosis. Common conditions
with which antipsychotics might be used include schizophrenia, mania and delusional disorder,
although antipsychotics might be used to counter psychosis associated with a wide range of other
diagnoses. Antipsychotics also have some effects as mood stabilizers, leading to their frequent use in
treating mood disorder (particularly bipolar disorder) even when no signs of psychosis are present.
Some antipsychotics (haloperidol, pimozide) are used to treat Tourette syndrome.
Antipsychotics are also referred to as neuroleptic drugs, or simply neuroleptics. There are currently
two main types of antipsychotics in use, the typical antipsychotics and atypical antipsychotics. A new
class of antipsychotic drugs has recently been discovered, known as dopamine partial agonists.
Clinical development has progressed rapidly on partial dopamine agonists, and one drug in this class
(aripiprazole) has already been approved by the Food and Drug Administration. Although the
underlying mechanism of this new class is different from all previous typical and atypical
antipsychotics, dopamine partial agonists are often categorized as atypicals.
Typical antipsychotics are sometimes referred to as major tranquilizers, because some of them can
tranquilise and sedate. This term is increasingly disused because many newer antipsychotics do not
have strong sedating properties and the terminology implies a connection with benzodiazepines,
whereas none exists.
Further there are within this group -
Atypical antipsychotic (also known as second generation
antipsychotics) are a class of prescription medications used to treat psychiatric conditions; All atypical
antipsychotics are FDA approved for use in the treatment of schizophrenia. Some carry FDA
approved indications for acute mania, bipolar mania, psychotic agitation, bipolar maintenance, and
other indications; clozapine (Clozaril), quetiapine (Seroquel) , Risperidone (Risperdal) , Ziprasidone
(Geodon). It may make some people tired, while making others unable to sleep olanzapine (Zyprexa)
Group pain relievers, antiphlogistics, analgesics, anti-
inflammatories, non-steroidal drugs
An
analgesic (colloquially known as a painkiller) is any member of the diverse group of drugs used to
relieve pain and to achieve analgesia. Analgesic drugs act in various ways on the peripheral and
central nervous system; they include paracetamol (acetaminophen), the nonsteroidal anti-
inflammatory drugs (NSAIDs) such as the salicylates, narcotic drugs such as morphine, synthetic
drugs with narcotic properties such as tramadol, and various others. Some other classes of drugs not
normally considered analgesics are used to treat neuropathic pain syndromes; these include tricyclic
antidepressants and anticonvulsants.
Antiphlogistic drugs and pain killers are agents, which are applied in medical therapy for relieving
pains, fevers and against inflammatory caused by various diseases. They are substances used in many
non-prescription drugs in the primary health sector. In the current context, antiphlogistic drugs and
pain killers are chosen for a separate section because of their widespread application in high doses
make up the largest tonnage of one group of pharmaceuticals.
Non-steroidal anti-inflammatory drugs, usually abbreviated to
NSAIDs, are drugs with analgesic,
antipyretic and anti-inflammatory effects - they reduce pain, fever and inflammation. The term "non-
steroidal" is used to distinguish these drugs from steroids, which (amongst a broad range of other
effects) have a similar eicosanoid-depressing, anti-inflammatory action. NSAIDs are sometimes also
referred to as non-steroidal anti-inflammatory agents/analgesics (NSAIAs). The most prominent
members of this group of drugs are aspirin and ibuprofen. Paracetamol (acetaminophen) has
negligible anti-inflammatory activity, and is strictly speaking not an NSAID.
Beginning in 1829, with the isolation of salicylic acid from the folk remedy willow bark, NSAIDs
have become an important part of the pharmaceutical treatment of pain (at low doses) and
inflammation (at higher doses). Part of the popularity of NSAIDs is that, unlike opioids, they do not
produce sedation, respiratory depression, or addiction. NSAIDs, however, are not without their own
problems. Certain NSAIDs, including ibuprofen and aspirin, have become accepted as relatively safe
and are available over-the-counter without prescription.
Group V: contrast media
Radiocontrast agents (or simply contrast agents) are compounds used to improve the visibility of internal bodily structures in an X-ray image. Iodinated contrast agents contain iodine, which enhances the visibility of vascular structures and organs during radiographic procedures.
Iodinated contrast media may either be oil-based or water-soluble, the former of which is slowly absorbed by body tissue and is usually only used in sialographic and hysterosalpingographic examinations. Water-soluble iodinated medium, which is more quickly absorbed, may be used in place of barium sulfate for gastrointestinal studies that are contraindicated by the use of barium for that reason. Contrast media are highly persistent. They will be further not subjected to further study within this project.
Properties of pharmaceuticals
Pharmaceuticals are compounds characterised by complex chemical structures. Most pharmaceuticals are charged and hydrophilic. Many pharmaceuticals have multiple ionisable functional groups. The hydrophobic reactions dominating partitioning neutral organic compounds to sediments and suspended solids (limited sorption properties) are relatively unimportant for most of the pharmaceuticals. Many pharmaceutical are chiral and often administered as racemic mixtures (Williams 2005). Properties of few pharmaceutical groups relevant for their behaviour in environment are given below.
Antibiotics
(Al-Ahmad 1999)investigated biodegradability of some clinically important antibiotics, Cefotiam,
Ciprofloxacin, Meropenem, Penicillin G, and Sulfamethoxazole, in the closed bottle test (CBT).
These drugs possessed different chemical structures and mode of action (= antibiotic spectra). None of
the investigated antibiotics was readily biodegradable (Table 1.6.1). Low biodegradation rates were
also reported in soil. Adaptation of microorganisms was not concluded. Penicillin seems to be easier
biodegradable than the rest of tested antibiotic compounds. These finding were in agreement with
reported poor biodegradability in soils. These on the other hand could have been caused by adsorption
resulting in poor bioavailability. Authors concluded that biodegradation of antibiotics in STPs might
not be a reliable expectation for the removal of antibiotic substances. The CBT was a screening test
using low bacteria density. In tests with higher bacteria density (biodegradation tests) or a higher
degree of simulating an STP, higher biodegradability and nonbiotic elimination processes like
adsorption, hydrolysis, or partial degradation of active moieties may take place in a higher extension,
but not necessarily.
Tabel 1.5: Results of the closed bottle test (OECD 301 D) (Al-Ahmad 1999)
Group β
-blockers, lipid lowering agents
High solubility (metoprolol >1000ppm) or moderate solubility (atenolol, propranolol 10-1000 ppm) and low logKow (<3) correspond to a high affinity of β-blockers to water. The presence of beta blockers in the gaseous compartment is neglectable due to its low vapour pressure.
In a study by (Black 2004), three selective serotonin reuptake inhibitors (SSRI's): paroxetine, sertraline and fluvoxamine, were tested for biodegradability using activated sludge inoculum from a waste water treatment plant. No degradation was observed during a test period of 28 days. It was concluded that none of the compounds could be labelled readily biodegradable in waste water treatment plants. (Cunningham, Constable et al. 2004) found in a preliminary biodegradation study
with sewage biomass a rapid depletion of paroxetine from solution over the first day of the studies followed by no further depletion despite culture acclimations and enrichments. In extensive aerobic biodegradability studies no depletion of paroxetine was observed in the period after sorption had reached equilibrium. The antidepressants are not volatile from water or they have a very slow rate of volatilisation as Henry constants are low, KH < 1.3·10-7 atm·m3 ·mole-1. They have rather little mobility or are immobile in soil and will most likely sorb to soil and sludge. It is expected that the compounds tend to bioaccumulate as logKOW values are around 3 and higher. In a study performed by (Brooks, Turner et al. 2003) fluoxetine, sertraline and the metabolites norfluoxetine and desmethylsertraline were detected at levels greater than 0.1 ng/g in all tissues examined from fish residing in a municipal effluent-dominated stream.
Pain relievers, antiphlogistics, analgesics, anti-
inflammatories, non-steroidal drugs
Salicilic acid found to be easily biodegraded. Higher degradability of paracetamol can be assumed (Henschel, 1997). Zwiener et al, (2000) found a high degree of degradation for ibuprofen in the oxic biofilm reactor, which was attributed to adaptation of the biofilm to the residue (Zwiener, 2000). Two metabolites could be identified on the basis of their mass spectra and comparison with literature data, viz. hydroxyibuprofen and carboxyibuprofen.
Quantities of pharmaceuticals used (Dutch situation)
Pharmaceuticals for human treatment are used in high quantities. The consumption and abundance of
pharmaceutical compounds differ per country. The global consumption of pharmaceuticals used by
humans is predicted as 100,000 tons per year. This number corresponds to a worldwide average pro
capita consumption of 15 g.cap-1.a-1 (Ternes 2006), (Kummerer 2004).
The consumption of all therapeutic groups of pharmaceuticals in the Netherlands in years 2001 till
2005 expressed in number of users is given in Table 1.6.
Table 1.6: Users per ATC group of pharmaceuticals (* 1000) in the Netherlands (CVZ 2006)
ATC group
A Alimentary tract and metabolism
B Blood and blood forming organs
C Cardiovascular system
D Dermatologicals
G Genito urinary system and sex hormones
H Systematic hormonal preparations
J Antiinfectives for systematic use
L Antineoplastic and immunomodulating agents
M Musculo-skeletal system
N Nervous system
P Antiparasitic agents, insecticides, repellents
R Respiratory system
S Sensory organs
Group A, C, D, G, J, M, N and R are characterised by the highest number of users, between 2,5 and 4 mln people per ATC group. In Table 1.7 number of DDDs are listed per ATC main group. The prevailing groups are then A, B, C, D, N and R.
Table 1.7: Amount of DDDs (* 1000) used in The Netherlands in years 2001-2006 (CVZ 2006)
ATC group
A Alimentary tract and metabolism
792.040 839.970 897.320 828.640 924.510
B Blood and blood forming organs
398.190 416.550 441.640 433.180 448.140
C Cardiovascular system
1.592.100 1.713.300 1.870.900 2.047.900 2.190.500
D Dermatologicals
477.450 495.190 522.350 472.220 486.990
G Genito urinary system and sex hormones
798.290 790.670 799.100 277.720 284.000
H Systematic hormonal preparations
107.930 113.920 120.190 125.290 129.590
J Antiinfectives for systematic use
L Antineoplastic and immunomodulating agents
M Musculo-skeletal system
235.650 241.730 256.170 250.080 238.240
N Nervous system
642.170 670.150 699.110 686.140 691.350
P Antiparasitic agents, insecticides, repellents
R Respiratory system
586.760 592.720 582.280 565.320 568.760
S Sensory organs
198.390 208.450 220.610 220.300 222.910
In Table 1.8 top ten of the most often prescribed specific compounds in year 2005 in Netherlands is
given.
Tabel 1.8: Top 10 prescribed pharmaceuticals 2005 (CVZ 2006)
ATC code, active compound
Number
prescribed
C07AB02 Metoprolol
angina pectoris and high
N05BA04 Oxazepam
N05CD07 Temazepam
M01AB05 Diclofenac
B01AC06 Acetylsalicilic acid
Blood plasma, inhibition
A02BC01 Omeprazol
B01AC08 Carbasalaatcalcium
Blood plasma, inhibition
C10AA01 Simvastatine
Decreasing cholesterol
A10BA02 Metformine
H03AA01 Levothyroxine
To enhance thyroid hormone
In the following an example of a procedure is shown of selecting pharmaceutical compounds for the
further study (laboratory phase). The C group (cardiovascular system) was chosen as the
pharmaceuticals used for cardiovascular system are consumed by a large part of population in high
quantities. Within this group there are 9 subgroups (Table 1.9); and pharmaceuticals from 6 subgroups
are used in the highest quantities – above 0.5 mln of users.
Table 1.9: Subgroups of ATC C group and their consumption between 2001 and 2005 (in number of users)
C01 Cardiac therapy
C02 Antihypertensives
C04 Peripheral vasodilators
C05 Vasoprotectives
C07 Beta-blocking agents
1.327.000 1.368.000
C08 Calcium channel blockers
C09 ace inhibitors
1.177.000 1.268.000
C10 Lipid modifying agents
1.046.000 1.160.000
In Table 1.10 specific pharmaceuticals used in the highest quantities in numbers of users and number
of DDDs consumed (2005) (CVZ 2006)
Table 1.10: Consumption and environmental load (mass of the sold products) of 5 pharmaceuticals from ATC
group C
Env. load, t/year
C07AB02 Metoprolol
C10AA01 Simvastatine
C10AA05 Atorvastatine
C03AA03 Hydrochloorthiazide
C03CA01 Furosemide
Among the pharmaceutical compounds used for cardiovascular system, metroprolol, simvastatine, atorvastatine, hydrochlorothiazide and simvastatine are the compounds used by a largest number of people. Considering the total number of Daily Defined Doses (DDD) sold as well as an individual DDD (mg.person-1.d-1), the total load of sold compounds was calculated (Figure 1.5). Metroprolol is consumed in the highest quantity.
C07AB02 Metoprolol
C10AA01 Simvastatine C10AA05 Atorvastatine
C03CA01 Furosemide
Hydrochloorthiazide
Pharmaceuticals group
Figure 1.5: Yearly Consumption of mostly used pharmaceuticals for cardiovascular system in The Netherlands
in 2005 (CVZ 2006)
In another pharmaceuticals group of musculo-skeletal system (group M), anti-inflamatories and anti-
rheumatic agents are used by the largest number of people (almost 3 mln in 2005 (CVZ 2006)).
Diclofenac (acetic acid derivative) was used by 1.4 mln of people, followed by ibuprofen and
naproxen (propionic acid derivatives). The yearly consumption of mentioned compounds in shown in
Table 1.11 and Figure 1.6.
Table 1.11: Specific pharmaceuticals used in the highest quantities in numbers of users and number of DDDs
consumed (2005); ATC group M (CVZ 2006)
M01AB05 Diclofenac
M01AE01Ibuprofen
M01AE02 Naproxen
1) Assumption: administered is excreted for 100% as a parent compound
M01AB05 Diclofenac
Pharmaceuticals group
Figure 1.6: Yearly Consumption of mostly used pharmaceuticals for musculo-skeletal system in The
Nethelands in 2005 (CVZ 2006)
For comparison consumption of certain specific pharmaceutical compounds in Denmark is given in
Table 1.12.
Tabel 1.12: Consumption of specific substances and therapeutic groups in Denmark (5.2 mln inhabitants in
1995) of some pharmaceutical substances (Halling-Sorensen 1998)
substances
DDD per jaar
DDD grams
Applied weight, tones
(millions)
Single substances
Therapeutic groups
Analgesics (NSAID)
Literature
(EU 2004). Directive 2004/27/EC of the European Parliament and of the Council of 31 March 2004 amending
Directive 2001/83/EC on the Community code relating to medicinal products for human use (Text with
EEA relevance). Official Journal L 136, 30/04/2004 P. 0034 - 0057.
32004L0027.
Al-Ahmad, A., Daschner, F.D. and Kummerer, K. (1999). "Biodegradability of cefotiam, ciprofloxacin,
meropenem, penicillin G. and sulfamethoxazole and inhibition of waste water bacteria." Archives of
Environmental Contamination and Toxicology
37: 158-163.
Black, M., Armbrust, Kevin, Henry, Theodore B., Kwon, Jeong-Wook (2004). The Environmental Occurrence,
Fate, and Ecotoxicity of Selective Serotonin Reuptake Inhibitors (SSRIs) in Aquatic Environments, Progress Report.
Boxall, A. B. A. (2004). The environmental side effects of medication How are human and veterinary medicines
in soils and water bodies affecting human and environmental health? EMBO reports. E. M. B. O. (EMBO).
Brooks, B. W., P. K. Turner, et al. (2003). "Waterborne and sediment toxicity of fluoxetine to select organisms."
Chemosphere
52(1): 135.
Carballa, M., F. Omil and J.M. Lema (2003). "REMOVAL OF PHARMACEUTICALS AND PERSONAL
CARE PRODUCTS (PPCPS) FROM MUNICIPAL WASTEWATERS BY PHYSICO-CHEMICAL
PROCESSES." Electron. J. Environ. Agric. Food Chem.
2 (2): 309-313.
Carballa, M., Omil, F.,*, Juan M. Lema, Mar!ıa Llompart, and I. R. Carmen Garcıa-Jares, Mariano Gomez,
Thomas Ternes (2004). "Behavior of pharmaceuticals, cosmetics andhormones in a sewage treatment
plant." Water Research
38: 2918–2926.
A M A R I, † A N D E T T O R E Z U C C A T O *, ‡ (2006). "Removal of Pharmaceuticals in
Sewage Treatment Plants in Italy." Environ. Sci. Technol.
40: 357-363.
Clara, M., B. Strenn, et al. (2004). "Comparison of the behaviour of selected micropollutants in a membrane
bioreactor and a conventional wastewater treatment plant." Water Science And Technology
50(5): 29-
36.
Clara, M., Strenn, B. and Kreuzinger, N. (2004). "Carbamazepine as a possible anthropogenic marker in the
aquatic environment: investigations on the behaviour of Carbamazepine in wastewater treatment and
during groundwater infiltration." Water Research
38: 947–954.
Clara, M., Strenn, B., Gans, O., Martinez, E., Kreuzinger, N. and Kroiss, H. (2005). "Removal of selected
pharmaceuticals, fragrances and endocrine disrupting compounds in a membrane bioreactor and
conventional wastewater treatment plants." Water Research
39: 4797-4807.
Cunningham, V. L., D. J. Constable, et al. (2004). "Environmental Risk Assessment of Paroxetine." Environ.
Sci. Technol.
38(12): 3351-3359.
CVZ. (2006). "Farmacotherapeutisch Kompas." Daughton, C. G. a. J.-L., T. (eds) (2001). Pharmaceuticals and Personal Care Products in the Environment: Scientific and Regulatory Issues. C. G. a. J.-L. Daughton, T. Washington, D.C., 2001, American Chemical
Derksen, J. G. M., Rijs, G.B.J. and Jongbloed, R.H. (2004). "Diffuse pollution of surface water by
pharmaceutical products." Water Science and Technology
49(3): 213-221.
F.D.A., U. S. (2004). "Glossary of Terms." from http://www.fda.gov/cder/drugsatfda/glossary.htm#F. Gobel, A., A. Thomsen, et al. (2005). "Extraction and determination of sulfonamides, macrolides, and
trimethoprim in sewage sludge." Journal of Chromatography A
1085(2): 179.
Golet, E. M., A. Strehler, et al. (2002). "Determination of Fluoroquinolone Antibacterial Agents in Sewage
Sludge and Sludge-Treated Soil Using Accelerated Solvent Extraction Followed by Solid-Phase
Extraction." Anal. Chem.
74(21): 5455-5462.
