Magnetoterapia-weterynaria.pl
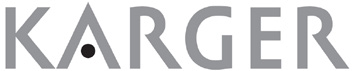
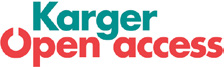
Cell Physiol Biochem 2015;37:651-665
DOI: 10.1159/000430384
2015 S. Karger AG, Basel
Published online: September 08, 2015
Spaas et al.: Chondr
ogenic Priming Enhances MSC Adhesion
ed: August 04, 2015
This is an Open Access article licensed under the terms of the Creative Commons Attribution-
NonCommercial 3.0 Unported license (CC BY-NC) (www.karger.com/OA-license), applicable to
the online version of the article only. Distribution permitted for non-commercial purposes only.
Chondrogenic Priming at Reduced Cell
Density Enhances Cartilage Adhesion
of Equine Allogeneic MSCs - a Loading
Sensitive Phenomenon in an Organ Culture
Study with 180 Explants
Jan H. Spaasa Sarah Y. Broeckxa Koen Chiersb Stephen J. Fergusonc,d
Marco Casarosac Nathalie Van Bruaenea Ramses Forsythe Luc Duchateauf
Alfredo Franco-Obregóng,h,i Karin Wuertz-Kozak c,d
aGlobal Stem cell Technology, ANACURA group, Evergem, Belgium; bDepartment of Pathology, Bacteriology and Poultry Diseases, Faculty of Veterinary Medicine, Ghent University, Merelbeke, Belgium; cInstitute for Biomechanics, ETH Zurich, Zurich, Switzerland; dCompetence Center for Applied Biotechnology and Molecular Medicine CABMM, University of Zurich, Zurich, Switzerland; eDepartment for Anatomo-pathology, Brussels University Hospital, Brussels, Belgium; fDepartment of Comparative Physiology and Biometrics, Faculty of Veterinary Medicine, Ghent University, Merelbeke, Belgium; gDepartment of Surgery, Yong Loo Lin School of Medicine, National University of Singapore, Singapore; hDepartment of Physiology, National University of Singapore, Singapore; iNational University Hospital Sports Centre, National University of Singapore, Singapore
Key Words
MSCs • Cartilage • Chondrogenic • Horse • Peripheral Blood
Background: Clinical results of regenerative treatments for osteoarthritis are becoming
increasingly significant. However, several questions remain unanswered concerning
mesenchymal stem cell (MSC) adhesion and incorporation into cartilage. Methods: To this end,
peripheral blood (PB) MSCs were chondrogenically induced and/or stimulated with pulsed
electromagnetic fields (PEMFs) for a brief period of time just sufficient to prime differentiation.
In an organ culture study, PKH26 labelled MSCs were added at two different cell densities (0.5
x106 vs 1.0 x106). In total, 180 explants of six horses (30 per horse) were divided into five groups:
no lesion (i), lesion alone (ii), lesion with naïve MSCs (iii), lesion with chondrogenically-induced
MSCs (iv) and lesion with chondrogenically-induced and PEMF-stimulated MSCs (v). Half of
the explants were mechanically loaded and compared with the unloaded equivalents. Within
each circumstance, six explants were histologically evaluated at different time points (day 1,
J.H. Spaas and S.Y. Broeckx share first authorship. A. Franco-Obregón and K. Wuertz-Kozak share last authorship.
Jan H. Spaas
Global Stem cell Technology, ANACURA group, Noorwegenstraat 4, 9940 Evergem,
and Karin Wuertz-Kozak
(Belgium), or Institute for Biomechanics, ETH Zurich, and Competence Center for
Applied Biotechnology and Molecular Medicine CABMM, University of Zurich, Zurich,
(Switzerland), E-Mail [email protected], E-Mail [email protected]
198.143.55.1 - 10/27/2015 12:26:51 PM
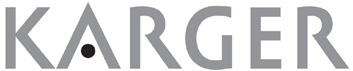
Cell Physiol Biochem 2015;37:651-665
DOI: 10.1159/000430384
2015 S. Karger AG, Basel
Published online: September 08, 2015
Spaas et al.: Chondrogenic Priming Enhances MSC Adhesion
5 and 14). Results: COMP expression was selectively increased by chondrogenic induction
(p = 0.0488). PEMF stimulation (1mT for 10 minutes) further augmented COL II expression
over induced values (p = 0.0405). On the other hand, MSC markers remained constant over
time after induction, indicating a largely predifferentiated state. In the unloaded group, MSCs
adhered to the surface in 92.6% of the explants and penetrated into 40.7% of the lesions.
On the other hand, physiological loading significantly reduced surface adherence (1.9%) and
lesion filling (3.7%) in all the different conditions (p < 0.0001). Remarkably, homogenous cell
distribution was characteristic for chondrogenic induced MSCs (+/- PEMFs), whereas clump
formation occurred in 39% of uninduced MSC treated cartilage explants. Finally, unloaded
explants seeded with a moderately low density of MSCs exhibited greater lesion filling (p =
0.0022) and surface adherence (p = 0.0161) than explants seeded with higher densities of
MSCs. In all cases, the overall amount of lesion filling decreased from day 5 to 14 (p = 0.0156).
Conclusion: The present study demonstrates that primed chondrogenic induction of MSCs at
a lower cell density without loading results in significantly enhanced and homogenous MSC
adhesion and incorporation into equine cartilage.
Copyright 2015 S. Karger AG, Basel
Osteoarthritis, also known as degenerative joint disease (DJD), is a pathology
characterized by degenerative and sometimes hypertrophic changes of bone and cartilage,
resulting in progressive apposing of joint surfaces causing pain and joint distortion. In the
US between the years of 2010 and 2012, 52.5 million adults (22.7%) demonstrated doctor-
diagnosed arthritis [1]. By 82 years of age, as high as 50% of the population will develop
knee osteoarthritis [2], with greater morbidity in modern societies [3]. Also in horses, 60%
of locomotory disorders are correlated with osteoarthritis [4], which is considered a major
economic loss for the industry [5, 6].
The evolutionary distance between human and rhesus monkey genome is estimated
to be around 35 million years, whereas this would be 80 million years for rodents [7].
Large animals, such as horses and dogs, are located somewhere in between [8]. Histological
evaluation of horse, goat, sheep, dog, and rabbit stifle joint cartilage demonstrated closest
proximity of equine stifle cartilage to its human equivalent [9]. Therefore, horses may
be considered as a relevant pre-clinical animal model for human therapies. Also the FDA
reported in 2005 the use of equine cartilage studies for assessing clinical endpoints in
human clinical trials [10].
Recently, it has been shown that pulsed electromagnetic field (PEMF) treatment of
injured rabbit knees can significantly improve histological scores and hyaline cartilage
formation after 6 weeks of daily PEMF treatment for one hour a day [11]. In 28 elderly
humans with bilateral knee osteoarthritis, PEMF stimulation at 3 times per week over 6
weeks significantly improved pain, stiffness, and physical function in comparison to the
untreated contralateral joint [12]. Trock et al. found that treating human patients with knee
osteoarthritis (n=86) with PEMFs for a total of 18 times caused significant differences in
pain, pain on motion, and tenderness in comparison to placebo treatments [13]. Interestingly,
PEMF stimulation of human umbilical cord-derived mesenchymal stem cells (MSCs) was
able to enhance proliferation and accelerate chondrogenic differentiation and extracellular
matrix production in vitro [14] and similar beneficial effects were observed for adipose-
derived MSCs in both, 2D and 3D culture [15].
It was shown that experimentally induced cartilage lesions in horses treated with
autologous adipose tissue or bone marrow-derived MSCs in combination with hyaluronic
acid or fibrin hydrogels revealed only modest clinical improvements in comparison to the
placebo treatment group, however, with enhanced tissue repair and increased ACAN levels
[16-18]. Rather than using uninduced MSCs, several researchers have chosen to perform
198.143.55.1 - 10/27/2015 12:26:51 PM
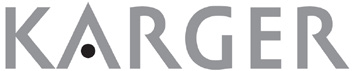
Cell Physiol Biochem 2015;37:651-665
DOI: 10.1159/000430384
2015 S. Karger AG, Basel
Published online: September 08, 2015
Spaas et al.: Chondrogenic Priming Enhances MSC Adhesion
chondrogenic predifferentiation of MSCs, which resulted in enhanced therapeutic effects
In order to provide more insights in the aforementioned findings, the present study
consists of a series of combined cell culture and organ culture experiments. Firstly, equine
peripheral blood (PB) MSCs were exposed to chondrogenic induction with and without
PEMF treatment and changes in the mRNA expression of chondrogenic markers relative
to untreated PB-MSCs was analysed after 6, 24 and 72 hours (n=3). In the second part,
lesions were introduced into stifle joint cartilage explants of six horses, followed by topical
application of allogeneic PB-MSCs obtained from one donor horse, at two different seeding
densities. Five different groups were considered at three time points (day 1, 5 and 14) with
or without loading: no lesion (i), lesion alone (ii), lesion with naïve MSCs (iii), lesion with
chondrogenically-induced MSCs (iv) and lesion with chondrogenically-induced and PEMF-
stimulated MSCs (v).