Halling-Sorensen, N. N., S., Lanzky, P. F., Ingerslev, F., Holten Liitzhoft, H.C. and S.E. Jorgensen (1998).
"Occurrence, Fate and Effects of Pharmaceutical Substances in the Environment- A Review."
Chemosphere
Vol. 36(No.2): 357-393.
Heberer, T. (2002). "Occurrence, fate, and removal of pharmaceutical residues in the aquatic environment: A
review of recent research data." Toxicology Letters Shannon. [print] May 10th
131(1-2): 5-17.
Heberer, T., K. Reddersen, et al. (2002). "From municipal sewage to drinking water: fate and removal of
pharmaceutical residues in the aquatic environment in urban areas." Water Science and Technology.
[print]
46(3): 81-88.
Heberer, T., Verstraeten, I.M., Meyer, M.T., Mechlinski, A., Reddersen, K. "Occurence and fate of
pharmaceuticals during bank filtration - preliminary results from investigations in Germany and the United States."
Huggett, D. B., I. A. Khan, et al. (2003). "Determination of beta-adrenergic receptor blocking pharmaceuticals
in United States wastewater effluent." Environmental Pollution
121(2): 199-205.
Joss, A. (2005). "Removal of pharmaceuticals and fragrances in biological wastewater treatment (vol 39, pg
3139, 2005)." Water Research
39(18): 4585-4585.
Kalsch, W. (1999). "Biodegradation of the iodinated X-ray contrast media diatrizoate and iopromide." The
Science of the Total Environment
225: 143-153.
Khan, S. J. a. O., J.E. (2002). "Estimation of pharmaceutical residues in primary and secondary sewage sludge
based on quantities of use and fugacity modelling." Water Science and Technology
46(3): 105–113.
Kolpin DW, F. E., Meyer MT, Thurman EM, Zaugg, S.D., Barber, LB, Buxton, HT (2002). "Pharmaceuticals,
Hormones, and Other Organic Wastewater Contaminants in U.S. Streams, 1999-2000: A National
Reconnaissance." Environ. Sci. Technol.
36: 1202-1211.
Kummerer, K. (2004). Pharmaceuticals in the environment-Sources, fate, effects and risks. Kümmerer, K. A. A., A. Mersch Sundermann, V. (2000). "Biodegradability of some antibiotics, elimination of
the genotoxicity and affection of wastewater bacteria in a simple test." Chemosphere-. April, 2000;
40(7): 701-710.
Loraine, G. A. and M. E. Pettigrove (2006). "Seasonal Variations in Concentrations of Pharmaceuticals and
Personal Care Products in Drinking Water and Reclaimed Wastewater in Southern California."
Environ. Sci. Technol.
40(3): 687-695.
Miao, X. S., Y ang, J.J. and Metcalfe, C.D. (2005). "Carbamazepine and Its Metabolites in Wastewater and in
Biosolids in a Municipal Wastewater Treatment Plant." Environ. Sci. Technol.
39: 7469-7475.
Mohle, E., C. Kempter, et al. (1999). "Examination of the degradation of drugs in municipal sewage plants using
liquid chromatography-electrospray mass spectrometry." Acta Hydrochimica Et Hydrobiologica
27(6):
430-436.
O'Brien E. and Dietrich, D. R. (2004). "Hindsight rather than foresight: reality versus the EU draft guideline on
pharmaceuticals in the environment." TRENDS in Biotechnology
22 (7): 326-330.
Reddersen, K., T. Heberer, et al. (2002). "Identification and significance of phenazone drugs and their
metabolites in ground- and drinking water." Chemosphere
49(6): 539.
Ruiz, A. J. G., Ruiz, I.G., Lara, P.A., Montesinos, A.C., Crespo, F.M., Cuesta, de la F.S. (1997). "Hypertensive
patients under treatment in Spain: 1990-1993." Rev. Esp. Salud Publica
71(1): 1-12.
Sacher, F., Lange, F.T., Brauch, H-J., and Blankenhoorn, I. (2001). "Pharmaceuticals in groundwaters.
Analytical methods and results of a monitoring program in Baden-Wuerttemberg, Germany." Journal
of Chromatography A
938: 199-210.
Stuer-Lauridsena, F., Birkveda, M, Hansena, L.P., Holten Lutzhoft, H-C, Halling-S¢rensen, B. (2000).
"Environmental risk assessment of human pharmaceuticals in
Denmark after normal therapeutic use." Chemosphere
40: 783-793.
Tauxe-Wuersch, A., De Alencastro, L.F., Grandjean, D. and Tarradellas, J. (2006 (in press)). "Occurrence of
several acidic drugs in sewage treatment plants in Switzerland and risk assessment." Water Research.
Ternes, T., A., Joss, A. (2006). Human pharmaceuticals, hormones and fragrances. The challenge of
micropollutants in urban water management., IWA Publishing.
Ternes, T., Joss, A., Kreuzinger, N., Miksch, K., Lema, J.M., and U. von Gunten, McArdell, Ch.S., Siegrist, H.
(2005). Removal of pharmaceuticals and personal care products: results of Poseidon project. WEFTEC.
Ternes, T. A. (1998). "Occurence of drugs in German sewage treatment plants and rivers." Water Research
32(11): 3245-3260.
Ternes, T. A., Bonerz, M., Herrmann, N., Löffler, D. Keller, E., Lacida, B.B., and Alder, A.C. (2005).
"Determination of pharmaceuticals, iodinated contrast media and musk fragrances in sludge by LC
tandem MS and GC/MS." Journal of Chromatography A
1067: 213-223.
Ternes, T. A. and R. Hirsch (2000). "Occurrence and Behavior of X-ray Contrast Media in Sewage Facilities
and the Aquatic Environment." Environ. Sci. Technol.
34(13): 2741-2748.
Ternes, T. A., Janex-Habibi, M., Knacker, T., Kreuzinger, N. and Siegrist, H. (2004). Detailed report related to
the overall project duration. Poseidon project.
Ternes, T. A., M. Meisenheimer, et al. (2002). "Removal of Pharmaceuticals during Drinking Water Treatment."
Environ. Sci. Technol.
36(17): 3855-3863.
WHO, Ed. (2006). ATC classification index with DDDs. Oslo, Collaborating Centre for Drug Statistics
Methodology WHO.
Wikipedia. "http://en.wikipedia.org/wiki/." Williams, R. T. e. (2005). Human pharmaceuticals. Assessing the impacts on aquatic ecosystems., Society of
Environmental Toxicology and Chemistry (SETAC).
Yoon, Y., Westerhoff, P., Snyder, S.A., Wert, E. C. (2006). "Nanofiltration and ultrafiltration of endocrine
disrupting compounds, pharmaceuticals and personal care products." Journal of Membrane Sciences
270: 88-100.
pathways
occurrence
(aquatic)
environment
Wastewater
The presence of several pharmaceuticals in STP effluents has been confirmed in e.g. Germany, The Netherlands, Switzerland, United Kingdom, France, Greece, Sweden and Italy, Spain the United States, Canada, Brazil, and Australia (Castiglioni 2006), Table 2.1.
Table 2.1: Occurrence of pharmaceutical residues in STP effluents (Castiglioni 2006)
Hospital wastewater
Hospital wastewater is a significant source of pharmaceuticals such as antibiotics, anti-cancer agents and iodinated contrast media. The share of specific antibiotics used in hospitals may vary between few percent up to 90% of total emission (BLAC, 2003). Most hospitals are directly connected to a sewer and no pre-treatment takes place. Also nursing homes are significant point sources of some specific pharmaceuticals.
Surface water
To be able to describe negative effects of pharmaceuticals on the on the aquatic organisms a lot of monitoring studies are being performed to determine the concentrations of different pharmaceutical compounds found in various aquatic compartments. Pharmaceuticals are present in surface water in measurable concentrations. Concentrations depend on a type of pharmaceutical and its active compound and aquatic environment compartment and vary roughly from tens to hundreds of nanograms per liter (surface water) to tens of micrograms per liter in raw influent.
Compounds found most often in surface water are:
- almost all X-ray contrast media, - (few) pain killers - (few) beta-blockers - antiepileptics (carbamazepine and primidon) - antibiotics
Prevalence and concentrations found in the Netherlands are not different than those found in German
(or other European) studies.
In Table 2.2 the maximum concentrations of human pharmaceuticals are given in ng/l (Boxall, 2004).
Table 2.2. Pharmaceuticals detected in surface water monitoring studies; (Boxall 2004), (Daughton, 1999),
(Kolpin DW 2002), (Boxall, 2004a).
Pharmaceutical group
Substance
Max concentration (ng/l)
Chlortetracycline
Sulphadimethoxine
Sulphamethoxazole
Acetylsalicylic acid
Dehydronifedipine
Antihypertensive
Antihyperlipidemic
Acetaminophen (Paracetamol)
Anti-inflammatory
17a-Ethinylestradiol
X-ray contrast media
Ground water
Sacher (2001) analysed 105 ground water wells and in one third of tested ground water samples (39) pharmaceuticals from groups beta-blockers, analgesics, antiepileptics, antirheumatics, antibiotics, iodinated X-ray contrast media could be detected. Carbamazepine was detected in ground water
sample up to 1,1 µg.l-1 (Ternes, 2001) . In a German monitoring program 32 bank filtration samples from 22 surface water were measured; sulfamethoxazole was found at concentrations up to 0.079 µg.l-
1 and diatrizoate up to 1.4 µg.l-1 (BLAC, 2003). The highest concentrations for ground water samples were found for iodinated contrast media iopamidol, up to 2,4 µg.l-1 (Ternes and Hirsch 2000).Of other
dimethylaminophenazone (Reddersen, Heberer et al. 2002), lipid regulators gemfibrozil were detected in ng range (Daughton 2001).
Drinking water
Due to a specific situation with water resources around of city Berlin, some pharmaceutical compounds were detected in drinking water samples: clofibric acid (270 ng.l-1), diclofenac, propylphenazone, ibuprofen. Several compounds were detected in raw drinking water samples in San Diego county, California – clofibric acid, ibuprofen, ibuprofen methyl ester (Loraine and Pettigrove 2006).
Sewage sludge
Some antibiotics were detected in sewage sludge, fluoroquinolones, ciprofloxacin, norfloxacin (Golet, Strehler et al. 2002). Recently other antimicrobials, sulfapyridine, sulfamethoxazole, trimethoprim, azithromycin, clarithromycin and roxithromycin in sewage sludge were detected in activated sludge up to 0.20 mg.kg-1 of dry matter (Gobel, Thomsen et al. 2005). From neutral and acidic drugs only diclofenac was quantified above the limit of quantification (0.2 – 0.45 mg.kg-1) (Ternes 2005).
Predicted environmental concentrations
Predicted (environmental) concentrations is often calculated under the following assumptions:
- all sold pharmaceuticals are used in the same year - the pharmaceuticals are released to the sewer - there is no elimination in man or the sewerage system - the use pattern is evenly distributed temporally and spatially.
This is a worst-case estimate of predicted environmental concentration (PEC) for the surface water where removal in man is not encountered, is calculated as follows:
Consumption(
g / 1000
inh. /
day )
surfacewa e
(
m3 / 1000
inhab d
.
ay ) ⋅
D
Predicted environmental concentration in surface water, taking into account human metabolism:
( 00 −
R)
w = 365 ⋅
P ⋅
V ⋅
D ⋅100
where:
A – the amount of pharmaceutical (active substance) used per year (kg/y)
R – removal (%) in man and sewer
P – numbers of inhabitants
V – volume of wastewater per capita (m3/d)
D – dilution factor in the environment (10 often used) (Stuer-Lauridsena 2000).
Example:
Fibrates/β-blockers: concentration of metoprolol in concentrated wastewater streams: DDD = 200
mg/person/d (Ruiz 1997), excretion of a parent compound E = 5%, Volume undiluted urine: 1.5 L,
ECurine = 6,67mg/L, in black water (BW) collected with vacuum: VBW=7.5 L,
PECBW = 1.3 mg/L.
Calculations of expected concentrations of other PhACs (in this case all beta-blockers) in
concentrated wastewater streams are given in Table 2.3.
Table 2.3: Calculated concentration of selected pharmaceutical compounds in concentrated wastewater
(undiluted urine, black water collected with vacuum toilets)
DDD (Ruiz 1997),
Quantities used in
concentrated black
the Netherlands,
16200 (RECETO) 2.354.000 prescriptions
compound, 90% metabolite)
300 (95% receto)
3 PhACs fate in (re)source oriented sanitation
Source separation based sanitation concept
A number of different wastewater streams are produced in households as a consequence of various human activities (Figure 3.1). In the existing combined sanitation system, all the streams originating from the households are collected with the same piping system and end up to the conventional WWTPs. Wastewater streams can be separated based on their composition and concentrations (STOWA 2005). Black water originating from the toilets is one of the most concentrated streams and consists of faeces, urine and flush water (Otterpohl, Albold et al. 1999; Kujawa-Roeleveld and Zeeman 2006). Grey water is the combination of the sub-streams originating from shower, bath, laundry and kitchen and is relatively diluted (Kujawa-Roeleveld and Zeeman 2006). Black water contains high organic contents as well as the major fraction of the nutrients in domestic wastewater. Besides, most of the pathogens and micro pollutants (pharmaceuticals, hormones etc.) are also present in this stream which has a small volume. Separating urine or black water stream from the others enables to concentrate the risks in a very small volume. This gives an opportunity to have a better control, enabling the recovery of nutrients and energy and limit the negative environmental effects (Kujawa-Roeleveld and Zeeman 2006).
black water kitchen waste
Figure 3.1: Wastewater streams produced in households.
4 Urine as fertilizer: fate of PhACs in soil
Separate collection and processing of human urine is gaining interest. First of all this lies in the fact that human urine contains the largest fraction of nutrients: nitrogen (approximately 80%), phosphorus (approximately 50%) and potassium (approximately 70%)) emitted from households. These could be used, after an appropriate treatment if required, as fertilizers in agriculture (e.g. Wilsenach 2006, Kujawa-Roeleveld and Zeeman 2006). On the other hand, Human urine contain the largest fraction of human pharmaceuticals and their residues (metabolites, conjugates) excreted from human body. Separate collection and treatment of urine may therefore reduce the amounts of residual pharmaceutical compounds that are currently discharged through sewer overflows and by wastewater treatment plants (WWTPs) that are not designed to efficiently eliminate these compounds. Thirdly, disconnection of the urine stream (or part of the stream) from the sewer would enable to save energy at WWTPs (Wilsenach 2006), spent for nitrification of ammonium mainly originating from urine. These are three important reasons why a separate collection and treatment of urine is being considered.
Ambachtshuys Meppel, Anderen: application of urine
separation in the Netherlands
In 2005 the first No Mix (urine separation) toilets were installed in a social working place in Meppel. The results of this demonstration project were positive and received national media attention. To increase the knowledge on urine separation and upscale the practical application of this approach the feasibility of use of urine as a fertilizer for energy crops. It is related to the pilot location in Anderen (Drenthe), which consists of a renovated farm with eight housing units for mentally disabled persons. A total of 20 no-mix toilets have been installed which will be used by about 36 persons. The location is in use since May 2008. The yearly urine production at the location is approximately 6 m3. The field nearby the farm can be used for fertilization with urine and energy crop (most probably coleseed production).
Goal of the research
An important question in this research was whether there is any risk of groundwater contamination with residual pharmaceutical compounds because of the application of urine to a farmland. Most of the pharmaceuticals and hormones are excreted from the human body through urine (approximately 70%) as a parent compound and active or inactive metabolites. This research project assessed the fate of 8 selected pharmaceuticals in soil. There were three types of experiments performed:
1. sorption experiments were conducted to assess the sorption capacity of the soil from the
project location in Anderen for the selected pharmaceuticals. Sorption experiments were performed inbatch mode (determination of sorption isotherms) and continuous model (column experiments).
2. desorption experiments were conducted to assess the strength of the binding; 3. biodegradation experiments were conducted to assess whether and how fast biological
degradation of the selected compounds can take place in the soil.
Research questions
As urine might be a good source of nutrients like N and P there is an increasing interest for applying urine as fertilizer. The main research question for this study was whether reuse of urine may affect the composition of groundwater in relation to the presence of pharmaceutical compounds in urine. The ground water level in Anderen location is at approximately 2 m below the ground level. In the experiments summarized in this chapter sorption processes and biodegradation of selected pharmaceutical compounds were studied in order to predict the transport of the pharmaceuticals in the soil to the groundwater level.
Selection of medicines
Eight pharmaceutical compounds were selected for lab experiments representing a few therapeutic groups, wide range of physical/chemical properties. These were, in alphabetical order: acetyl salicylic acid, bezafibrate, carbamazepine, clofibric acid, diclofenac, fenofibrate, ibuprofen and metoprolol. The criteria used to attained the above selection were: (High) consumption rates in the Netherlands, representation of different therapeutic groups, occurrence in environment, eco-toxicity, physical-chemical properties (hydrophobicity, hydrophilicity, charge, all implicating sorption potential) and expected susceptibility to biological degradation. Not all criteria were fulfilled for each compound. The main idea behind the selection was to have a group of compounds with different properties (e.g. easily biodegradable – persistent, characterized by high sorption affinity and on the other hand very mobile in a given matrix, etc) and representing at least few therapeutic groups (finally there are five represented). The DDD (defined daily dosis), excretion rates, users' fraction of a given compound and frequency of use served as a base to estimate the concentrations of the subsequent pharmaceuticals in urine to apply as a spike value in the performed experiments. Final initial concentration of the selected pharmaceuticals were a compromise between the calculated values and the requirements of the analytical method. This study focused on transport of the selected pharmaceutical compounds under extreme conditions and on the biodegradation at different redox conditions. The obtained information can be used for a first estimation of leaching risks of medicines to ground water.
Soil samples
Soil samples at Anderen were taken. From the soil profile it became clear that several more or less uniform layers could be distinguished. The top soil (0-20 cm) was rather heterogeneous due to grass roots. The organic matter content is expected to determine retention of pharmaceuticals when sprayed on top of the soil. From 20-40 cm a dark layer could be observed with 2.7 % organic matter content (OM). The layer at 40-60 cm showed to be rather yellow and contained 1.4 % OM. Layers of 20-40 cm and 40-60 cm were used in the experiments.
Sorption experiments
Three types of sorption experiments were performed to investigate three different aspects related to sorption of pharmaceuticals to the soil. The first aim was to find out the partition coefficient (Kd) value for the chosen pharmaceuticals in the two soil layers. The second aim was to mimic the behavior of the medicine mixture in urine for a long period as well as for a period of one year with the specific soil of the Anderen location. The third goal was to find out whether pharmaceuticals could become irreversibly bound to soil particles in a similar way as it was demonstrated earlier for hydrophobic organic compounds.