Materials and Methods
Cell Culture StudyIsolation and expansion of MSCs. In total, 50 ml of blood was collected in sterile EDTA tubes from the
vena jugularis of three different adult donor horses, which were tested for different transmittable diseases at
Böse laboratory (Harsum, Germany), as previously reported [22]. Approval of Global Stem cell Technology's
ethics committee was obtained (EC_2012_001). In order to isolate mesenchymal stem cells (MSCs), the
blood sample was centrifuged at 1000 G for 20 minutes and the buffy coat was collected and diluted 1:2 in
phosphate buffered saline (PBS) 1x. Afterwards, this suspension was gently layered on an equal amount of
Percoll® density gradient (GE Healthcare) and isolated cells were further cultured in expansion medium
consisting of DMEM low glucose + 20% FCS + 50 units/ml penicillin, 50 µg/ml streptomycin and 125 ng/ml
amphotericin at 37°C and 5% CO2 and characterized as MSCs [23]. During the expansion phase, the culture
medium was changed every two days and cells were subcultured at 70% confluency with 0.25% trypsin. All
three horses were used in the cell culture study, whereas a single donor horse with the highest MSC yields
was used as a source of allogeneic stem cells for the organ culture study.
For quality control purposes, an immunophenotypic characterization [23] of all donor MSCs was
performed at passage 5 (P5) and P10. Briefly, untreated as well as chondrogenically-induced MSCs were
tested by flow cytometry for the following stem cell markers: mouse anti-human CD29-APC (Biolegend),
mouse anti-horse CD44-FITC (AbD Serotec) and mouse anti-horse CD90 PE-Cy7 (VMRD); and for the
adult blood cell (negative) markers: mouse anti-human CD45 RPE-Cy5.5, mouse anti-horse major
histocompatibility complex (MHC) type II-PE and monocyte/macrophage mouse anti-human macrophages-
Alexa 488 (all from AbD Serotec). Cross reactivity of negative markers with equine epitopes was assessed
using equine peripheral blood mononuclear cells as previously reported [23].
Stimulation of MSCs (chondrogenic induction, PEMFs). At P4, MSCs were seeded for either standard
expansion, chondrogenic induction or chondrogenic induction in combination with stimulation with pulsed
electromagnetic fields (PEMFs). Cells were chondrogenically induced as previously reported [19, 20] by
culturing 6.7x103 MSCs/cm² until the next confluency in a medium consisting of DMEM LG, 20% FCS, 1%
AB/AM (Penicillin-Streptomycin-Amphotericin B), 1.7x10-4 % insulin-like growth factor (IGF)-I and 1.0x10-5 % transforming growth factor (TGF)-β. The seeding protocol was dependent on the type of study (see
For the cell culture study (Fig. 1) that aimed at detecting the effect of chondrogenic induction and
PEMF treatment on the expression of cartilage-specific markers, MSCs were seeded at a density of 1 x104
MSCs/cm² in T25 flasks. For the organ culture experiments in which the aim was to analyse the regenerative
capacity of MSCs as a function of treatment (induction, PEMF, loading, seeding density), MSCs were seeded
at a density of 1 x104 MSCs/cm² in T75 flasks. In order to obtain a sufficient number of cells for seeding 2
different cell densities onto created lesions (0.5 or 1 x106 MSCs per cartilage sample, 9 cartilage samples to
be treated with MSCs), nine T75 flasks were prepared for each of the 3 conditions. The experimental set-up
is illustrated in Fig. 1.
198.143.55.1 - 10/27/2015 12:26:51 PM
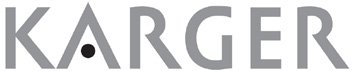
Cell Physiol Biochem 2015;37:651-665
DOI: 10.1159/000430384
2015 S. Karger AG, Basel
Published online: September 08, 2015
Spaas et al.: Chondrogenic Priming Enhances MSC Adhesion
Fig. 1. Study outline
assessing peripheral
blood-derived MSCs in
a cell and organ culture
For both experiments (cell/organ culture), PEMF stimulation at 1mT and 0.5mT was applied to the
respective samples for a single exposure of 10 minutes using a custom-made device [24]. Growth factor-
based chondrogenic induction was conducted as previously described [19, 20].