Kd determination
The Kd value is generally accepted and used in retention studies to rank the sorption rates. A standard OECD batch sorption test was chosen to determine Kd values. However the OECD test is only valid for sorption of one specific medicine in water. To create a cost effective experimental set up it was agreed with the "supervision committee" to perform the test with the mixture of the selected pharmaceuticals (pharmaceutical cocktail). However as control one experiment with anti-inflammatory drug diclofenac was performed fully according the OECD protocol. As matrix effects may influence Kd it was decided to perform a sorption experiment using urine instead of water as matrix.
Mimic long term medicine leaching
In a second sorption experiment the aim was to estimate the minimum retention time in the upper soil layer from 20 to 60 cm. For this, column experiments were performed with two 20 cm columns of the soil layers 20-40 cm and 40-60 cm respectively. In these laboratory columns long term effects of a high loading of pharmaceuticals was simulated. The worst case scenario includes a more than 30 yearly doses of excreted pharmaceuticals together with one year rain excess. Also a simulation for both soils was done with an excreted medicine dose in urine of 1 year in combination with 1 year rain excess. To be sure biodegradation is limited to a minimum, the duration of this experiment was only a few hours. As the soil column height of 20 cm is identical to the height of the soil layers, it can be assumed to estimate the residence time in the layer from 20 to 60 cm that the effluent of the 20-40 cm columns can be used as influent for the 40-60 cm column. It was also assumed that the behaviour of the 40-60 cm at low medicine concentration is identical for the low effluent concentration from the 20-40 cm column. This may lead to an overestimation of medicine transport to the groundwater. The results of the OECD sorption experiment and the column experiments were compared. The outcome for the different pharmaceuticals is discussed.
Determination residual medicine concentration in soil
A third sorption experiment was performed to study the reversibility of sorption of pharmaceuticals. In studies of sorption of organic micro pollutants like polycyclic aromatic hydrocarbons (PAH), Polychlorinated biphenyls (PCB), nonylphenol and other compounds it was demonstrated that binding of these compounds to organic matter is (only) partly reversible. Therefore, often a residual fraction of these compounds can be found in soil. In general, the residual fraction increases with time. This process is known as aging. The larger the residual fraction is, the risk for transport of pharmaceuticals to the groundwater will reduce. Here, in this study the use of solid phase extraction was used for the first time for the wide variety of pharmaceuticals investigated here.
Biodegradation experiments
To get a more complete picture of a possible behaviour of the pharmaceutical compounds in soil, additionally, biodegradation experiments were performed. The idea was to obtain a potential biodegradability of subsequent compounds when subjected to a biomass for a longer period (up to 30-60 days). Several batch tests were performed using two types of biological sludge (activated sludge, anaerobic digestion sludge), different redox conditions (aerobic, anoxic, anaerobic) and different temperatures. The sludge mixtures were spiked with 8 pharmaceuticals at the calculated concentrations based on defined daily dose (DDD), excretion rate in urine and urine dilution, and their depletion (if any) was followed by sampling in time intervals. It should be emphasised that the analysis of the subsequent compounds were performed in solid (sludge) and liquid phase so a real depletion (= biodegradation, biotransformation) was determined.
The conditions of the biodegradation batch test were quite different than these in soil, especially regarding the density of biomass. Optimal conditions for biodegradation were applied in which, the susceptibility of the eight specific compounds to biological degradation could be attained. This part of the research could be refined in the future by applying a more closely reflecting conditions in soil (biomass density, prevailing temperature, realistic concentrations of the pharmaceutical compounds, etc.) (see also chapter 6).
Classification of PhAC
The analysis of the results enables to distinguish between two categories of compounds and to assess
the overall risks for ground water contamination (Table 4.1).
Category 1: Compounds posing (very) low risk for ground water contamination represented here by:
− Acetylsalicylic acid could have a high risk of breakthrough because of it is hydrophilic character.
However, this compound degrades rapidly and thus forms no risk for the groundwater.
− Fenofibrate adsorbs very quickly and well. Also this compound seems to be well biodegradable or
transformable (is probably quickly transformed to fenofibric acid in the first instance). As fenofibrate
is hardly used in the Netherlands (although detected in the environment, Versteegh et al., 2007) as a
pharmaceutical, seems not to be relevant. On the other hand all pharmaceuticals compounds
characterized by such a high Kd value (higher than 5) should be strongly bound in soil and form a
threat to ground water reservoirs.
− Metoprolol is used in high quantities in the Netherlands (55 ton/yr). It adsorbs well (no
breakthrough in the column tests and relatively high retardation factor) and is biodegraded relatively
quickly. The accumulation capacity of the soil for this compound is expected to be sufficiently high to
adsorb higher loads of this compounds prior its biodegradation.
− The sorption affinity of diclofenac in the soil was relatively high; there was sufficient sorption
capacity to prevent breakthrough in the soil column runs 2 and 3. The Tenax experiments showed
easy desorption, which indicates a high bioavailability. The aerobic biodegradation tests, showed 95%
degradation within 30 days of the experiment at 10oC (see also chapter 6). The desorption and
biodegradation of the compound is most likely within months.
Although biological conditions in the soil differ significantly from the conditions provided in the, here
performed and described, biodegradation batch tests it is expected that compounds characterized by
a high biodegradation potential will be also degraded in the soil to a significant extent. Compounds
characterized by a high sorption and biodegradation (transformation) potential should also not form a
threat for a ground water resources.
Category 2: Existing or enhanced risk depending on balance between desorption and extent of
degradation in soil:
All compounds listed below (bezafibrate, ibuprofen, clofibric acid and carbamazepine) leached to a
certain extent in the column experiments. The risk for ground water contamination by these types of
compounds will depend on the equilibrium between bioavailability (desorption) and biodegradation
rate in the soil. More attention should be paid in the future research to this group of the compounds:
− Bezafibrate had relatively low retardation factor. It is aerobically biodegradable – full
biodegradation was reached in the tests after 30 days at 10oC. The used quantities of this specific
compound are relatively low in the Netherlands thus the DDD approach was clearly an
overestimation.
− Ibuprofen had low R but it is quickly biodegraded. It is consumed in high quantities but the accumulation capacity of the soil for ibuprofen could be sufficiently high to adsorb higher loads prior to biodegradation. − Clofibric acid does not adsorb very well (low R). As no biodegradation of this compound was found in the tests, it may reach the groundwater in the long run. Clofibric acid is however hardly used in the Netherlands (0.17 ton /y in 2006) and will not likely be used in Anderen. Netherless attention has to be paid to this kind of compounds with low sorption and biodegradation affinity. − Carbamazepine adsorbs much better and has a strong interaction with the soil (not easily desorbed). The soil can accumulate the compound for longer periods. However, as no biodegradation of carbamazepine was found in the tests, it may reach the groundwater in the long run. Carbamazepine is widely used (23 ton /y) and is also used by the residents in Anderen. These types of compounds for a realistic risk for groundwater contamination. For the last two types of compounds it is not excluded that in the long run specific bacteria may develop within the soil matrix that will degrade persistent compounds like carbamazepine or clofibric acid. Also in this point more fundamental, long-term microbiological study should be devoted to this possible phenomenon. Table 4.1. Risk of a pharmaceutical breakthrough after the first 60 cm of soil and the aerobic biodegradability at 10oC: resulting overall risk of groundwater contamination.
1) Defined Daily Dose (DDD) - the assumed average maintenance dose per day for a drug used for its main indication in adults; 2) +++ = rapidly biodegradable (within few hours), ++ = readily biodegradable (less than 1 day), + = moderately biodegradable (1-2 days), +/- = biodegradable only at retention time > 2 days, - = not biodegradable (within test periode). The results of this study emphasize the extremely complex behaviour of the pharmaceutical compounds in soil. At this moment a modeling of the spreading and diffusion of the specific compounds in soil and to the ground water respectively, based on characteristics of the compound (e.g. Kow, Koc) would not be reliable. Based on experimentally determined fate of selected compounds in soil only an indication and estimation of the potential risk can be made. A more specific insight into the soil transport leading to the breakthrough and biodegradation patterns would be desired. The following specific experimental recommendations are listed here shortly:
- Longer duration of column experiments; - Verification of the column tests in terms of pore volumes and extended selection of only
mobile compounds;
Investigation of the effect of soil type and characteristics on behaviour of pharmaceuticals;
Simulating biological conditions in long-term column experiments.
Focus on the behaviour of veterinary compounds being currently applied in excess to the cattle.
Translation of the obtained results to other pharmaceutical compounds
The results obtained with a restricted number of compounds, like in this study, can be theoretically translated to other compounds. Such translation needs more research as one needs to collect a number of data, to mention the most important: partition coefficients (Kd), acid dissociation constant (pKa), chemical structure, consumption per spot, excretion (amounts and forms), etc and perform the statistical analysis. Within the time frame of this project, this goal could not be achieved. For possible continuation of the research on the fate of others, than here chosen, pharmaceutical compounds, one should analyse similarities in chemical structure, physical-chemical properties, toxicity, consumption between these new in here investigated compounds (in combination with already significant knowledge described in the literature). To obtain a generic picture of the behaviour of all registered pharmaceuticals (> 3000 in the EU) in different environmental compartments man could make use of QSAR analyses. Such analysis, being very complex, do not guarantee however that theoretical model prediction will reflect the reality.
5 Fate and behaviour of PhACs residues in plant tissues
and soils
Urine, also called yellow water, is discussed as an alternative fertiliser for agriculture as it contains relatively high concentrations of the macronutrients nitrogen, phosphorus, and potassium
(1-4). But this usage of urine includes the risk of transfer of pharmaceutical residues to agricultural fields
(5,6). Little is known on the fate of pharmaceuticals regarding their accumulation in soils, transfer to groundwater, and incorporation by plants. However, these effects cannot be excluded as fairly high concentrations of pharmaceuticals are expected in urine (
5). The uptake of pharmaceuticals in plants and the effects they exaggerate on plant physiology and development were of major interest when crops are fertilised with urine. Literature states that the uptake of organic compounds by plants is correlated with the molecular weight of the organics (
7). It is assumed that a molecular weight of >1000 makes the absorption by cellular membranes impossible (
8). Moreover, the octanol-water partition coefficient is looked at as a characteristic of the organics strongly affecting their uptake. Briggs et al. (
9,10) have detected that uptake into shoots was most efficient for chemicals with log KOW = 2. In contrast, KOW is reported to be of lower importance according to Trapp (
11) and Topp et al. (
7) who found predictions of the membrane permeability of very lipophobic compounds by their KOW to be very weak (
11). An ion-trap mechanism, i.e. a process with the chemical being neutral outside and dissociated inside the cell (
11,12), is responsible for the incorporation of organics by plants. Additionally, literature shows that pharmaceuticals can affect plant growth when dosed in sufficient concentrations (
13,14) and can be taken up by plants
(15,16). The question is, whether concentrations applied by urine fertilisation to fields are causing any adverse effects towards plants. Another question to be clarified was whether pharmaceuticals are taken up by the investigated plants, and in which concentrations by which plant parts.
Experimental Section
Selection of pharmaceuticals
The number of pharmaceuticals selected for these investigations were limited due to the decision that not only the effects of single substances but also of combinations of several substances should be tested. With respect to conditions such as space requirements etc., it was decided to investigate the effects of three pharmaceuticals as well as various combinations of them at two different concentration levels. The selected pharmaceuticals had to fulfil several requirements. First, they should have been detected in German groundwater. Additionally, they should differ with respect to log KOW, molecular weight and their expected concentrations in "Average German Urine" (AGU (
5); see also Supporting Information). Consequently, three pharmaceuticals were selected: carbamazepine (CZ, CAS-No. 298-46-4), ibuprofen (IBU, CAS-No. 15687-27-1), and 17α-ethinylestradiol (EE2, CAS-No. 57-63-6) (for their characteristics see Supporting Information). All three pharmaceuticals were purchased from Sigma-Aldrich: IBU, minimum 98 % GC; EE2, minimum 96 % HPLC, and CZ (no information on purity given by provider).
Preparation of spiked yellowwater
The urine used as fertiliser in the plant experiments was spiked with the average concentration of the three pharmaceuticals calculated for AGU further on referred to as "natural" (n) concentration level and at a higher level referred to as "artificial" (a): the ten-fold concentration in case of CZ and IBU and a 40-fold concentration for EE2 in order to ensure analytical detection. Twofold pharmaceutical
concentrations compared to AGU were added as pots obtained only half of the liquid planned to add. Nevertheless, the amount of urine guaranteed an optimal supply for the growth period of three months. Beside the single pharmaceuticals, all combinations possible with the three pharmaceuticals were tested at the natural and artificial concentration level. Each pharmaceutical in urine mixture was applied to plants in triplicate. Urine was collected from healthy males in bottles in the two weeks prior to application of urine (week 25) spiked with the pharmaceuticals designated as "UPmix". It was decided to use male urine to keep the hormone level as low as possible. None of the donors were under any medication. Concentrations of macronutrients and TOC as well as average conductivity and pH in the mixed urine were in the usual range (see Supporting Information). Preparation and application of UPmix was carried out in three steps. First, pharmaceutical concentrates were prepared in the laboratory. The required concentration for the three repetitions was achieved by dissolving the solid pharmaceutical in 500 µl methanol. The pharmaceutical-methanol (PM) solution was stored in the fridge at 5°C and transported in a cooling box. In the second step the PM solution was added to 750 ml of urine. The vial containing the PM solution was rinsed with 100 µl distilled water which was added to the UPmix as well. In the case of blanks, the same procedure was executed, but adding 500 µl pure methanol without any pharmaceuticals. Afterwards, the UPmix was stirred with a glass rod, divided into three equal portions of 250 ml and applied to the pots. After each application, the full equipment was thoroughly rinsed with distilled water before the next UPmix was prepared.
Application of pharmaceuticals
The plant experiments were accomplished at the Institute of Plant Nutrition of the University of Bonn from June to September 2007 in the greenhouse of the institute. A number of 64 "Kick-Brauckmann-pots" (height: 26 cm, diameter: 20 cm (
17)) were filled with 9 kg air-dried soil (type: Meckenheimer Krume; luvisol: 16 % clay, 77 % silt, 7 % sand (
18)). The pots contained a bottomless inner pot in a planter with drainage for leachate. The drainage was closed and all the water remained within the pot. Pots were connected to a micro-irrigation system (drop irrigation; Blunat) and adjusted to keep the soil moisture at 80 %. Soil moisture was controlled from time to time by weighing the pots. In week 23, seeds of ryegrass (
Lolium perenne) were seeded in rows into the pots (0.95 g seeds per pot). Germination and development of seedlings was regular. After establishment and initial growth for a period of 2 weeks, the seedlings were treated with different UPmix as direct application on seeds may lead to delay of germination (
19). 250 ml liquid were added to each pot. To keep pots with equal pharmaceutical additions as far away as possible from each other, the experiment was parted into three series: pot No. 1-20, No. 21-40, and No. 41-60. Pots No. 61-64 were additional ones. In each section the same UPmix was only once applied. The experimental setup contained 2 blanks (pots only treated with urine and methanol) in each series as well as 4 pots completely untreated (no pharmaceuticals, no urine). The following morning plants of pots treated with UPmix did not show any differences to the untreated ones. Plants were cut seven times until harvest, the last time being September 9, 2007. Fresh and dry weight of the aerial plant parts cut was determined on each occasion.
Final harvest and sample preparation
The experiment was terminated on September 17, 2007. After the last cutting of the grass, soil samples from top to bottom of each pot were taken using a mechanical soil corer. Approximately 150 g DM were taken from each pot resulting in 5 to 8 holes per pot. The samples were dried at 40°C until a constant weight was achieved, and then ground to small pieces by mechanical pressure exaggerated by a wooden cylinder (specifically used for soil samples). The low drying temperature was used to prevent pharmaceuticals from eventual destruction. After this procedure, soil was sieved through a certified sieve with a pore size of 2 mm. During sieving, grit, gravel and plant parts were removed manually from the sieve. The fine fractions passing the sieve were collected on a foil. After
each sieving, the four endings were lifted; the sample was moved mechanically to the centre and divided into two final samples. Subsequently to processing of each soil sample all tools were cleaned carefully. After soil samples were taken all pots were completely emptied and the root soil mixture was cut into several parts. From this mixture roots were collected manually for further analysis. Roots as well as plant parts close to soil, leftovers from cuttings, were washed with tap water and distilled water in sieves of pore sizes of 0.25 and 0.49 mm, separated from each other and air dried. Aerial plant parts were dried at 40°C for approx. 3 d until constant weight.
Analytical determination of pharmaceuticals
All pharmaceutical analyses were performed in the central laboratory of Hamburg University of Technology.
Soil
Prior to analyses soil samples were dried once more at 50°C and ground. Samples of 10 g soil were
shaken with 50 ml methanol for 2 hours. Subsequently, the suspension was filtered over a paper filter.
The extract was concentrated to 1 ml in a rotary evaporator and stored in 2 ml vials until further
processing.
For silylation, 800 µl of the concentrated extract were pipetted into a compression-proved vial,
evaporated to dryness by a gentle stream of nitrogen, and silylated with 200 µl MSHFBA (N-methyl-
N-trimethylsilylhepta-fluorobutyramide) at 70°C for 1 hour. The residue was dissolved in a small
volume of acetonitrile which was transferred to a graduated flask adjusted to a final volume of 1 ml.
The silylated extracts were analysed by GC/MS. For GC/MS conditions and m/z see Supporting
Information. Each sample was analysed in duplicate. For recovery rates (derived from
pharmaceutical-spiked blank soil analysed as described), LOD, and LOQ see Table 5.1.
Table 5.1:
Recovery rates, limits of detection and quantification of the three investigated pharmaceuticals in soil.
Recovery
detection
(µg kg-1 DM)*
(µg kg-1 DM)*
* Lowest recovery rate considered.
Roots and aerial plant parts
Roots were cut with a cutting machine into fine parts and further ground in a coffee grinder. As only small amounts of root material were available (1.5-4 g DM), the entire material from one plant was used to prepare one sample extract. The ground material was shaken for 2 h in a buffer solution of HCl and KCl (6.5 ml 0.2 M HCl and 25 ml 0.2 M KCl) at pH 2. Solid parts were filtered off by a fluted paper filter and the extract was subdued to solid-phase extraction with abselut Nexus cartridges (500 mg/12 ml, VARIAN). After washing the cartridges with rinsing water of the extraction bottles and subsequently with little H2O of analytical grade, the analytes were eluted with 5 ml methanol and the eluate was concentrated to a volume of 1 or 2 ml (roots) and 2 ml (aerial plant parts). The solutions were analysed by GC/MS as described for soil samples. The identification of each substance was verified by spiking one part of each sample extract with the respective pharmaceutical standards as the matrix of the extracts was very complex. For recovery rates, LOD, and LOQ see Table 5.2. Out of the three substances only carbamazepine could be detected in the extracts, however as its decomposition product iminostilbene (CAS-No. 256-96-2).
Table 5.2:
Recovery rates, limit of detection and quantification of carbamazepine** and ibuprofen in roots and aerial plant parts.
Recovery rate
detection
(µg kg-1 DM)*
(µg kg-1 DM)*
Aerial plant parts
* Lowest recovery rate considered. ** Detected in form of iminostilbene.
EE2 was not detected. The reason being that uptake rates of the investigated plants were much lower than expected from results reported by Schneider (
18). So, even the artificial concentration for EE2 was selected as too low.
Statistical evaluation of experimental results
Results of the pot experiments on rye grass were statistically evaluated with SPSS 15. A one-way ANOVA was accomplished as a one-way descriptive method in cooperation with a Student-Newman-Keuls procedure. α was set to be 0.05 to determine a significant difference between various treatments.
Results & Discussion
Pharmaceutical concentrations determined in soil
IBU and EE2 could not be detected in any soil sample after a three months growth period, although the pots exposed to the "artificial" IBU level contained an initial pharmaceutical concentration of 490 µg IBU kg-1 DM soil after addition of the UPmix. This concentration is 490 times larger than the limit of detection of IBU in soil. Initial EE2 concentration theoretically only amounted to 0.053 µg kg-
1 DM (artificial level) in soil and thus was below its determined limit of quantification in soil. Contrasting to IBU and EE2, CZ was detected in all pots (Figure 5.1) except in one of the two duplicate analyses of the pot exposed to 3n (58 µg CZ l-1 urine). In this case however CZ was detected in traces which were above LOD. CZ is known to be persistent (
20-22), and additionally its concentration was higher than that of EE2.
Figure 5.1:
Measured mean concentrations of carbamazepine in soil samples at day 92. By n "natural", and by a "artificial" concentrations applied are indicated. Error bars show standard deviations of triplicate fertilisation experiment.
Concentrations found in soil samples after 92 d (Figure 5.1) correlate clearly with the applied initial concentrations of 3.2 µg kg-1 DM ("natural concentration", n) and 32 µg kg-1 DM ("artificial concentration", a). On average, 49 % of the applied CZ concentrations were recovered 3 months after application varying between 20 % (in CZ-IBU-n) and 69 % (CZ-IBU-a). Additionally, variations between the duplicate analyses for one pot were low. Variations observed between pots with the same treatment were higher, however, especially for exposures to CZ-a and 3a (standard deviation of 13.8 and 10.7 µg kg-1 DM soil respectively; Figure 5.1). Therefore results presented in Figure 5.1 are only based on two pots. In the case of 3a, concentrations of CZ in one pot were significantly lower with only 4.4 µg kg-1 DM, resulting in the large standard deviation. Via one-way ANOVA the null hypothesis could be clearly rejected: concentrations of CZ applied with the UPmix had clear consequences for concentrations measured in soil. The probability that differences between groups are of coincidental origin was 0.7 %. This result is additionally supported by the Student-Newman-Keuls test partitioning samples into two sub groups confirming that differences were significant. These findings are concurrent with literature on soils irrigated with treated wastewater. Kinney et al. (
20) report detection and even accumulation of CZ in the upper 30 cm layer of soils irrigated with treated wastewater containing approx. 70 ng l-1 of CZ. Concentrations decreased with depth. Additionally, CZ was detected in groundwater wells 12-15 m below fields irrigated with treated wastewater exhibiting 200 ng l-1 of CZ, whereas IBU and EE2 were not detected in groundwater below the respective areas (
21). This is an additional indicator for the higher stability and mobility of CZ compared to IBU and EE2 (
23-25).
Effects of the investigated pharmaceuticals on rye grass
Indirect effects on aerial plant parts
The growth of aerial plant matter was identified for the entire 3 months experimental period. The factor of fresh weight to dry weight was only slightly varying between 6.01 (Control 2) and 7.03 (CZ-IBU-n). Therefore, the results are presented and discussed only in terms of dry weight.
Figure 5.2.:
Overall dry weight of aerial plant parts of rye grass determined during the full growth period. n = natural concentration (white bars), a = artificial concentration (grey bars). "Control 1" indicates plants treated with MeOH and urine, "Control 2" did not receive any application beside water; "3" is the designation for the combination of CZ, IBU, and EE2.
No visual effects were observed except for Control 2 which received only irrigation water without nutrients and thus showed only about 25 % of the biomass production compared to the fertilised grass (Figure 5.2.). Aerial plant parts of the non-fertilised Control 2 were smaller and thinner. The lack of
fertilisation led to a large weight reduction. The overall fresh as well as dry matter of all plants fertilised with yellow water did not show any effect of the added pharmaceuticals (Figure 5.2.). Statistical analysis confirmed these findings. One-way ANOVA, P <0.05, showed a significant difference of the unfertilised control group for fresh and dry matter. Apart from this result, no significant differences were observed and the null hypothesis cannot be rejected which says that pharmaceutical applications at the tested concentrations do not affect the synthesis of plant matter. Moreover, no relationship was detected between the amount of harvested plant matter and the added doses of the particular pharmaceuticals applied disregarding the specific pharmaceuticals contained in the UPmix. As well, pharmaceuticals did not have any effect on the course of the production of aerial plant parts during the growth period (for details see Supporting Information).
Uptake of carbamazepine and ibuprofen into roots and aerial plant parts
Due to the matrix of the plant material extract, detection of CZ and IBU were difficult. Only in those plants exposed to artificial concentrations, CZ could be quantified in the roots. Similarly, CZ could be quantified only in the aerial parts of plants exposed to artificial concentration levels. CZ concentrations in the aerial parts of plants exposed to the natural CZ level (58 µg l-1 in AGU) were similar to limit of quantification (75 µg kg-1 DM). IBU was not determined in aerial plant parts due to the problematic matrix. CZ concentrations in roots were between 131 µg kg-1 DM (one sample of CZ-EE2-a) and 426 µg kg-
1 DM (one sample of CZ-a) with a mean concentration of 225 µg kg-1 DM while a tenfold concentration was reached in aerial plant parts (mean concentration: 4950 µg kg-1 DM; span: 2600 µg kg-1 DM (CZ-EE2-a) to 6950 µg kg-1 DM (CZ-a)). Instead of measuring CZ concentrations in roots, in two pots (exposing CZ-IBU-a and 3a) the plant crowns were extracted and analysed as amount of root material was insufficient. As concentrations in the two samples of stocks were within the range of the two analyses of roots of the same series (CZ-IBU-a: 243 µg kg-1 DM in the crown, 202 and 321 µg kg-1 DM in the roots; 3a: 131 µg kg-1 DM, 175 and 184 µg kg-
1 DM), for these two pots the concentration in crown was assumed to be close to mean concentrations in roots (Figure 5.3).
Figure 5.3:
Mean concentrations of carbamazepine measured in roots and aerial plant parts of rye grass after 92 days growth period. Error bars show standard deviations for the three equally exposed pots.
Figure 5.4:
Comparison between incorporation of carbamazepine (applied at artificial level) into aerial plant parts and roots of rye grass.
Statistically relevant correlations between the uptake of CZ into roots and into aerial plant parts could not be determined (Figure 5.4). This might be a consequence of considerable coefficients of variation in the difficult matrix of plant extracts. Only when comparing the mean concentrations of the three pots of one UPmix, a weak correlation was observed. In general, CZ concentrations in aerial plant parts were one order of magnitude larger then in roots. An average of 0.21 % (between 0.12 and 0.40 %) of the total amount of CZ applied to each pot (under artificial conditions 290 µg per pot) was found in the roots of rye grass, but 30 % (between 15 and 42 %) in the aerial plant parts. It can be assumed that IBU (neither detected in soil nor in plant roots) is not incorporated by plants due to its fast biodegradation in soil while CZ which is present in soil for longer periods due to its recalcitrance. Thus, CZ remains available for plants for a much longer period and is thus transferred to the plants, especially to the aerial plant parts in the case of rye grass. It has to be pointed out that the uptake rates of the aerial plant parts of natural and artificial concentrations were non-linear. CZ concentrations in the aerial plant parts exposed to the natural CZ level were in the range of the limit of quantification (75 µg kg-1 DM) and those exposed to artificial CZ levels showed an average concentration of 4950 µg kg-1 DM. While CZ concentrations measured in soil and roots reflected the order of one magnitude (10 fold) which was chosen for the two application regimes ("natural" and "artificial"). This research did not consider potential degradation of pharmaceuticals in the rye grass during the growth period. Moreover, this study does not allow for an evaluation of potential toxic effects of pharmaceuticals ingested by humans with crops.
References
(1) Larsen, T.; Gujer, W.: Separate management of anthropogenic nutrient solutions (human urine).
Water
Sci. Technol. 1996,
34 (3-4), 87-94.
(2) Muskolus, A. Anthropogenic plant nutrients as fertiliser. PhD Thesis, Institut für Pflanzenbauwissenschaften, Humboldt-Universität zu Berlin, Berlin, Germany, 2008.
(3) Otterpohl, R.: Options for alternative types of sewerage and treatment systems directed to improvement
of the overall performance.
Water Sci. Technol. 2002,
45 (3), 149-158.
(4) Vinnerås, B.; Jönsson, H.: The performance and potential of faecal separation and urine diversion to
recycle plant nutrients in household wastewater.
Bioresour. Technol. 2002,
84 (3), 275-282.
(5) Winker, M.; Tettenborn, F.; Faika, D.; Gulyas, H.; Otterpohl, R.: Comparison of analytical and
theoretical pharmaceutical concentrations in human urine in Germany.
Water Res. 2008,
42 (14), 3633-3640.
(6) Lienert, J.; Güdel, K.; Escher, B.: Screening method for ecotoxicological hazard assessment of 42
pharmaceuticals considering human metabolism and excretory routes.
Environ. Sci. Technol. 2007,
41 (12),
4471-4478.
(7) Topp, E.; Scheunert, I.; Attar, A.; Korte, F.: Factors affecting the uptake of 14C-labeled organic chemicals
by plants from soil.
Ecotoxicol. Environ. Saf. 1986,
11 (2), 219-228.
(8) Sanderson, H.; Brain, R.; Johnson, D.; Wilson, C.; Solomon, K.: Toxicity classification and evaluation of
four pharmaceuticals classes: antibiotics, antineoplastics, cardiovascular, and sex hormones.
Toxicology 2004,
203 (1-3), 27-40.
(9) Briggs, G.; Rigitano, R.; Bromilow, R.: Relationship between lipophilicity and root uptake and
translocation of non-ionised chemicals by barley.
Pestic. Sci. 1982,
13 (5), 495-504.
(10) Briggs, G.; Bromilow, R.; Evans, A.; Williams, M.: Relationship between lipophilicity and the
distribution of non-ionised chemicals in barley shoots following uptake by the roots.
Pestic. Sci. 1983,
14, 429-
500.
(11) Trapp, S.: Modelling uptake into roots and subsequent translocation of neutral and ionisable organic
compounds.
Pest Manag. Sci. 2000,
56 (9), 767-778.
(12) Briggs, G.; Rigitano, R.; Bromilow, R.: Physico-chemical factors affecting uptake by roots and
translocation to shoots of weak acids in barley.
Pestic. Sci. 1987,
19 (2), 101-112.
(13) Jjemba, P.: The effect of chloroquine, quinacrine, and metronidazole on both soybean plants and soil
microbiota.
Chemosphere 2002,
46 (7), 1019-1025.
(14) Kumar, K.; Gupta, S.; Chander, Y.; Singh, A.: Antibiotic use in agriculture and its impact on the
terrestrial environment.
Adv. Agron. 2005,
87, 1-54.
(15) Dolliver, H.; Kumar, K.; Gupta, S.: Sulfamethazine uptake by plants from manure-amended soil.
J.
Environ. Qual. 2007,
36 (4), 1224-1230.
(16) Boxall, A.; Johnson, A.; Smith, E.; Sinclair, C.; Stutt, E.; Levy, L.: Uptake of veterinary medicines from
soils into plants.
J. Agric. Food Chem. 2006,
54 (6), 2288-2297.
(17) Kick, H.; Große-Brauckmann, E.: Über die Konstruktion eines Vegetationsgefäßes aus Kunststoff.
J.
Plant Nutr. Soil Sci. 1961,
95 (1), 52-55.
(18) Schneider, R. Pharmaka im Urin: Abbau und Versickerung vs. Pflanzenaufnahme. In Proceedings of
Nährstofftrennung und -verwertung in der Abwassertechnik am Beispiel der "Lambertsmühle"; Bonn, Germany, 2005.
(19) Simons, J.; Clemens, J. Urin-/Pharmaka-Keimtest. In Proceedings of
Nährstofftrennung und -verwertung in der Abwassertechnik am Beispiel der "Lambertsmühle"; Bonn, Germany, 2005.
(20) Kinney, C.; Furlong, E.; Werner, S.; Cahill, J.: Presence and distribution of wastewater-derived
pharmaceuticals in soil irrigated with reclaimed water.
Environ. Toxicol. Chem. 2006,
25 (2), 317-326.
(21) Ternes, T.; Bonerz, M.; Herrmann, N.; Teiser, B.; Andersen, H.: Irrigation of treated wastewater in
Braunschweig, Germany: An option to remove pharmaceuticals and musk fragrances.
Chemosphere 2007,
66
(5), 894-904.
(22) Winker, M.; Faika, D.; Gulyas, H.; Otterpohl, R.: A comparison of human pharmaceutical concentrations
in raw municipal wastewater with yellowwater.
Sci. Total Environ. 2008,
399 (1-3), 96-104.
(23) Quintana, J.; Weiss, S.; Reemtsma, T.: Pathways and metabolites of microbial degradation of selected
acidic pharmaceutical and their occurrence in municipal wastewater treated by a membrane bioreactor.
Water
Res. 2005,
39 (12), 2654-2664.
(24) Ying, G.; Kookana, R.: Sorption and degradation of estrogen-like-endocrine disrupting chemicals in soil.
Environ. Toxicol. Chem. 2005,
24 (10), 2640-2645.
(25) Richter, O.; Kullmer, C.; Kreuzig, R.: Metabolic fate modeling of selected human pharmaceuticals in
soils.
Clean 2007,
35 (5), 495-503.
6 Biodegradability of PhACs from concentrated
wastewater streams
A biodegradability of eight selected pharmaceutically active compounds (PhAC) was assessed under various environmental conditions (red-ox, temperature, character of biological sludge). Selected PhACs were characterized by different physical-chemical-biological properties in order to be able to extend the results of this research to the broader group of environmentally relevant micro-pollutants. The selected compounds were: acetylsalicylic acid (ASA), bezafibrate (BZF), carbamazepine (CBZ), clofibric acid (CFA), diclofenac (DCF), fenofibrate (FNF) and metoprolol (MTP). Many PhAC can be biodegraded under aerobic conditions; the extent of biodegradation depends in many cases on the exposure time of a biomass to a given compound. Aerobic biodegradation is faster than anoxic degradation; elevating operational temperatures speed up the biodegradation processes, as expected. Under anaerobic conditions and relatively long retention times (HRT=30 d) some PhAC can be degraded (ASA, IBU, FNF) but at much lower rate than under aerobic or anoxic conditions. The anaerobic digestion process, is however not expected, to contribute significantly to elimination of majority of PhACs. Optimisation of process conditions for a (semi)persistent group of PhAC (CBZ, CLF, DCF) will only result in their partial (if any) biodegradation. For new sanitation concepts for source separated wastewater, where anaerobic digestion is applied as an efficient pre-treatment for a bulk of organic matter, and aerobic as a main treatment, addition of a physical or chemical polishing unit to eliminate persistent compounds (when demanded) will be unavoidable.
Human pharmaceutically active compounds (PhAC) are consumed in large quantities world wide; the consumption is in the range of tons per year per one PhAC per country, depending on its size. The expectations are that these amounts will only keep increasing because of a improving health care system and longer life expectations of the citizens. The PhACs comprise a large group of chemical compounds (more than 3000 registered in EU) having different therapeutic mode of action in a human body, different chemical-physical properties and susceptibility to degradation in biological systems. After administered by humans they are metabolized and excreted partially as a parent compound (usually a small fraction) and a number of inactive or active metabolites. Generally approximately 70% of PhAC forms are excreted with urine while 30% with feaces (Lienert 2007). Once excreted, they enter a sewer and a sewage treatment plant (STP). In current STPs employing activated sludge process as a main treatment, PhAC are removed only partially (Ternes 2006). Discharged to surface water they form a threat to aquatic life and re-enter water cycle. Advanced post-treatment processes (e.g. oxidation techniques, tight membrane filtration, activated carbon sorption) are reported to be promising techniques to lower further the concentrations of emitted PhACs. They are however very expensive since large wastewater volumes need to be treated. A new sanitation approach, applying separation of wastewater streams containing all (black water) or majority (urine) of PhACs and their target treatment, may enable to minimise the emission of human PhACs to the environment since it concerns a significantly smaller stream to be handled (sewage 200 L/p/day; urine 1.5 L/p/d when collected undiluted, black water 7.5 L/p/d when vacuum toilet is applied). Little is known on fate of PhAC when biologically treating very concentrated wastewater streams. This study focus on fate of selected PhACs (Table 6.1) in biological wastewater treatment system. For a selection of test compounds a number of criteria were taken into account: consumption, occurrence in aquatic environment, differences in physical-chemical properties (e.g. polarity, hydrophobicity) and suspected biological degradability (persistent, biodegradable), potential eco-toxicological effects and availability of analytical methods, to mention the most important. An attempt is taken hereby to represent with this selection of eight compounds a broader group of PhACs.
Table 6.1 Selected PhACs for biodegradation tests
Acetylsalicilic acid, (
ASA) painkiller, anti-inflammatory,
Metoprolol, (MTP) beta blocker C15H25NO3 logKow=1.9,
antithrombotic C9H8O4
logKow=1.426, pKa=3.5,
Diclofenac,(
DCF)
anti-inflamatory
C14H11ClN2O2
Clofibric acid, (CFB) metabolite of cholesterol lowering
logKow=0.7-4.5, pKa=4.15, kbiol<0.1 L/gSS/d
agent, C10H11ClO3 logKow=2.57, pKa=3.0, kbiol 0.3-0.8
Ibuprofen,
C16H13ClN2O
Bezafibrate,
logKow=3.481, pKa=4.5-5.2, kbiol<21-35 L/gSS/d
C19H20ClNO4 logKow=4.25, pKa=3.6, kbiol 2.1-3.0 L/gSS/d
Carbamazepine ,
(CBZ) antiepileptic C15H12N2O
Fenofibrate,
(FNF) cholesterol lowering agent, C20H21ClO4
logKow=2.69, pKa=<1; 13.9,
Fate of some of these compounds (e.g. CBZ, DCF, IBU) in wastewater treatment systems have been already described in literature to some extent, but very little in relation to source separation based wastewater treatment concepts. The major difference between two concepts is a significantly higher concentration of PhACs (lower mg/L range against µg/L as measured in conventional STP) as well as macro-pollutants (bulk organics, nutrients) and different process configurations (anaerobic pre-treatment followed by aerobic main treatment and tertiary physical or chemical polishing, (Kujawa-Roeleveld and Zeeman 2006)) . Biodegradation potential was determined in batch tests under various red-ox conditions (aerobic, anoxic and anaerobic), at various temperatures using different types of sludge spiked with a mixture of PhACs.