Analysis of changes in chondrogenic marker gene expression. In the cell culture study, changes in the
gene expression of the chondrogenic markers oligomeric matrix protein (COMP), aggrecan (ACAN) and
collagen type II (COL II) upon stimulation of MSCs with chondrogenic medium with or without PEMF were
analysed by qPCR. As indicated in Fig. 1, MSCs were cultured in normal expansion medium or induction
medium for 6, 24 or 72 hours of culture before being lysed in 1 ml of Trizol (Invitrogen) and RNA was
isolated by standard chloroform phase separation (250 µl, Sigma-Aldrich) as previously described [25].
RNA was quantified on the Nanodrop Lite (Fisher Scientific) before reverse transcribing 1 µg of RNA into
cDNA (10 minutes at 25°C, 120 minutes at 37°C), using the TaqMan Reverse Transcription Reagents Kit
(Life Technologies). cDNA was mixed with equine specific primers (Applied Biosystems, Assays on Demand:
COMP = Ec03468079_g1, ACAN = Ec03469667_m1, COL II = Ec03467386_g1, GAPDH = Ec03210916_gH)
and PCR Fast Master Mix (Applied Biosytems) and gene expression was analysed in triplicate on the Biorad
CFX96 Touch Real-Time PCR detection system as previously reported [19].
Values were normalized to GAPDH (house-keeping gene) and are presented as fold change relative
to uninduced MSCs (i.e. MSCs in expansion medium without PEMF treatment), using the comparative CT
method (= 2-∆∆CT method).
Organ Culture StudyPKH 26 labeling of MSCs. For the organ study, MSCs were cultured in normal expansion medium
or induction medium in the dedicated samples as indicated in Fig. 1. Upon 60% confluency, MSCs were
harvested by trypsin treatment and fluorescently labeled with the PKH26 Red Fluorescent Cell Linker Kit
for General Cell Membrane Labeling according to the manufacturer's protocol (Sigma-Aldrich) to allow
long-term cell tracking upon seeding onto cartilage samples. Briefly, MSCs from one treatment condition
were pooled, centrifuged at 300 G for 8 minutes, counted by hemacytometer and aliquoted into nine cell
suspension portions (= 3 horses per experiment at 3 time points per treatment) of either 0.5 or 1 x106 MSCs
each (to be seeded onto one cartilage explant each). After centrifugation, individual MSC samples were
resuspended in 50 µl of DILUENT C, followed by addition of the same volume of PKH26 stain and incubated
for 5 minutes with periodic mixing. After incubation, 100 µl of FCS was added to block the reaction and
labelled MSCs were retrieved by centrifugation (400 G, 5 minutes) following one wash cycle with PBS.
In a pilot study, the sustainability of the PKH26 labelling of the herein used cell type was examined and
confirmed in 2D culture for up to 18 days (data not shown).
Sampling and incision of cartilage explants. At the slaughter house cartilage explants were aseptically
excised from the patellar cartilage from six slaughtered horses between 4 and 21 years-old (4 mares and 2
198.143.55.1 - 10/27/2015 12:26:51 PM
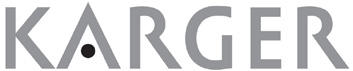
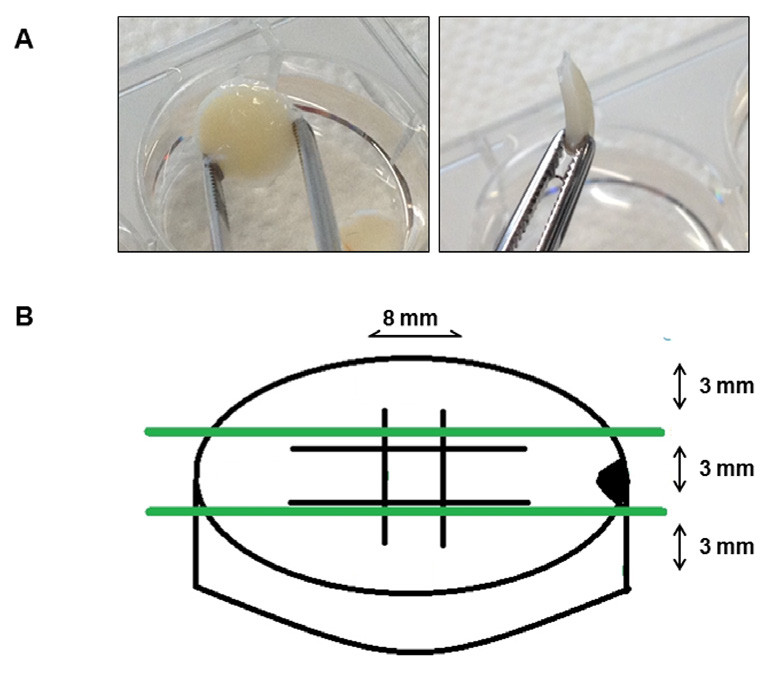
Cell Physiol Biochem 2015;37:651-665
DOI: 10.1159/000430384
2015 S. Karger AG, Basel
Published online: September 08, 2015
Spaas et al.: Chondrogenic Priming Enhances MSC Adhesion
geldings) using an 8mm biopsy punch (n=30 per horse). The biopsies were removed from the subchondral
bone with a scalpel blade, placed separately in 15 ml tubes containing sterile PBS plus penicillin (100 units/
ml), streptomycin (100 µg/ml) and amphotericin (250 ng/ml) and transported to the laboratory. Samples
were rinsed with the same solution under the laminar flow and individually placed in 24 well plates with