Materials and methods
Chemicals, solutions
The PhACs were obtained from Sigma-Aldrich (Steinheim, Germany): ASA ≥99.0% (CAS-nr: 50-78-2), BZF ≥98% (CAS-nr: 41859-67-0), CBZ (CAS-nr: 298-46-4), CFB 97% (CAS-nr: 882-09-7), DCF (diclofenac sodium salt) (CAS-nr: 15307-79-6), FNF ≥99% (CAS-nr 49562-28-9), IBU ≥98% (GC) (CAS-nr: 15687-27-1) and MTP as Metoprolol (+)-tartrate salt ≥98% (titration) (CAS-nr: 56392-17-7). Sodium nitrate (for anoxic tests) and chloroform (for sample preservation) (pro analysi) were obtained from Merck (Darmstadt, Germany). Methanol (for stock solution of PhACs) (HPLC-grade) was obtained from LAB SCAN (Dublin, Ireland). A stock solution of PhACs was prepared in 50 ml of methanol. A 0.5 ml of this concentrated stock solution was spiked to the batches for obtaining the desired concentration of each of 8 PhAC. The required initial concentrations of the eight PhACs in the batch experiments were approximately 2; 0.3; 0.8; 0.9; 0.5; 0.8; 2 and 2 mg/L for ASA, DLC, IBU, CBZ, MTP, CFA, BZF and FNF respectively. Such relatively high concentrations are likely to occur in source separated sanitation concept were urine or concentrated black water are separately collected and treated. The calculation of the concentration is based on the Defined Daily Dose (DDD) (WHO 2006) and an excretion rate of a parent compound by humans being treated (KNMP 2006).
Sludge origin and characteristics
Activated sludge for aerobic and anoxic batch tests was obtained from municipal STP in Bennekom (the Netherlands) involving a biological moderately loaded (0.4 kg COD/m3/d) activated sludge treatment with nutrient removal. Activated sludge samples for the aerobic and anoxic biodegradation batch tests were collected at the end (effluent to sedimentation tank) of the aeration circuit. The sludge for the anaerobic biodegradation test originated from a demonstration-scale UASB septic tank treating concentrated black water (T=35oC) in Sneek (the Netherlands) (Zeeman 2007).
Aerobic biodegradation experiment
In the aerobic biodegradation experiment a mixture of PhACs was spiked to a 1 L of activated sludge. The sludge was incubated and aerated at a constant temperature. Experiments were performed in duplicates at two temperatures of 20oC and at 10oC (AER-20, AER-10). The tests were carried out for
30 days. In the first two days an intensive sampling took place, referring to the typical HRT at a STP. After, two samples were taken, at 15 and 30 days, to see any adaptation of sludge or its changed behaviour when operating under stress conditions (no organic co-substrate added). Prior to start of the experiment the activated sludge was aerated for a few hours to bring it to endogenous conditions and the required temperature. The batches were aerated to keep a sufficient high oxygen level and ensure good mixing of the sludge and added substances. Water losses due to evaporation were compensated by addition of (Millipore) water.
Anoxic tests
In the anoxic biodegradation experiment a mixture of PhACs was spiked to the 1 L volume activated sludge subjected to anoxic conditions. The sludge was incubated at a constant temperature under oxygen free and nitrate rich conditions. Analogously to the aerobic tests the anoxic experiments were performed at 20oC and 10oC (ANOX-20 and ANOX-10), all in duplicates. The activated sludge was sampled from the STP a day prior starting the experiment. The oxygen in a liquid phase was depleted by storing the sludge without aeration over night. To obtain and keep oxygen free conditions, the gas phase in the batches was flushed with nitrogen gas before the start of the experiment and after sampling. A nitrate solution was prepared to obtain an initial concentration of nitrate in the batches of 20-40 mg N-NO3/L. Nitrate concentration in the liquid was followed in time. When denitrification was almost completed, an appropriate volume of NaNO3 solution was added again to obtain a required N-NO3 level in the remained volume. To assure a good mixing in the batches a shaker was used (85 rpm).
Anaerobic tests
In the anaerobic biodegradation experiment (AN-30) a mixture of PhACs was spiked to the 0.4 L volume of anaerobic (mesophilic) sludge. The batches (duplicate) were incubated under anaerobic conditions at constant temperature of 30 oC and shaken continuously. To ensure strictly anaerobic conditions, the bottles' content was flushed with nitrogen (10 s) prior to the start of the experiment. The bottles were capped. After each sampling, the gas phase of the bottles was flushed again with nitrogen. During all batch experiments mixed liquor samples were taken; liquid and solid fraction were separated from each other to determine the concentration of the PhACs in both phases. From the declined total amount of considered compounds the biodegraded fraction was determined as well as process kinetics. Control tests containing a mixture of 8 selected PhACs in Millipore water to trace any possible interactions between PhACs themselves or (other) abiotic transformation were performed in parallel. The total solids and volatile solids (TS, VS) of the sludge were determined at the beginning of the test, after 2 days (except for AN-30) and after 30 days. Before the addition of PhACs, a sample was taken to determine the background concentration of PhACs in the activated sludge mixture. During the experiment pH, T and DO or ORP measurements were regularly performed. Bottles were covered with aluminium foil to prevent photolytic degradation.
Sampling
A 30 mL mixed liquor samples were taken with a syringe (AER) or sampling needle (ANOX, ANAE). By flushing with nitrogen, 30 ml of mixture was taken out of the bottle in the anoxic test. To the 30 ml of sludge mixture sample a 4-5 drops of chloroform were added. Subsequently, the samples were centrifuged for 10 min at 4000 rpm / 2800 rpf using the centrifuge FirlabO SW12R (with rotor type FACENSW12001) and IEC thermo CL31R (with rotor type AC 100.10A). After centrifuging, the solid (4 mL) and liquid phase (20mL) were separated. From the controls, samples of 20 ml liquid were taken. All samples were stored under -75 oC in disposable centrifuge tubes (PP-Test tubes 50ml, CELLSTAR). The series of samples were sent for analysis to RIVM (ARO-CRL) during and after finishing the experiment.
Analysis of PhACs
All chemicals and reagents used in analytical method were of high purity quality. Liquid chromatography (LC): Waters Chromatography Acquity UPLC separation module; column: Acquity UPLC BEH C18 1.7 µm (100 * 2.1 mm ID) were applied. Column temperature was 65°C. The LC mobile phase consisted of a mixture of 0.1 percent acetic acid (solution A ) and acetonitrile (100%). The gradient used was linear, started at 10% B and progressed to 30% B in 3 minutes after which it was increased to 100% B in 6 minutes . After 9 min the mobile phase was kept for 2 min at 100% B, then the percentage B was decreased to 10 percent in 0.01 minute. The mobile phase flow was set at 0.4 mL/min. The injection volume was 20 µL. Mass-spectrometer (MS) analysis was carried out on a Waters-Micromass Ultima Platinum. Depending on a compound the measurement was carried out in positive or negative electrospray ionisation (ESI) mode. In case of co-eluting compounds the ionisation alternates between positive and negative. The following settings were used in positive ESI mode (MTP, CBZ, BZF, FNF): capillary voltage was 3.5 kV. Cone voltage was 35 V. RF lens 1: 15, aperture: 0.1 and RF lens 2: 0.3. Source temperature was 120°C and desolvation temperature: 325°C. The cone gas flow was 116 L/h and the desolvation gas flow was 701 L/h. LM1/HM1 resolution was 14, with ion energy: 0.8. LM2/HM2 resolution was 14.5, with ion energy: 1.0. For the collision cell the entrance was 7, with a CE gain of 2 and exit 0. Collision cell pressure 3.06e-03. In negative mode (ASA, CFA, DCF, IBU): capillary voltage was 1.2 kV. Cone voltage was 35 V. RF lens 1: 5, aperture: 0.5 and RF lens 2: 1.0. Source temperature was 120°C and desolvation temperature: 325°C. The cone gas flow was 116 L/h and the desolvation gas flow was 701 L/h. LM1/HM1 resolution was 14, with ion energy: 0. LM2/HM2 resolution was 14.5, with ion energy: 1.0. Collision cell pressure 3.06e-03. For the collision cell the entrance was 10, with a CE gain of 1 and exit 0. Sample clean-up of the liquids was straight forward. The samples were 10 times diluted in LC-eluents A, after which they were vortexed for 10 seconds. Samples with lower concentrations were acidified with 2µl 50% acetic acid. The samples were direct injected. Sample clean-up of the sludge was performed by a liquid liquid extraction. A portion of the sample (circa 0.5 gram) was weighted and 5 mL of acetonitrile was added. The samples were sonified by an ultrasonic finger for 20 s followed by rotating head over head for 10 min. After which the sample was centrifuged. The supernatant was transferred to a clean tube and evaporated under nitrogen at 55°C. The dried sample was reconstituted in 1 mL of eluents, followed by 10 min ultrasonification. To correct for losses due to sample storage and to correct for signal suppression due to matrix compounds were the calibration curves prepared in reprehensive blank materials for each corresponding experiment.
Results and conclusions
Background concentrations of PhACs
The concentration of PhACs in the activated sludge mixture from the end of the activated sludge tank (=effluent) and sludge mixture from the UASB septic tank are shown in Figure 6.1. Except for ibuprofen all PhACs were detected in the activated sludge in the low µg/l range, confirming literature findings. DCF was present in relative high concentrations. The presence of majority of the selected compounds in the effluent of a conventional STP confirms their poor or incomplete removal. In the anaerobic sludge from the demonstration scale UASB septic tank the PhAC concentrations were much higher (up to 100 µg/L). This higher concentrations confirmed expectations; the digester treats at least 20x more concentrated wastewater than sewage and the expected removal efficiency under anaerobic conditions is low for majority of PhACs. All measured PhACs prevailed in the liquid phase.
MTP ASA CBZ CFA BZF DCF IBU FNF
Figure 6.1: Background concentrations of selected PhACs in activated sludge sample from an
effluent of the aeration tank of the municipal STP (left) and mesophilic anaerobic sludge from UASB
septic tank treating concentrated black water (right).
6.3.2 Aerobic biodegradability
In AER-20 a fast decrease of ASA was observed; within 1 hour the concentration in the water phase was under the detection limit (d.l. = 0.005 µg/l). In AER-10 the concentration was lower than d.l. after 3 h (Figure 6.1.2). A fast decrease of FNF concentration was observed; at 20oC the total concentration decreased to values under the detection limit. However, this was also observed in the controls. At 10oC a disappearance of FNF in the biodegradation test and in the control was observed as well. For this reason it is uncertain, which part of the FNF reduction was due to biological activity and which part was caused by abiotic reactions (sorption to materials used during the test, analysis and sampling). IBU was exponentially eliminated to concentrations under the detection limit within 2 days of the test. The disappearance rate of IBU was slower at 10oC as compared to 20oC. MTP was eliminated exponentially but at slower rate as compared to IBU. At 20oC, this PhAC was eliminated to concentrations under the detection limit within 2 days, while at 10oC it was then still present. Prolongation of the test to 30 days, enabled to remove MTP below the detection limit. BZF was removed less efficiently. After 2 days of aerobic test at 20oC a 40% of BZF was removed. In the AER-10 the reduction of BZF was not significant. The difference between the tests was quite large. After 30 days the BZF in all three aerobic tests was under the detection limit. DCF was not eliminated in the first 2 days. In both tests, no significant decrease in DCF was measured within 48 hours. Remarkably after 30 days, DCF was transformed significantly, up to about 90% in both tests. This shows that DCF could be potentially eliminated in biological systems. No decrease in concentrations of CBZ and CFA was observed after 2 days nor after 30 days.
Anoxic biodegradability
The ANOX-10 was performed over a time period of 2 days while the ANOX-20 over 30 days. ASA was completely transformed in both tests, however this transformation was slower than in the aerobic tests. The transformation of ASA was faster at 20oC than at 10oC. At anoxic conditions FNF was eliminated relatively fast, like in the aerobic tests. Differences in transformation rates between different temperatures were not significant. The stability of the concentration of FNF in the controls in both anoxic tests at the first 2 days of the experiments was observed, contrary to aerobic tests. The stability of the FNF concentration in the controls in the first 2 days, show that the conversions of FNF had a biological character. IBU was removed, but at slower rate than under aerobic conditions. Differences between the anoxic degradation rate in relation to temperature were observed, with a higher rate at a temperature of 20°C, as expected. In contrary to aerobic tests, MTP was only eliminated to a small extent within 2 days. At 20°C, MTP continued to decrease in concentration after 48 hours to reach the d.l. after 30 d. In the ANOX-10 no significant removal of MTP was observed. BZF did not decrease in concentration in the ANOX-10 test, while in ANOX-20 a significant elimination of BZF was observed; after 1 month a concentration of BZF reached the d.l. Compared to the aerobic tests, a degradation rate in the ANOX-20 test was higher than this in the aerobic tests. At a temperature of 10°C, DCF concentration remained constant in time. In the ANOX-20, DCF seemed to
be reduced to a certain extent after 48 hours but the samples taken after 30 days showed that the concentration of DCF was still in the same range. Both anoxic tests showed no decrease in concentration of CBZ and CFA. Comparing both, aerobic and anoxic tests, the PhACs, which showed to be (partly) degradable under aerobic conditions showed generally a lower degradation rate under anoxic conditions, with the exception of BZF in anoxic test at 20°C. Next to this, ASA, IBU, MTP and BZF showed a different degradation rate between the tests at 20o and 10oC. For MTP and BZF this temperature difference resulted in a small removal at 20oC and no significant removal at 10oC within 48 hours. After 30 days MTP, BZF, IBU, ASA and FNF decreased in concentration to under or close to the detection limit. In these anoxic tests the control concentrations stayed constant in the time interval in which PhAC concentrations decreased. Elimination of PhACs in anoxic tests was therefore most likely a result of biotransformation processes.
Anaerobic tests
ASA, FNF and IBU were exponentially reduced in anaerobic tests, however with a much slower rate than under aerobic and anoxic conditions. Nevertheless, after 30 days, which could be a common HRT for anaerobic treatment of concentrated black water (STOWA 2005), the concentration of all three PhACs decreased by more than 90%. However, in the controls a sharp decrease in their concentrations was measured as well. Because of that the disappearance of a compound in the biodegradation tests, can not be fully assigned to biotransformation processes. Removal efficiency of IBU in anaerobic digesters was confirmed in literature and amounted to 26-56% (Carballa, Omil et al. 2007). However in their research also a removal of DCF was reported, of 59-79%, not found in this study. For CBZ no removal was observed, as in this study. For MTP, BZF, DCF, CBZ and CFA, no significant decrease in concentration was measured in the anaerobic test. Selected results of the batch tests, AER-20, ANOX-20 and AN-30 are presented in Figure 6.2.
Sorption
The fraction of the selected PhAC sorbed to the sludge was of a minor importance. For most of PhACs the concentration in the solid phase was <10%. For the non-acidic pharmaceuticals, MTP and CBZ, sorption was somewhat higher. The very hydrophobic but fast eliminated FNF was highly sorbed (up to 80% in anaerobic system).
Assessment of biodegradation kinetics
The results, in which exponential decrease of PhACs in the course of a given test was obtained, were used to calculate, the first order degradation rate constant (k, 1/d) and the pseudo first order reaction rate constant (kbiol, L/gTS/d) (Joss, 2006) (Table 6.2). The degradation rate constant of FNF and ASA was also determined, but not in all tests it is demonstrated that their removal is due to biological processes; certain values therefore may represent the disappearance rate as indicated in the table. The degradation kinetics in the aerobic, anoxic and anaerobic tests differed clearly. Comparing the aerobic and the anaerobic tests, the degradation rates were 20 to 200 higher for ASA, FNF and IBU. A difference between aerobic and anoxic tests was of a factor 2 to 4 for IBU and BZF. For other compounds, no exponential curve could be fitted, so no comparison could be made between the different rates. The degradation kinetics under aerobic conditions are reported in literature for some of the selected PhAC (Joss 2006). In this research the kbiol – values were lower than these found in literature. The differences between these experiments and the experiments reported in literature is the concentration of PhACs. Also de Mes (2007) investigating biodegradability of elevated concentration of human estrogens found lower kbiol than reported in literature when working with lower concentrations. The activated sludge used in this research was not adapted to such high concentrations of PhACs. A
continuous, pilot-plant experiment, where biomass would be exposed to higher PhAC concentrations could reveal possible enhancement of biodegradation due to adaptation of biomass. In contrast, the anaerobic sludge was already imposed for a longer period to elevated concentration of PhACs, but the anaerobic conditions do not favour the biodegradation of majority of PhAC compounds.
Figure 6.2: Course of PhACs in selected biodegradation tests; ♦ AER-20; ▲ANOX-20; ○ AN-30.
The biodegradation tests showed a potential for some of the selected PhACs to be bio-transformed, in
some cases significantly. The degradation rates differed per compound and environmental conditions
applied. The continuation of the aerobic and anoxic batch tests for 30 days provided more
information. The, at the first sight, persistent DCF was eliminated for 90% in the aerobic tests after 1
month. The PhACs partially eliminated during 2 days, like MTP and BZF, were completely removed
to levels under or close to the d.l. of 0.005 ug/l when the aerobic and anoxic test was prolonged to 30
days and biomass was subjected to stress conditions (no external substrate supplied).
It should be kept in mind that only the removal of the original PhAC was analyzed. Whether a given
compound is mineralized or degraded and if the subsequent produced degradation product is
biodegradable is at this moment unclear. Regarding IBU and ASA the produced metabolites are not
likely to be persistent to biodegradation. According to results of (Quintana 2005) the metabolites of
BZF are also degradable. The possible produced metabolite fenofibric acid of FNF can be transformed
most likely too although not much is known about other metabolites produced. The biodegradability
of metabolites of MTP and DCF are unknown.
Some compounds remain very persistent independent on the environmental conditions applied during
the biological treatment (CBZ, CLF). If the objective of wastewater treatment will become once to
eliminate all PhACs, the addition of advanced chemical or physical process units seems to be
unavoidable to remove biologically persistent compounds.
Table 6.2 The degradation rate constant k, its 95% confidence interval, the range of specific degradation rate
constant kbiol based on the TS concentration and related the 95% confidence interval and the R2 of the regression
model for tested PhACs under various environmental conditions (assessed where possible)
PhAC
* the specific
disappearance rate constant, since other abiotic processes could have played a role, n.a. not assessed
Conclusions
Biodegradation experiments were performed for eight selected PhACs under various red-ox conditions. The selection had a goal to include compounds of various physical-chemical-biological characteristics implying their different behaviour during biological wastewater treatment processes.
The applied initial concentrations of PhACs were significantly higher than these at the conventional treatment plant (low mg/L range against low µg/L range). The reason was the applicability of the results to treatment concepts for concentrated black water or urine.
A summary of biotransformation behaviour for all selected pharmaceuticals together with the influence of different environmental conditions is given in Table 6.3.
Table 6.3: Comparison of biotransformation rate of the selected PhACs at different environmental conditions.
Biotransformability: +++ high, ++ good, + moderate, +/- only at HRTs > 2 days, - not biotransformed
As certain PhACs remain persistent to biological degradation extension of biological treatment (anaerobic-anoxic-aerobic) of source separated wastewater with physical or chemical process units will be unavoidable when the intention is to stop the emission of PhAC to the environment.
Acknowledment
The National Institute for Public Health and the Environment (RIVM), Laboratory for Food and
Residue Analysis is greatly acknowledged for development and performance of the analytical part of
PhACs.
Literature
Carballa, M., F. Omil, et al. (2007). "Fate of pharmaceutical and personal care products (PPCPs) during
anaerobic digestion of sewage sludge." Water Research
41(10): 2139-2150.
Joss, A., Zabczynski, S., Goebel, A., Hoffman, B., Loeffler, D., McArdell, Ch.S., Ternes, Th.A., Thomsen, A.
and Siegrist, H. (2006). "Biological degradation of pharmaceuticals in municipal wastewater treatment:
proposing a classification scheme." Water Research
40(1686-1696).
KNMP (2006). Informatorium Medicamentorum. K. W. Ap. Kujawa-Roeleveld, K. and G. Zeeman (2006). "Anaerobic Treatment in Decentralised and Source-Separation-
Based Sanitation Concepts." Reviews in Environmental Science and Biotechnology
5(1): 115-139.
Lienert, J., Buerki, T. and Escher, B.I. (2007). Reducing micropollutants with source control: substance flow
analysis of 212 pharmaceuticals in feces and urine. Advanced Sanitation Conference, Aachen.
Quintana, B., Weiss, S, and Reemtsma, T. (2005). "Pathways and metabolites of microbial degradation of
selected acidic pharmaceutical and their occurrence in municipal wastewater treated by a membrane
bioreactor." Water Research
39: 2654–2664.
STOWA (2005). "Anaerobic treament of concentrated wasterwater in DESAR concept." Stowa report
2005-14.
Ternes, T. A. a. J., A. (2006). Human pharmaceuticals, hormones and fragrances. The challenge of
micropollutants in urban water management. London, IWA Publishing
WHO, Ed. (2006). ATC classification index with DDDs. Oslo, Collaborating Centre for Drug Statistics
Zeeman, G., Kujawa, K., Mes, de, T., Hernandez, L., Graaff, de, M., Mels, A., Meulman, B., Temmink, H.,
Buisman, C., Lier, van, J., Lettinga, G. (2007). Anaerobic treatment as a core technology for energy, nutrients and water recovery from source separated domestic waste(water). 11th World Congress on Anaerobic Digestion, Brisbane, Australia.
7 PhACs during physical-chemical treatment of
concentrated wastewater streams
In implementation of new sanitation (source separation, sustainable sanitation, decentralised sanitation) concepts much attention is paid to the treatment of separated wastewater streams: urine, feaces, black water, grey water, etc. for elimination of harmful constituents and recovery of resources. Emerging group of compounds present in these streams are human pharmaceuticals (urine, feaces, black water) and personal care products (grey water). The majority of pharmaceuticals administered by humans are excreted with urine – approximately 70%. As urine constitutes a relatively clean wastewater stream of a very small volume it is believed it can be treated efficiently to remove pharmaceutical compounds. In this study urine separately collected from a nursery house/service apartment in the Netherlands was subjected to a number of tests – oxidation with ozone and adsorption to activated carbon, as these technologies seem to have a high potential for removal of refractory compounds. Wastewater, and specifically urine, from a location like an elderly home or a hospital, contains a lot pharmaceutical residues. It is considered that urine from these kinds of locations could be stored and treated periodically with advanced physical-chemical methods to deplete pharmaceutical compounds and lower their overall emissions to the environment. The laboratory batch tests were conducted to determine the potential and efficiency of activated carbon sorption and oxidation with ozone for the selected number of pharmaceutical compounds. These were: Acetylsalicilic acid (aspirine) (ASA), Ibufrofen (IBU), Diclofenac (DCF), Metoprolol (MTP), Carbamazepine (CBZ), Clofibric acid (CFA), Bezafibrate (BZF), Fenofibrate (FNF). For a selection of test compounds a number of criteria were taken into account: consumption, occurrence in aquatic environment, differences in physical-chemical properties (e.g. polarity, hydrophobicity) and suspected biological degradability (persistent, biodegradable), potential eco-toxicological effects and availability of analytical methods, to mention the most important. An attempt has been taken hereby to represent with this selection of eight compounds a broader group of PhACs. Both tested techniques have potential to remove pharmaceutical compounds from a concentrated (waste)water streams. For concentrated streams, such as human urine, optimization of processes in terms of required pre-treatment, sequence of processes and operational parameters is required.
Material and methods
Urine separation
The urine sample used here described tests originated from Sleen, The Netherlands, from a nursery house/service apartment
Leveste/ De Schoel were no-mix toilets of Gustavsberg were installed. The flush water was expected to contribute to the dilution of urine with a factor of 2 a 3. The collected urine was stored in the underground storage tanks.
Pharmaceuticals consumption in nursery houses
The consumption of pharmaceuticals in nursery houses/hospital is generally very high. The list of all administered, by the residents of above mentioned nursery house
Leveste/ De Schoel, pharmaceuticals and supplements is available, together with the amounts taken daily, the therapeutic function a given compound and its excretion. For a new sanitation concept with objective to eliminate pharmaceutical compounds from source separated urine, only these compounds are of interest, which are partially or completely excreted with urine.
Selected test pharmaceuticals
The selected compounds for the laboratory research were: Acetylsalicylic acid (aspirine) (ASA), Ibufrofen (IBU), Diclofenac (DCF), Metoprolol (MTP), Carbamazepine (CBZ), Clofibric acid (CFA), Bezafibrate (BZF), Fenofibrate (FNF). For a selection of test compounds a number of criteria were taken into account: consumption, occurrence in aquatic environment, differences in physical-chemical properties (e.g. polarity, hydrophobicity) and suspected biological degradability (persistent, biodegradable), potential eco-toxicological effects and availability of analytical methods, to mention the most important. An attempt is taken hereby to represent with this selection of eight compounds a broader group of PhACs. This selection was decided as a continuation of earlier research (e.g. chapter 6). In future projects the list of tested pharmaceuticals can be further extended if necessary. The structural formulas of the selected compounds are given in Figure 7.1 while the physical-chemical-biological properties of the selected compounds are given in Table 7.1.
Ibuprofen (IBU) C
Diclofenac (DCF) C
Carbamazepine (CBZ) C
Metoprolol (MTP) C
Clofibric acid (CFA) C10H11ClO3
Bezafibrate (BZF)C19H20ClNO4
Fenofibrate (FNF) C20H21ClO4
Figure 7.1: Chemical and structural formulas of eight selected pharmaceutical compounds for sorption and
oxidation experiments in Sleen project.
Table 7.1. Physical-chemical properties of the selected pharmaceuticals
Pharmaceutical
Therapeutic
Hydrophilic /
pKa value at
kbiol for CAS
hydrophobic
(L/ gSS/d)1
lipid regulating
lipid regulating
lipid regulating
1(Joss 2006), 2(Ternes 2006); 3 (van Beelen 2007)
Main reagents & media
The PhACs were obtained from Sigma-Aldrich (Steinheim, Germany): ASA ≥99.0% (CAS-nr: 50-78-
2), BZF ≥98% (CAS-nr: 41859-67-0), CBZ (CAS-nr: 298-46-4), CFB 97% (CAS-nr: 882-09-7), DCF
(diclofenac sodium salt) (CAS-nr: 15307-79-6), FNF ≥99% (CAS-nr 49562-28-9), IBU ≥98% (GC)
(CAS-nr: 15687-27-1) and MTP as Metoprolol (+)-tartrate salt ≥98% (titration) (CAS-nr: 56392-17-
7).
Six granular activated carbon (GAC) types were tested:
-
NRS CARBON EA 0,5-1,5 is a thermally reactivated extruded carbon, especially developed for wastewater treatment and groundwater remediation. NRS CARBON EA 0,5-1,5 is a durable product with excellent adsorption properties for a range of organic compounds, like COD, colour and organic micropollutants (f.i. detergents, AOX, EOX). On condition that the exhausted carbon complies with the acceptance criteria, NRS CARBON EA 0,5-1,5 is suitable for intake by Norit Recycling Service. Following intake and quality checks the exhausted carbon is reactivated under controlled circumstances at high temperature.
NORIT ROW 0,8 SUPRA is an extruded activated carbon, which offers superior adsorption properties in a wide range of applications such as purification of (potable) water. Its dedicated pore size distribution makes NORIT ROW 0,8 SUPRA highly suitable for the removal of taste and odour, organic micro pollutants such as pesticides, other dissolved organic substances, chlorine and ozone. Its superior hardness makes NORIT ROW 0,8 SUPRA particularly suited for thermal reactivation.
ORGANOSORB 10-AA (DESOTEC Activated Carbon) The activated carbon ORGANOSORB 10 AA is an agglomerated carbon with an ideal porosity. This makes the carbon very suitable for decolourisation and purification of liquids.
ORGANOSORB 11 (DESOTEC Activated Carbon) Standard acid washed coal based carbon for liquid applications.
DARCO 12x20 (Norit) from lignite (biological source), granulated material, used in the purification of potable water and foods. It is frequently used for water purification because of its excellent adsorption capacity for taste and odour causing compounds, colours and algal toxins;
NORIT VAPURE 612 granular activated carbon is recommended for use in gaseous applications involving purification and separation processes. It is a premium grade product manufactured from select grades of coal. As the result of a unique, patented steam-activation process and stringent quality control, NORIT VAPURE 612 granular activated carbon offers superior adsorption properties and is recommended for removal of odors, toxic vapors, irritants, corrosive gases, and to recover solvents and hydrocarbons from various gas streams.
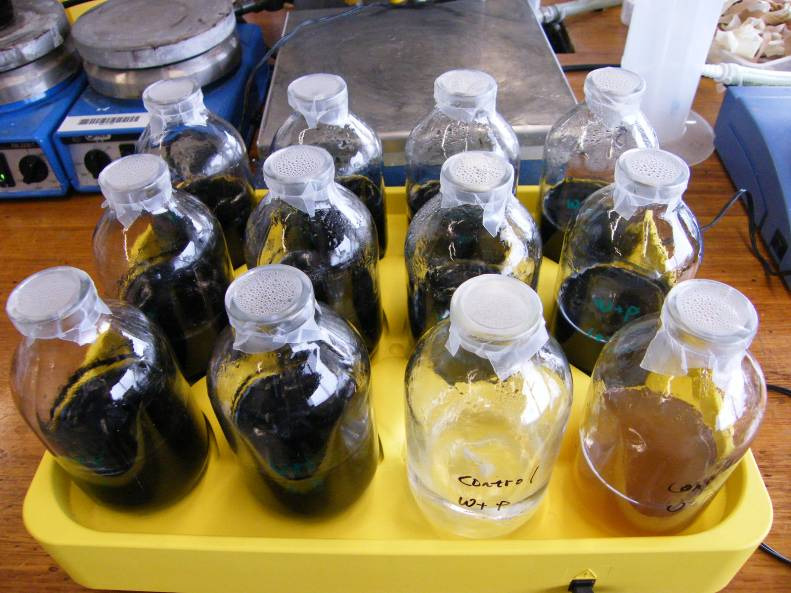
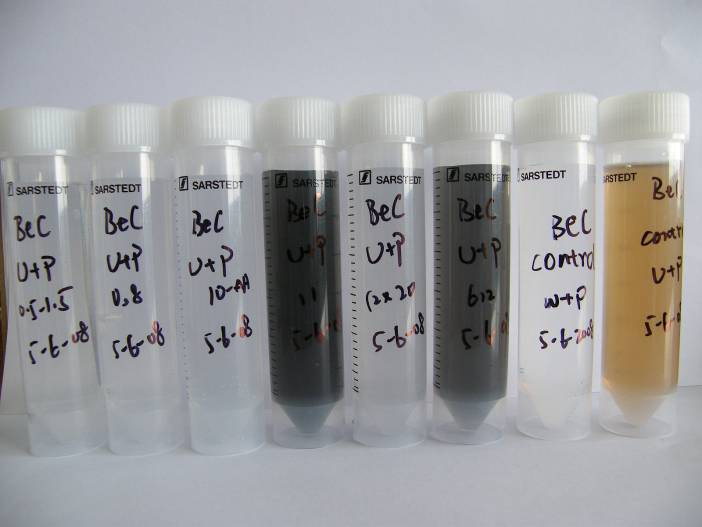
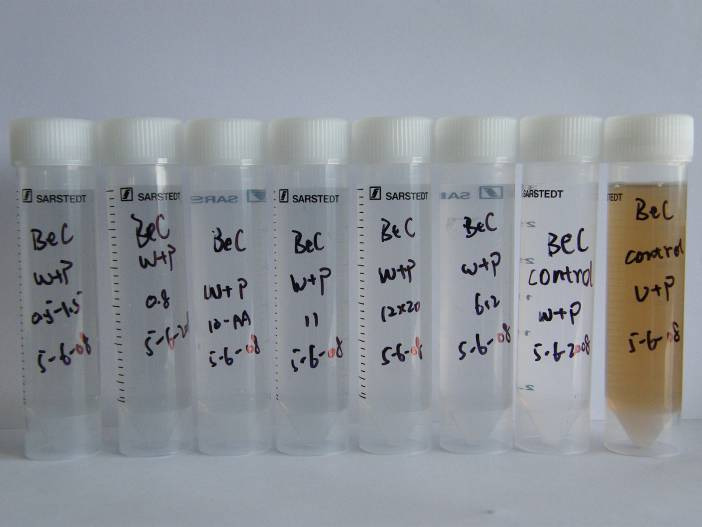
These subsequent types of GAC will be named further on as: GAC 0.5-1.5, GAC 0,8; GAC 10-AA, GAC 11, GAC 12×20, GAC 612 respectively.
Selection of optimal GAC type
The experiments were performed in 200 mL bottles (Figures 7.2-7.4). Two types of experiments were performed (results in Table 7.2): (1) Experiments with Millipore water, pharmaceuticals and GAC To each bottle 99 mL millipore water, 1 mL pharmaceutical stock solution (1000mg/L), 1 g GAC were added. The mixture of water and activated carbon were shaken first for 24 h to get to equilibrium. After pharmaceuticals were spiked the mixtures were shaken for more 18 hours. (2) Experiments with separately collected urine from a nursery home (preserved at 2oC), pharmaceuticals and GAC To each bottle 99 mL urine sample, 1 mL pharmaceutical stock solution (1000mg/L), 1 g GAC were added. The mixture of water and activated carbon were shaken first for 24 h to get to equilibrium. After pharmaceuticals were spiked, the mixtures were shaken for more 18 hours.
Figure 7.2: Flasks containing urine or water, different types of GAC and the same concentrations of
pharmaceuticals.
(1) urine+phar.+GAC
(2) water+phar.+GAC
Figure 7.3: Urine and water samples after contact with GAC, taken after the settling took place ready
to be put in centrifuge (GAC 11 and 612 being very fine type of activated carbon did not settle well)
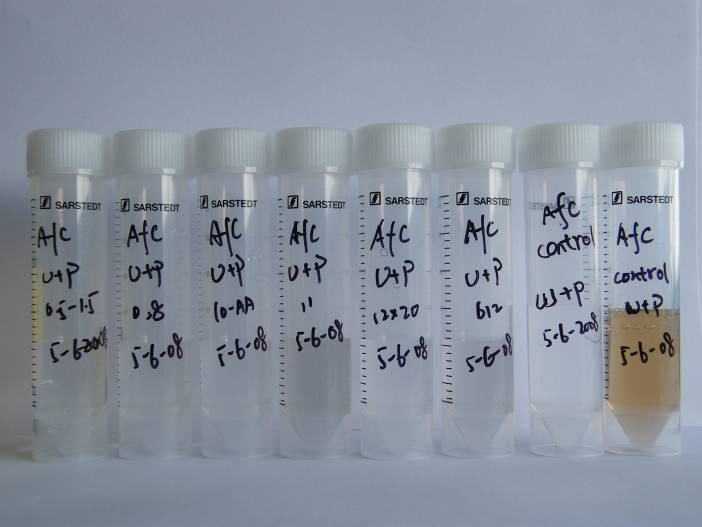
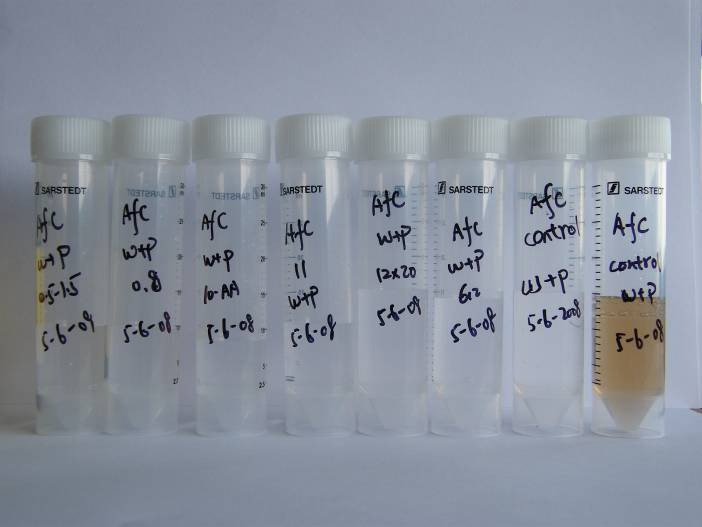
(1) urine+phar.+GAC
(2) water+phar.+GAC
Figure 7.4: Urine and water samples after contact with GAC, taken after the settling and
centrifugation. All suspended GACs (GAC 11 and 612) seem to be separated. The colour of urine is
eliminated (the last sample is control (urine) that was not in contact with GAC).