1 ml of expansion medium (see above) to keep samples hydrated. One explant at a time from the lesion
groups was removed from the well, positioned on a sterile gauze and treated with a 1.5mm deep incision in
the centre of the biopsy (2 parallel cuts of 4mm length each, plus 2 identical cuts in perpendicular plane as
shown in Fig. 2), created through a number 15 scalpel blade with a custom-made holder to control depth
of incision. On top of the front side of each biopsy, an oblique 3mm punch biopsy lesion was created for
MSC application onto cartilage explants. Cells were washed once, centrifuged at 400G for 5 minutes
and resuspended at a dose of 0.5 or 1 x105/µl in 10 µl of bioreactor medium (expansion medium with
penicillin (100 units/ml), streptomycin (100 µg/ml) and amphotericin (250 ng/ml)).
Biopsies were positioned into 24-well plates containing 200 µl of bioreactor medium, with the injured
side (if applicable) facing upwards. 1 x 106 of the PKH 26 labelled MSCs from the respective treatment
groups were resuspended in 10 µl of expansion medium and slowly seeded on top of their respective
explant. The other half of the explants were covered with 0.5 x 106 cells in 10 µl of expansion medium. In
cell-free samples, 10 µl of pure expansion medium was added to the explant. After allowing MSCs to attach
to the explants for approximately 20 min in the incubator, 800 µl of bioreactor medium was carefully added
per well in order to cover the whole sample with medium.
Fourteen hours later, the explants were brought to new wells with fresh media and non-adhered cells
were visualized and photographed using a fluorescence microscope. In order to determine the percentage
of MSCs that attached to the cartilage explant, the media of the old wells was collected and cells attaching
to the plastic surface were harvested by trypsin treatment and pooled with the culture medium, followed
by cell counting.
All cartilage explants were positioned in new wells with fresh medium each day and maintained
at 37°C with 5% CO , independent of whether mechanical loading was applied, or not (see below). The
remaining 24-well plates were analysed each day for floating and detached cells.
Loading of cartilage explants. 40 hours after the initial seeding, half of the cartilage explants that were
assigned to daily physiological loading were exposed to their first loading cycle at 37°C, while non-loaded
samples were maintained in the incubator. For physiological dynamic loading of cartilage explants, a custom-
made bioreactor was used that allows simultaneous loading of six specimens at a time [26]. Briefly, cartilage
explants were positioned between two metal plates of a sterile loading device positioned in 50 ml Falcon
tubes containing 20 ml of bioreactor medium to fully cover the specimens throughout the loading phase.
Dynamic loading was performed on the Instron E10000 (Instron International) for 1 hour a day at 40 N per
explant (1 Hz) and the same time point each day. After 1, 5 and 14 days, the respective samples (as well as
the unloaded samples) were fixed for histological analysis (see below).
Histological scoring of cartilage explants. At the respective time point (day 1, 5 and 14), the specimens were
fixed in neutral buffered 10% formalin, embedded in paraffin, sectioned at 4 µm thickness and stained
Fig. 2. 8mm cartilage punch biopsies of 3mm thick-
ness were removed from the equine femoropatellar
joint (A). Two parallel cuts of 4mm length were cre-
ated in the horizontal and vertical plane creating a
central grid (B). On top of the front side of each biop-
sy, an oblique 3mm punch biopsy lesion was created
for histological orientation (B).
198.143.55.1 - 10/27/2015 12:26:51 PM
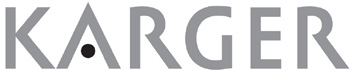
Cell Physiol Biochem 2015;37:651-665
DOI: 10.1159/000430384
2015 S. Karger AG, Basel
Published online: September 08, 2015
Spaas et al.: Chondrogenic Priming Enhances MSC Adhesion
with Hematoxylin & Eosin, Safranin O and Alcian Blue. All sections were blindly scored by an anatomical
pathologist (RF). The following parameters were considered: lesion presence (0 = not present or 1 =
present); surface cell adhesion (0 = not present or 1 = present); cell integration (0 = not present or 1 =
present); number of cell layers; chondrocyte necrosis (0 = no, 1 = moderate or 2 = severe); chondrone
formation grade (0 = no, 1 = moderate or 2 = excessive); chondrone density (1 = 2 cell layers, 2 = 2 to 5 cell
layers, 3 = more than 5 cell layers); lesion filling (0 = no, 1 = moderate or 2 = extensive); clump presence (0
= not present or 1 = present) and number of clump layers.
Statistical analysisFor the cell culture study, gene expression of treatment groups (induction, PEMFs) was analysed
based on a mixed model with horse as random effect and time, medium and/or PEMF stimulation and their
two-way interaction as categorical fixed effects. The F-test at 5% significance level and Tukey's adjustment
method were used for multiple comparisons.
For the organ culture study, the stratified Wilcoxon rank sum test for response variables with more
than two levels and the stratified McNemar test for response variables only two levels were used. Loaded
explants were compared with unloaded explants stratifying for horse, treatment and time. Treatments
were compared stratifying for horse and time and only using data without loading. Finally, time points were
compared stratifying for horse and treatment and only using data without loading.
In order to compare the two doses, Student T-test was used to compare the average horse values
(averaged over time, treatment and loading).
In all analyses, a significance level of 5% was used.
Cell Culture Study
MSC response to chondrogenic induction. The effects of chondrogenic induction medium
were analysed on the gene expression level (mRNA expression of COMP, ACAN and COL
II) as well as on the protein level (Alcian Blue staining, counterstained with Hematoxylin
for visualizing unstained cells). PCR revealed an increase in cartilage specific genes after
chondrogenic induction of the MSCs in three different horses (Fig. 3A). While ACAN
expression increased only 2-3 fold upon exposure to induction medium, which was not
statistically significant (p = 0.2300), COMP expression significantly increased 5-6 fold (p
= 0.0488). In contrast, no considerable effects were observed for COL II at any of these early
time points, indicating limited chondrogenic induction (Fig. 3A).
Flow cytometry revealed no significant changes in the percentage of positive (CD29,
CD44 and CD90) and negative (CD45, MHC II and monocyte/macrophage marker) stem cell
markers over time (p = 0.37) nor after chondrogenic induction at P5 (p = 0.51) and P10 (p
= 0.28) in all horses (Fig. 4A & B). This confirmed maintenance of MSC immunophenotypic
properties over the course of 72 hours, hence supporting the notion of early chondrogenic
induction. A spindle-shaped morphology could be noticed in uninduced MSC cultures (Fig.
4C), whereas chondrogenic induced MSCs displayed a more rectangular morphology (Fig.
4D). Alcian Blue staining confirmed the presence of glycosaminoglycans in the chondrogenic-
induced group (Fig. 4D), whereas no blue staining was observed in MSCs cultured in
expansion medium (Fig. 4C).
MSC response to PEMF. COL II expression in MSC cultures exposed to 1mT of PEMFs for
10 minutes increased significantly (p = 0.0405) after 24 hours in chondrogenic induction
medium (Fig. 3B). PEMF exposure of MSCs cultured in expansion medium at either 0.5 or
1mT amplitudes did not result in a significant increase (p = 0.8521) in any of the evaluated
cartilage genes (i.e. ACAN, COL II and COMP) at any of the time points in comparison to
unstimulated MSCs (Fig. 3C presents 24 hour time point as example). In contrast with
0.5mT, the 1mT stimulated MSCs cultured in chondrogenic induction medium resulted in
a significant increase in COL II expression (Fig. 3D), revealing a culture medium- and PEMF
dose-dependence. Hence, at 24 hours PEMF stimulation was able to significantly enhance
198.143.55.1 - 10/27/2015 12:26:51 PM
Cell Physiol Biochem 2015;37:651-665
DOI: 10.1159/000430384
2015 S. Karger AG, Basel
Published online: September 08, 2015
Spaas et al.: Chondrogenic Priming Enhances MSC Adhesion
Fig. 3. RT-PCR for gene expression of ACAN, COL II and COMP. Increase of cartilage gene expression after
chondrogenic induction in comparison to uninduced MSCs (A). Effect of PEMF on COL II expression of un-
induced and chondrogenic induced MSCs at different time points (B). Effect of 0.5 mT and 1 mT of PEMF
stimulation on the expression of different cartilage genes in uninduced (C) and chondrogenic induced MSCs
(D) at 24 hours. Values are given as the mean of three measurements ± SEM; * indicates p < 0.05.