Table 7.2: Concentration of selected pharmaceuticals (µg/L), after contact with different types of GAC. The
initial concentration in each bottle was approximately 10 mg/L for each pharmaceutical compound
u+p = urine + pharmaceuticals, w+p = water + pharmaceuticals In general the removal of all pharmaceuticals present in excess (10 mg/L each), with different types of GAC was very high. The removal efficiencies were always above 99% (the worst result for 612). The Norrit Vapure 612 performed the worst for the mixture with urine, but satisfactory for the mixture with water. Organosorb 11 performed worse than other types of GAC, for both urine and water. Finally for the further research GAC 12x20 and GAC ROW 0.8 Supra were selected.
Sorption to granular activated carbon
The experiment to asses Freundlich isotherm for selected two types of GAC (Darko 12x20, ROW 0,8 Supra) were performed based on the procedure of Norit with source separated urine and water. Initial concentrations of all pharmaceuticals were approx. 1 mg/L each. Five increasing concentrations of GAC has been applied, viz. 0.5, 1, 2, 5, 10 g/L. The tests were performed in 200 mL flasks (serial method). The volume of liquid in the bottles was 100 mL (99 mL urine or water and 1 mL pharmaceutical stock solution).
The experiment was started by mixing a liquid medium (urine or water) with the required amount of GAC. The equilibrium was established within 24 h. After, the mixtures were spiked with pharmaceuticals and mixed again. The contact time was set to 4 h. After termination of this period, mixing stopped, GAC was allowed to settle. Subsequently the liquid samples were centrifuged, preserved in T = -20oC before sent to analysis for pharmaceutical compounds.
Ozonation of medium containing pharmaceuticals (water,
urine)
From a gas bottle (4 bar) oxygen (O2) was guided through an ozone generator. In this generator part of
oxygen is converted into ozone (O3). Via a spectrophotometer the ozonized air stream is directed to
the bottom of the reaction vessel. The reactor contains a solution (either Millipore water or urine)
which is spiked with 8 selected pharmaceuticals. By means of a magnet-stirrer the ozone gas bubbles
are distributed throughout the reactor. The part of ozone in the reactor which does not react with
organic substrate, leaves the reactor at the upper part. The spectrophotometer registers the outgoing
concentration of ozone, which then is discharged to a gas -lock (filled with a solution of
Sodiumthiosulfate) (Figure 7.5).
Data processing spectrophotometer
m agnetic stirrer
Figure 7.5: Scheme of experimental set-up (see also Figures 7.6-7.7)
The spectrophotmeter measured the absorption (at λ = 254 nm) of ingoing and outgoing ozone. These
absorption values were converted into ozone concentrations and then to total ozone consumption in
the reactor.
During the experiments samples were taken by a needle from the bottom of the reactor. By adding 5
drops of chloroform the samples any biological activity was presumably stopped. Then the samples
were frozen (- 20 °C) before sent to analysis at RIVM for determination of residual pharmaceuticals.
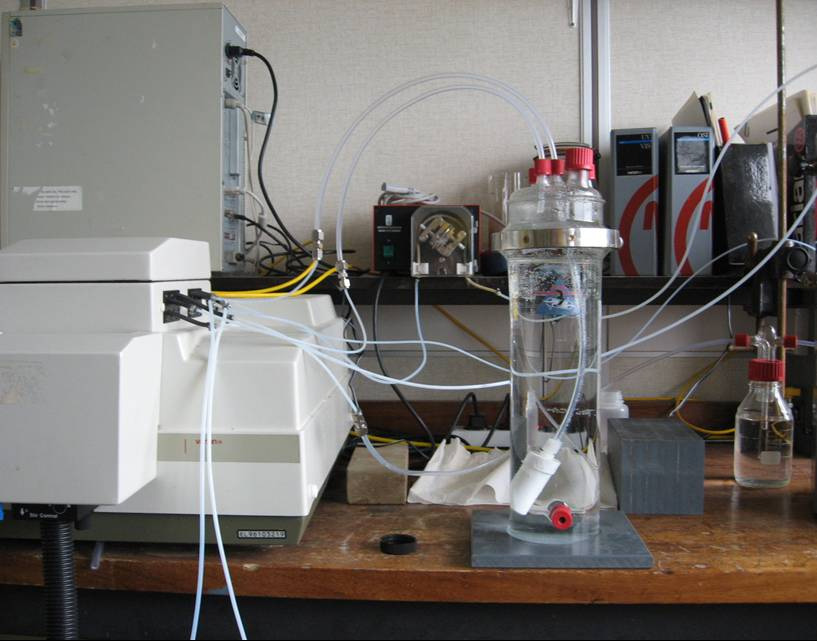
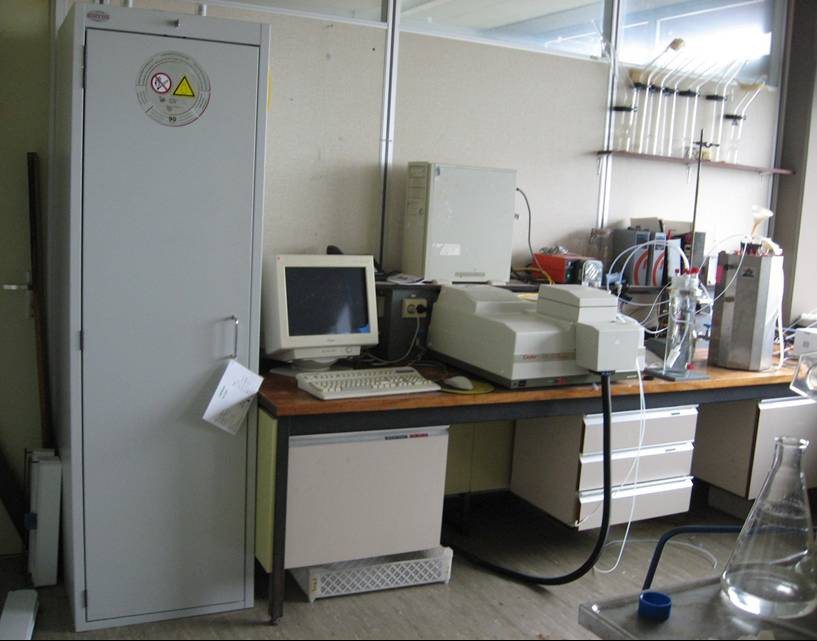
Figure 7.6: Experimental set-up for oxidation of liquid medium (water, urine) with ozone
(oxygen bottle in the closet, computer for data reading, spectrophotometer for O3
concentration, reactor, ozone generator (at the end, white box not to see), mass flow meter
(also not shown).
Figure 7.7: Empty reaction vessel for medium ozonation (still a magnetic stirrer need to be placed
underneath for a better mixing).
Execution of the experiments
The aim of experiments was to get insight in the oxidation capacity of ozone to remove
pharmaceuticals present in urine. For that purpose two tests were run:
Test 1: The ozone was brought to the reaction vessel filled with 2600 ml Millipore water and a
solution of 8 selected pharmaceuticals. The initial concentration per pharmaceutical in the reactor was
1.0 mg/L.
Test 2: Ozone was discharged in the reactor filled with 2600 ml of urine originated from Sleen and a
solution of 8 selected pharmaceuticals. The initial concentration of pharmaceuticals in the reactor was
1.0 mg/L each.
Both tests were performed in four runs: Run 1.1, 1.2, 2.1 and 2.2.
The reason to perform these two types of experiments was to get insight into the matrix effect of urine
on the removal efficiency of pharmaceuticals. The experiment with Millipore water was expected to
reveal a potential of ozone to remove pharmaceutical compounds only.
Stock solution of pharmaceuticals
To add the 8 selected pharmaceuticals to the required medium, a stock solution was first prepared
made in methanol as the pharmaceuticals usually solve poorly in water. Per pharmaceutical 0.065
gram was solved in 50 ml methanol. The concentration of this stock solution is 1.3 g each
pharmaceutical per L.
For the actual experiments at the start of the test a quantity of 2.0 ml stock solution (of 1.3 g/L) was
injected at the bottom of the reactor. As the volume of the reactor was 2600 ml, it resulted in the
initial total concentration per pharmaceutical of 1.0 mg/L (2.0 ml * 1300 mg/2600 ml)
Ozone treatment of pharmaceuticals in Millipore Water (RUN 1.1 and 1.2)
Two runs were executed at similar conditions. The reactor was filled with 2600 ml Millipore water, to
which 2.0 ml of pharmaceuticals' stock solution was added.
Calculations on ozone consumption were performed. The supply of oxygen to the ozone generator in
this experiment was 80 ml O2/min. The spectrophotometer registered the incoming and outgoing
ozone in mol/min. Converted to (incoming) ozone supply per liter content of the reactor this is
approximately 1.7 mg O3/(L*min). The duration of the experiment was 4.5 hours. The samples were
taken at the following time-intervals: 0, 20, 40, 60, 80, 100, 120, 180, 240 and 270 min.
Ozone treatment of pharmaceuticals present in urine (RUN 2.1 and 2.2)
Two runs are executed under similar conditions. The reactor was again filled with 2600 ml urine to
which 2.0 ml stock solution was added. The concentration (per pharmaceutical) in the reactor was 1.0
mg/L.
A difference between the two runs is the concentration of organic compounds in the urine. This aspect
will be further discussed in chapter 4.
For RUN 2.2 he supply of oxygen to the ozone generator was again 80 ml O2/min. Converted to
ozone supply this resulted in approximately 1.7 mg O3/(L*min). The duration of the experiment was
8.0 hours.
In RUN 2.2 samples were taken at the following time-intervals: 0; 20; 40; 60; 80; 100; 120; 180; 240;
300; 360; 420 and 480 min.
Combined treatment of urine GAC followed by ozonation
The laboratory test was performed with combined treatment: sorption on activated carbon followed by oxidation with ozone. Initial concentration of pharmaceuticals (before subjected to sorption) was 1 mg/L for each compound. Two types of GACs were tested: DARCO 12×20 and ROW 0,8. Concentration of GAC in bottles was: 2 g/L Before addition of pharmaceuticals, urine sample was mixed with GAC for 24 h to establish equilibrium. Afterwards pharmaceuticals were added and the mixture was in contact for 24 h. Then the volume of urine was filtered (paper filter) and subjected to ozonation. The max concentration of ozone in the solution was approximately 53 mg/L. During ozonation 8 samples were taken for analysis of pharmaceuticals in the following time intervals: 20, 40, 60, 120, 180, 300, 420 and 600 min. The experimental set-up for ozonation consisted basically of reaction vessel with an active volume of 2.6L, ozone generator, photo-spectrometer and computer as described earlier (§ 3.4). Unfortunately due to analytical errors the obtained results were not clear enough to be further interpreted.
Analysis of pharmaceuticals
Analyses of pharmaceuticals were performed at The National Institute for Public Health and the Environment (RIVM) Laboratory for Food and Residue analysis, European Union Community Reference Laboratory (ARO-CRL).
Materials All chemicals and reagents were of high purity quality. Besides standard laboratory equipment the systems described below were used.
Apparatus
Liquid chromatography (LC): Waters Chromatography Acquity UPLC separation module. Column:
Acquity UPLC BEH C18 1.7 µm (100 * 2.1 mm ID). Column temperature was 65°C. The LC mobile
phase consisted of a mixture of 0.1 percent acetic acid (solution A ) and 100% acetonitrile (solution
B). The gradient used was linear, started at 10% B and progressed to 30% B in 3 minutes after which
it was increased to 100% B in 6 minutes . After 9 min the mobile phase was kept for 2 min at 100% B,
then the percentage B was decreased to 10 percent in 0.01 minute. The mobile phase flow was set at
0.4 ml min-1. The injection volume was 20 µl.
Mass-spectrometer (MS) analysis was carried out on a Waters-Micromass Ultima Platinum.
Depending on compound the measurement was carried out in positive or negative electrospray
ionisation (ESI) mode. In case of co-eluting compounds the ionisation alternates between positive and
negative.
The following settings were used in positive ESI mode: capillary voltage was 3.5 kV. Cone voltage
was 35 V. RF lens 1: 15, aperture: 0.1 and RF lens 2: 0.3. Source temperature was 120°C and
desolvation temperature: 325°C. The cone gas flow was 116 L.h-1 and the desolvation gas flow was
701 L.h-1. LM1/HM1 resolution was 14, with ion energy: 0.8. LM2/HM2 resolution was 14.5, with
ion energy: 1.0. For the collision cell the entrance was 7, with a CE gain of 2 and exit 0. Collision cell
pressure 3.06e-03. See Table 3.11 for the measured MRM transitions.
In negative mode the following settings were used: capillary voltage was 1.2 kV. Cone voltage was 35
V. RF lens 1: 5, aperture: 0.5 and RF lens 2: 1.0. Source temperature was 120°C and desolvation
temperature: 325°C. The cone gas flow was 116 L.h-1 and the desolvation gas flow was 701 L.h-1.
LM1/HM1 resolution was 14, with ion energy: 0. LM2/HM2 resolution was 14.5, with ion energy:
1.0. Collision cell pressure 3.06e-03. For the collision cell the entrance was 10, with a CE gain of 1
and exit 0. See table 7.3 for the measured MRM (multiple reaction monitoring) transitions.
Table 7.3: Pharmaceuticals measured and their corresponding retention time, ionisation mode,
MRM's, dwell time and corresponding collision energy.
Compound
Retention
Ionisation
Dwell time
Collision
time (min)
energy (V)
Acetylsalicylic acid
Sample clean-up Sample clean-up of the liquids was straight forward. The samples were 10 times diluted in LC-eluens A after which they were vortexed for 10 seconds. For samples with lower concentrations the samples were acidified with 2µl 50% acetic acid. The samples were direct injected. Sample clean-up of the soils was performed by a liquid liquid extraction. A portion of the sample (circa 0.5 gram) was weighted and five millilitres of acetonitrile was added. The samples were sonified by an ultrasonic finger for 20 seconds followed by rotating head over head for 10 minutes. After which the sample was centrifuged. The supernatant was transferred to a clean tube and evaporated under nitrogen at 55°C. The dried sample was reconstituted in one millilitre of eluens A, followed by 10 minutes ultrasonification.
Calibration curves To correct for losses due to sample storage and to correct for signal suppression due to matrix compounds the calibration curves were prepared in reprehensive blank materials for each corresponding experiment. In figure 7.8 a chromatogram is shown of a spiked sample containing a mixture of all the pharmaceuticals. Each trace represents the measured transition for the given compounds.
Figure 7.8:
Reversed phase microbore LC-ESI MSMS profiles of an sample spiked (5 ng/ml) with a mixture of pharmaceuticals
Urine composition in terms of macro-parameters
Few macro-parameters were determined for the used urine (Table 7.1.4). As it can be seen in the
Table 4.1 a COD of urine was approximately 1 g/L. Undiluted urine is expected to be characterized by
a COD as high as 8-10 g/L. In this term used urine seems to be 8-10 times diluted. Also concerning
other parameters a similar dilution factor could be derived: for ammonia, chlorides, potassium.
Table 7.4: Macro parameters measured in used for experiments urine
Parameters, unit
Theoretical expected, undiluted urine
Used urine
CODtotal , (mg/L)
Chloride, mgCl-/L
4757 (Grifith et al., 1976, after Wilsenach, 2006); 5600
Sulphate, mgSO4/L
1550 (the same source as above)
Qualitative analysis of used urine for the presence of
pharmaceutical compounds
The used for the tests urine was ‘screened for the presence of pharmaceuticals, other than the 8
primarily selected for laboratory research based on the list of pharmaceuticals being currently
administered at the location. Only the qualitative analysis was performed (Table 7.5). As it can be
seen a broad spectrum of various pharmaceuticals has been consumed a nursing home.
Table 7.5: Pharmaceutical compounds quantitatively determined in the tested urine. As it can be seen there is a
little overlap between previously selected 8 pharmaceuticals and pharmaceutical consumed from the location.
Chemical formula
Ascal cardio Brisp
Bisoprol fum. merc
Carbasalaat ca. card.
Chloortalidon RP
Enalapril maleaat
Hydrochloorthiazide
Isosorbide din. Ret.
Perindopr.tert.-Bu
Preliminary experiments ozonation of urine
The objective of this experiment was to test the ozonation set-up. A urine sample was subjected to oxidation with ozone. The O2 flowrate was established at 80 mL/L, resulting in O3 concentration of
0.028 mg/L. During this preliminary test COD of urine was determined in samples taken in time intervals and pH (Figure 7.9)
Figure 7.9: Oxidation of diluted urine from Meppel with ozone measured by depletion of COD (solid
line)
Slight reduction of COD observed (35% in 3 hours) could be because of the low oxidation capacity of
the used set-up and/or the nature of the remained COD in the old urine sample.
Ozone treatment of spiked Millipore Water
RUN 1.1 The results of RUN 1.1, in which the 8 selected pharmaceutical compounds have been solved in Millipore water at an initial concentration of 1 mg/L, are shown in Figure 7.10 and 7.1.11. Of the 8 represented pharmaceuticals it is noticeable that clofibric acid and ibuprofen take the longest before they are totally removed (respectively 420 and 480 minutes). The remaining pharmaceuticals are oxidised by ozone in an earlier stage. Acetylsalicylic acid is removed entirely after 250 minutes. Metoprolol, bezafibrate and fenofibrate are oxidized after 120 minutes. For the most persistent compounds, in traditional biological treatment, carbamazepine and diclofenac it takes only 40 minutes for their significant reduction (99.98 and 99.3 respectively).
Figure 7.10: Depletion of original pharmaceutical compounds (metoprolol, acetyl salicylic acid,
carbamazepine and clofibric acid) in Millipore water subjected to oxidation with ozone in RUN 1.1.
Figure 7.11: Depletion of original pharmaceutical compounds (bezafibrate, diclofenac, ibuprofen and
fenofibrate) in Millipore water subjected to oxidation with ozone in RUN 1.1.
RUN 1.2
The results of RUN 1.2, in which Millipore water was again spiked with the selected 8
pharmaceuticals at an initial concentration of 1 mg/L are shown in Figure 7.1.12 and 13. Data from
fenofibrate in this run is lacking because of an analytical error. Of the 7 represented pharmaceuticals it
is notable that acetylsalicylic acid is hardly decreasing in time. Also in the previous run (RUN 1.1)
acetylsalicylic acid was exposing irregular pattern. This could be also because of analytical
difficulties to analyse this specific compound). The remaining pharmaceuticals are, however, well
oxidised by ozone. Carbamazepine and diclofenac were again very quickly oxidised with ozone,
already within first 20 minutes of the test (99 and 96% reduction). Also metoprolol and bezafibrate
were quickly removed (after 40 minutes 98 and 95% respectively were reduced). After 180 minutes
clofibric acid and ibuprofen are also far removed.