COL II expression. We next tested the ability of the combination of PEMFs and induction to
improve the regenerative capacity of MSCs in an organ culture.
Organ Culture Study
PKH26 labelling and cell adhesion. PKH26 labelled MSCs could be visualized in more
than 90% of the cells until day 18 after culturing in control wells, indicating a suitable
staining for the 14 day explant experiment. At 14 hours after adding the cells on the explants,
non-adhered cells clearly contained the fluorescent label as confirmed by fluorescence
microscopy analysis (Fig. 5). Table 1 indicates the percentage of cells that initially did not
attach to the cartilage explants of the six horses before loading, shown separately for each
study group. In explant cultures designated for loading, an average of 38.6 ± 14.0% of the cells
was not able to attach (Table 1). For unloaded explants this remained within the same range
(35.9 ± 12.5%). In the low (0.5 x106 MSCs) and high (1 x 106 MSCs) seeding density groups,
an average of 41.3 ± 12.6% versus 33.1±12.8% of the cells did not attach to the explants,
respectively (Table 1). No significant differences in cell losses before loading were found
between explants treated with high and low density of MSCs. After 24 hours, no more cells
were detected in the supernatant or at the bottom of the wells. At each time point, adhered
MSCs were visualized with a fluorescence microscope; however, no more red fluorescent
signal could be detected after tissue sectioning.
Histological results of loaded cartilage explants. Daily compressive physiological loading
was applied to cartilage explants in order to enhance the in vivo comparability. Although
loading was only as high as 40 N for 1 hour per day at 1 Hz, it had severe effects on the
presence of MSCs (independent of the pre-treatment of cells). Taking all the time points
together, after exposure to loading, only 1.9% or 1 out of 54 (= 3 time points x 6 horses x
3 MSC treatment groups) cartilage explants demonstrated adherent MSCs on their surface
198.143.55.1 - 10/27/2015 12:26:51 PM
Cell Physiol Biochem 2015;37:651-665
DOI: 10.1159/000430384
2015 S. Karger AG, Basel
Published online: September 08, 2015
Spaas et al.: Chondrogenic Priming Enhances MSC Adhesion
Fig. 4. Flow cytometry confirmed a positive expression for CD29, CD44 and CD90 compared to isotype
control (A), whereas no expression for a monocyte/macrophage marker, major histocompatibility complex
(MHC) type II and CD45 could be observed at P5 and P10 on chondrogenic induced MSCs (B). Light micro-
scopic images of MSCs in their undifferentiated state (C) and chondrogenic induced state (D) after Alcian
Blue staining. Glycosaminoglycan production can be noticed after induction. Scale bars represent 50µm.
Fig. 5. Phase contrast
(A), fluorescence (B) and
overlay (C) images of
PKH26-labelled MSCs in
the different treatment
groups at 14 hours after
seeding: uninduced (I),
chondrogenic induced
(II) and PEMF stimulated
chondrogenic induced
(III) MSCs. Scale bars
represent 50 µm.
(Fig. 6) which resulted in absence of clump formation in the loaded group. Consequently,
lesion filling was only observed in 3.7% or 2 out of 54 cartilage explants (Fig. 6).
198.143.55.1 - 10/27/2015 12:26:51 PM
Cell Physiol Biochem 2015;37:651-665
DOI: 10.1159/000430384
2015 S. Karger AG, Basel
Published online: September 08, 2015
Spaas et al.: Chondrogenic Priming Enhances MSC Adhesion
Table 1. Percentage of
cells that initially did not
attach on the loaded (L)
and unloaded (U) carti-
lage explants at 14 hours
after seeding and before
the first loading session
Fig. 6. Representative images of
loaded versus unloaded cartilage
explants at 40-fold magnification.
Different groups are illustrated: no
lesion without MSCs (A), only a le-
sion without MSCs (B), lesion with
MSCs (C), lesion with chondrogenic
induced MSCs (D) and lesion with
PEMF stimulated chondrogenic in-
duced MSCs (E). Boxes represent
200 fold magnifications.