Acetylsalicylic acid
Figure 7.12: Depletion of original pharmaceutical compounds (metoprolol, acetylsalicylic acid,
carbamazepine, clofibric acid) in Millipore water subjected to oxidation with ozone in RUN 1.2.
Figure 7.13: Depletion of original pharmaceutical compounds (bezafibrate, diclofenac, ibuprofen) in
Millipore water subjected to oxidation with ozone in RUN 1.2.
Ozone consumption during the tests
In Figure 7.14 the data of the spectrophotometer revealing ozone consumption of RUN 1.2 were
processed.
Ozone consumption RUN 1.2 (spiked Millipore Water)
ozone consumption
ozone input (mg/min)
Figure 7.14: Ozone input (upper line) and ozone consumption (curve) during RUN 1.2 (spiked
Millipore Water)
After 180 minutes 6 of the 7 analysed pharmaceuticals (only not Acetylsalicylic acid) are entirely
removed by ozone. The marked surface in Figure 4.6 represents the total amount of ozone consumed
after this 180 minutes period. The marked surface corresponds with 203 mg O3/L. The concentration
of each pharmaceutical at the beginning of the experiments is 1 mg/L. This means for the total
removal of the 6 mentioned pharmaceuticals (6 x 1 mg/L, 203 mg O3/L was needed in situation when
there is any matrix disturbance. Simplifying, on average 34 mg O3 was needed to oxidise 1 mg of
given pharmaceutical.
Of the total amount of 306 mg/L of incoming ozone, 103 mg O3 (306-203) was not used for the
oxidation process and left the reactor.
Ozone treatment of spiked urine (RUN 2.1)
RUN 2.1
The results of RUN 2.1, in which urine was spiked with 8 pharmaceuticals at an initial concentration
of 1 mg/L of each, are shown in Figure 7.1.15 and 16. All 8 pharmaceuticals were reduced at varying
degree over a time period of 600 minutes. This reduction was significantly slower and lower than in
the test with Millipore water. The following reduction degrees were measured: 22 % (clofibric acid),
25% (bezafibrate), 32% (metoprolol), 42% (ibuprofen), 43% (carbamazepine), 65% (diclofenac), 88%
(acetylsalicylic acid) and 99% (fenofibrate).
(Trace) organic compounds, with concentration ranging from mg/L to µg/L are ozone-consumed
substances in urine. This could be the reason that a longer reaction time and a higher total ozone
supply are necessary for the removal of the pharmaceuticals in a more complex matrix of urine. This
suggests that for efficient process performance urine could be first pre-treated to remove a major
fraction of lowering the ozonation efficiency organic matter. The possible processes could be for
instance a pre-aeration or filtration on a (coarse) granular activated carbon.
This complex matrix of urine could be also the reason of insufficient extraction of the compounds
revealing in lower than expected initial concentration of the compounds (at t=0, each pharmaceutical
compound should be around 1000 µg/L) and irregular pattern of the depletion curves.
MetoprololAcetyl salicylic acid
Figure 7.15: Depletion of original pharmaceutical compounds (metoprolol, acetylsalicylic acid,
carbamazepine, clofibric acid) in spiked Sleen urine subjected to oxidation with ozone in RUN 2.1
Figure 7.16: Depletion of original pharmaceutical compounds (bezafibrate, diclofenac, ibuprofen,
fenofibrate) in spiked Sleen urine subjected to oxidation with ozone in RUN 2.1.
RUN 2.2
The results of RUN 2, in which the 8 pharmaceuticals were spiked to urine with a concentration of 1
mg/L, are shown in Figure 7.1.18 and 19. Besides from fenofibrate (Figure 7.17) no significant
decrease of the concentration of pharmaceuticals in time was observed. The difference between RUN
2.1 and RUN 2.2 can be explained by the urine characteristics used for this test. Urine used in RUN
2.2 was much more concentrated that that one used in RUN 2.1, as it concerned last available sample
containing elevated amounts of suspended material. This might be the reason that the detection of the
pharmaceuticals during the analyses was quite difficult and their oxidation was poor as other organic compounds were utilizing O3. These results suggest that to remove pharmaceutical compounds from pure urine much higher O3 dosages should be used or the urine should be first pre-treated. Another option could be implementation of advanced oxidation, in which more oxidation techniques are combined in one process. This however could not be validated within these tests.
Figure 7.17: Depletion of original pharmaceutical compounds (metoprolol, acetylsalicylic acid, carbamazepine,
clofibric acid) in spiked Sleen urine (more concentrated than in RUN 2.1 regarding organic matter content)
subjected to oxidation with ozone in RUN 2.2.
Figure 7.18: Depletion of original pharmaceutical compounds (bezafibrate, diclofenac, ibuprofen, fenofibrate)
in spiked urine ((more concentrated than in RUN 2.1 regarding organic matter content) subjected to oxidation
with ozone in RUN 2.2.
Ozone consumption during RUN 2.2
In Figure 7.19 the data from the spectrophotometer of RUN 2.2 is processed. The upper line reflects
ozone supply and the curve ozone consumption in the reactor during the experiment. As it can be seen
ozone consumption in that test was of 100% of the total input. This was probably caused by a higher
concentration of organic compounds in the urine used causing consumption of all available ozone.
Ozone consumption RUN 2.2 (spiked Urine)
ozone consumption
ozone input (mg/min)
Figure 7.19 Ozone input and ozone consumption during RUN 2.2 (spiked urine)
4.6 GAC treatment of spiked urine
In Figures 7.20-23 adsorption of pharmaceuticals in function of a GAC dose is shown. Two granular
activated carbon types were used in this research: DARCO 12x20 and SUPRA 0.8. The initial
concentration of pharmaceuticals was approximately 1 mg/L. As the sorption took place immediately,
rarely this initial concentration could be revealed from the first measurement. From Figures it can be
seen that at the concentration of DARCO 12x20 GAC type of 2 g/L, all pharmaceuticals except
clofibric acid are absorbed from a spiked urine solution. For metoprolol, acetylsalicylic acid and
fenofibrate this was even less (around 0.5 g GAC/L). Clofibric acid required somewhat higher dose of
GAC. For GAC DARCO 12x20 on average 250 mg GAC (2000mg/L/(8* 1 mg/L) was needed to
absorb 1 mg of pharmaceutical.
Figure 7.20: Sorption of pharmaceutical compounds (metoprolol, acetylsalicylic acid, carbamazepine, clofibric
acid), shown as depletion of a given compound from a liquid phase, from spiked Sleen urine onto granular
activated carbon DARCO 12x20 as a function of GAC dose.
c, mn 500tiotra 400
Figure 7.21: Sorption of pharmaceutical compounds (bezafibrate, diclofenac, ibuprofen, fenofibrate), shown as
depletion of a given compound from a liquid phase, from spiked urine onto granular activated carbon DARCO
12x20 as a function of GAC dose.
For SUPRA 0.8 similar results were obtained. Majority of pharmaceuticals at initial concentration of
1 mg/L ‘disappeared' from the liquid phase already at GAC dose of 2 or less g/L. Exception was
again clofibric acid (more than 5 g/L) and ibuprofen (> 4 g/L).
Figure 7.22: Sorption of pharmaceutical compounds (metoprolol, acetylsalicylic acid, carbamazepine, clofibric
acid) shown as depletion of a given compound from a liquid phase, from spiked Sleen urine onto granular
activated carbon SUPRA 0.8 as a function of the GAC dose.
c, mn 500tiotra 400
Figure 7.23: Sorption of pharmaceutical compounds (bezafibrate, diclofenac, ibuprofen, fenofibrate), shown as
depletion of a given compound from a liquid phase, from spiked Sleen urine onto granular activated carbon
SUPRA 0.8 as a function of the GAC dose.
In addition sorption tests were performed with two types of GAC (DARCO 12x20 and SUPRA 0.8)
and Millipore water containing pharmaceuticals at the initial concentration of 1 mm/L each. The
results are shown in Figure 7.1.25. The patterns of compounds depletion are quite similar, although
the considered compounds ‘disappear' from the liquid phase at a lower GAC dose. The absence of
other organic matter competing for available adsorption sites, seems to have a positive effect on the
removal of more polar compounds such as clofibric acid and ibuprofen.
Figure 7.24: Sorption of all pharmaceutical compounds, shown as depletion of a given compound from a liquid
phase, from spiked Millipore water urine onto two types of granular activated carbon: DARCO 12x20 (above)
and SUPRA 0.8 (below) as a function of the GAC dose.
Discussion and Conclusions
The eight selected pharmaceuticals: acetylsalicylic acid (aspirine) (ASA), ibufrofen (IBU), diclofenac (DCF), metoprolol (MTP), carbamazepine (CBZ), clofibric acid (CFA), bezafibrate (BZF), fenofibrate (FNF)are all potentially well oxidized by ozone. Presence of, other than pharmaceuticals, organic compounds in treated matrix slowed down or even inhibited process of oxidation of most of pharmaceuticals at applied low O3 dose. An ozone input in the ozonation tests described in this report was 1.7 mg/L/min. (Nguyen) in their tests on ozonation of elevated concentrations of a cocktail of pharmaceuticals (60 mg/L level) dissolved in a synthetic urine applied O3 input of 10 mg/L/min. From the investigated PhACs, 6 PhACs were sufficiently degraded after 2h by using the selective oxidant alone at a mentioned dosage, some pharmaceuticals were only removed partially. Various organic compounds that are present in urine matrix seem to have significant effects on the reactions. To lower the matrix effect and optimise ozonation a pre-treatment (e.g. biological, filtration) could be considered. The effect of applying combined oxidation techniques (advanced oxidation processes, AOP) on enhancement of oxidation of pharmaceuticals was not investigated in this study. For biologically treated wastewater with dissolved organic carbon (DOC) between 8 and 23 mgDOC/L, an ozone dose of 2 to 10 mgO3/L should be sufficient to remove majority of pharmaceuticals to 90-99% (Huber 2003) (Ternes 2003). From recent investigations with urine, it was concluded that complete oxidation of a representative set of micropollutants including pharmaceuticals and synthetic hormones may be achieved. Despite the quenching of oxidants by the
organic matrix in urine, it was shown that all the tested compounds could be transformed completely. At an ozone dose of 1.1 gO3/l, fast-reacting compounds such as ethinylestradiol were completely removed, while removal of more recalcitrant compound such as ibuprofen was 80% (Pronk 2006). Analysis of the results showed that oxidation took place directly by ozone as well as by OH radicals. Considering the high reactivity of the OH radicals with most organic micropollutants, ozonation can be regarded as a suitable method for removing a wide range of micropollutants from urine. With respect to the analyses and detection of pharmaceuticals we can assume that the higher the concentration of organic compound (in urine), the more difficult it is to get accurate results and better extraction techniques are needed. Sorption of pharmaceuticals on granular activated carbon (GAC) occurred for all tested pharmaceuticals. For more polar compounds such as clofibric acid a higher dosis of GAC was required. Both tested techniques, activated carbon sorption and ozonation, are appropriate to remove pharmaceutical compounds from various water matrixes. For economical reasons the sequence of the processes and implementation of pre-treatment techniques should be considered for more concentrated wastewater streams such as urine or black water.
8 PhAC removal from concentrated wastewater streams
Black water
Black water (feaces, urine, flush water), especially when collected with a minimum amount of flush water, constitutes a concentrated medium in terms of organic material, solids, nutrients and pharmaceuticals. Organic material can be removed and converted to biogas (energy carrier) by means of anaerobic digestion. As anaerobic digestion is not efficient towards removal of nutrients and micro-pollutants an additional step has to follow. In simplified application the content of the digestors (sludge, effluent) could be used in agriculture. The caution has to be taken however as both media are not stabilised. The fate of pharmaceuticals ‘on the fields' and its effects on the crops is not completely clear yet. Most probably sorption and in the course of time degradation of many pharmaceuticals would take place. In order to polish the anaerobic effluent an aerobic step could be added in form of:
suspended activated sludge;
suspended activated sludge with membrane filtration (MBR);
fixed activated sludge – biofilm (trickling filter, rotating biological contactor (RBC), submerged filter, downflow hanging sponge (DHS);
natural and semi-natural systems (facultative and maturation ponds, (constructed) wetland systems, aquatic plants).
In all of these systems removal of nutrients can be accomplished and further removal of organic material. Also further degradation of pharmaceuticals will take place; the degree will depend on the system used and process conditions. The complete removal of all pharmaceutical compounds cannot be assured in aerobic treatment systems. If the objective is to eliminate the pharmaceuticals compounds to a low level, only advanced physical-chemical techniques can assure it. In order to optimise efficiency of these systems one has to take sure that the influent has a very good quality, especially in terms of organic material and suspended material (as low as possible). Advanced physical techniques, which could be potentially applied and good results have been attained so far are:
oxidation techniques (ozone, advanced oxidation processes: combinations of O3, UV, H2O2, TiO2);
sorption on activated carbon, and
tight filtrations: nanofiltration and reverse osmosis.
As much experience have been gained already with above mentioned techniques in production of drinking water, their application in wastewater treatment is quite limited. Very little experience, if any, has been gained with application of advanced physical-chemical techniques for treated black water in order to remove pharmaceutical compounds. A general scheme for a recommended treatment of source separated black water has been presented schematically in Figure 8.1.
Nutrients, solids,Organic material,
Nutrient rich sludge
Figure 8.1: A sequence of processes to apply for black water treatment in order to eliminate human
pharmaceuticals emissions into the environment.
Urine is produced in small quantities (1-1.5 L/person/day) and can be collected undiluted. Although considered as a relatively clean it still contains a significant load of organic material, nutrients, pathogens and pharmaceuticals. Regarding the small volume of the urine in certain situation it could be treated separately using advanced physical-treatment at a relative high costs. Such a solution could be applied in situation when point sources emit elevated concentration of pharmaceuticals into the environment (hospitals and other health care centres, pharmaceutical industries). A train of processes applying aerobic biological degradation followed by advanced physical-chemical treatment could be applied as well. It has an advantage that a bulk of organic material is for a large part eliminated, allowing for optimisation of a post-treatment in terms of lower doses of reagents, contact times, energy consumption, etc. When urine is used, after a storage, as a fertiliser in agriculture, the pharmaceuticals will be removed, at least partially, as a consequence of the spectrum of (natural) processes in the course of time:
sorption on soil particles, roots,
biodegration in soil;
exposure to sun – photolytic degradation.
The possible treatment configuration for separated collected urine are scetched in Figure 8.1.2.
high reagents, energy inputcontact times
nutrients, solids,organic material,pathogens
Low parmaceuticals
nutrients, solids,organic material,
Application as fertiliser
plants, ground water resources
Processes in soil
Figure 8.2: Possible solutions for source separated urine for elimination/reduction of human
pharmaceuticals emissions into the environment.
Literature chapter 7 and 8
Huber, M. M., Canonica, S., Park , G.Y. and Gunten, von, U. (2003). "Oxidation of Pharmaceuticals during
Ozonation and Advanced Oxidation Processes." Environ. Sci. Technol. 37: 1016-1024.
Joss, A., Zabczynski, S., Goebel, A., Hoffman, B., Loeffler, D., McArdell, Ch.S., Ternes, Th.A., Thomsen, A.
and Siegrist, H. (2006). "Biological degradation of pharmaceuticals in municipal wastewater treatment: proposing a classification scheme." Water Research 40(1686-1696).
Nguyen, L. V. Treatment of high concentration of pharmaceuticals in urine by ozonation processes, Hokkaido
Pronk, W., Biebow, M. and Boller, M. (2006). "Treatment of source-separated urine by a combination of bipolar
electrodialysis and a gas transfer membrane." Water Science and Technology 53(3): 139–146.
Ternes, T., A., Joss, A. (2006). Human pharmaceuticals, hormones and fragrances. The challenge of
micropollutants in urban water management., IWA Publishing.
Ternes, T. A., Jeannette Stuber, Nadine Herrmann, Derek McDowell, Achim Ried, Martin Kampmann,
Bernhard Teiser (2003). "Ozonation: a tool for removal of pharmaceuticals, contrast media and musk fragrances from wastewater? Rapid communication." Water Research 37: 1976–1982.
van Beelen, E. S. E. (2007). Municipal Waste Water Treatment Plant (WWTP) Effluents a Concise Overview of
the Occurrence of Organic Substances. A. o. R. W. RIWA.
Source: http://www.switchtraining.eu/fileadmin/template/projects/switch_training/files/Resources/Kujawa-Roeleveld_2011_Removal_of_pharmaceuticals_compounds_from_concentrated_wastewater.pdf
International Bulletin of Pharmaceutical Sciences 2012, 1 (1-2): 17–29 International Bulletin of Pharmaceutical Sciences Article Type: Minireview Use of Metabolomics for Monitoring Metabolic Responses Caused by Drug Administration 2. Application of Metabolomics in the Study of Metabolic Changes Induced by Drugs
Asian J Androl 2006; 8 (2): 219–224 .Clinical Experience .Long-term treatment with intracavernosal injections in diabetic men with erectile dysfunction P. Perimenis, A. Konstantinopoulos, P. P. Perimeni, K. Gyftopoulos, G. Kartsanis, E. Liatsikos, A. Athanasopoulos Department of Urology, University Hospital, 26500 Patras, Greece Aim: To assess the behavior of patients with diabetes mellitus (DM) and erectile dysfunction (ED) during 10 con-secutive years of treatment with self-injection of vasoactive drugs. Methods: Thirty-eight diabetic men, including 12with type I and 26 with type II diabetes, were followed up regularly for 10 years after they began self-injecting forsevere ED. Real time rigidity assessment was used for the objective determination of the initial dosage and then doseswere regulated in order to introduce an erection suitable for penetration and maintenance of erection for approximately30 min. Patients were followed up every two months, and doses were increased only when the treatment responsewas not satisfactory. Results: The number of injections used per year by the patients was reduced each year (meannumbers: 50 in the first year and 22.5 in the 10th) and treatment shifted towards stronger therapeutic modalities(mixtures of vasoactive drugs instead of prostaglandin E1 alone). Type I diabetic men were standardized to a level oftreatment as early as 5 years after the initiation of treatment. That level was finally reached by type II patients afteranother 4-5 years. Conclusion: Treatment with self-injections of vasoactive drugs in diabetic men with severe ED isa safe and effective alternative in the long term. Diabetic men of both types show the same preferences in quality andquantity of treatment after 10 years. The key point for maintenance in treatment is the adjustment of the therapeuticmethod and dosage to optimal levels for satisfactory erections. (Asian J Androl 2006 Mar; 8: 219–224)