Histological results of unloaded explants. Taking all three time points of unloaded MSC-
treated groups (iii, iv and v) together, adherent MSCs were present in 92.6% of the explants
(= 50/54 = (Day 1 = 18/18) + (Day 5 = 17/18) + (Day 14 = 15/18)), which is significantly
more than 1.9% in the loaded explants (p < 0.0001). It made it also possible to histologically
score and compare the different unloaded treatment groups. For all the scored parameters,
no significant differences between chondrogenically-induced MSCs with or without PEMF
stimulation could be demonstrated. On the other hand, in chondrogenic induced MSC
groups (with or without PEMF), a homogenous cell distribution and no cell clumps were
more characteristic, whereas 7 out of 18 (39%) uninduced MSC treated cartilage explants
exhibited cell clumps of at least 8 cell layers thickness. Clumping incidence was significantly
different from the other treatment groups (p = 0.0156) and was independent of cell seeding
number. The number of explants with lesion filling (40.7% = 22/54) significantly decreased
(p = 0.0156) in the unloaded group from day 5 (n=11/18) to day 14 (n=4/18), in contrast to
what was observed from day 1 (n=7/18) to 5 (p = 0.2188). Although no more clumps were
noticed in any explant culture at day 14 (Fig. 7), the number of explants that demonstrated
adhered MSCs on their surface did not significantly alter over time (p = 1.000, p
198.143.55.1 - 10/27/2015 12:26:51 PM
Cell Physiol Biochem 2015;37:651-665
DOI: 10.1159/000430384
2015 S. Karger AG, Basel
Published online: September 08, 2015
Spaas et al.: Chondrogenic Priming Enhances MSC Adhesion
Fig. 7. Representative images of unload-
ed explants at a 40 and 200 fold magni-
fication. Cartilage explants treated with
uninduced MSCs contain clumps at day
1 (A) and day 5 (B), whereas clumps
detached before day 14 (C). Cartilage
explants after treatment with chondro-
genic induced MSCs demonstrate a ho-
mogenous cell adhesion which remains
constant from day 1 (D), towards day 5
(E) and day 14 (F).
0.6250, Fig. 7). The adhered non-clumped cell layers also remained relative constant over
time (p = 1.000, p
= 0.1250, Fig. 7).
Taking all treatment scores in unloaded conditions together for the three horses treated
with 0.5 x 106 MSCs (low density) versus the other three horses treated with 1 x 106 MSCs
(high density), significantly more cartilage explants demonstrated lesion filling (p = 0.0022)
and surface adhered MSCs (p = 0.0161) in the low density group. The average score for
lesion filling was 0.67 per low density treated cartilage explant and 0.15 for the high density
treated explants. Concerning adhered MSCs, an average score of 1.00 demonstrated presence
of MSCs on the surface of each low density explant, whereas this was not the case for the high
density MSC application (average score of 0.85). On the other hand, no significant difference
concerning integration (p = 0.6779), cell layers (p = 0.6974), clump formation (p = 0.6433)
and clump layers (p = 0.6340) could be noticed between both.
In the present study, peripheral blood (PB)-derived mesenchymal stem cells (MSCs)
of three horses were successfully isolated and chondrogenically induced as confirmed by
PCR and histology. Briefly, flow cytometry confirmed no loss of the typical MSC markers
during the predifferentiation process. Half of the 180 cartilage explants were loaded in order
to assess the cartilage integrity and integration capacities of fluorescently labelled MSCs
under physiological compression at three time points (day 1, 5 and 14). Proliferation and
differentiation are largely mutually exclusive processes in chondrogenesis. Chondrogenic
198.143.55.1 - 10/27/2015 12:26:51 PM
Cell Physiol Biochem 2015;37:651-665
DOI: 10.1159/000430384
2015 S. Karger AG, Basel
Published online: September 08, 2015
Spaas et al.: Chondrogenic Priming Enhances MSC Adhesion
induction was performed for only three days in a 2-dimensional culture system in order
to maintain MSC proliferative capacity in an injectable cell-based product undergoing the
initial stages of differentiation. The conservation of MSC markers and limited cartilage gene
upregulation both indicate that the cells were in a prechondroblastic stage and not fully
differentiated yet.
In order to track MSCs, labelling with the membrane incorporating substance PKH26
was performed, which was considered a suitable alternative to intranuclear labelling
methods (e.g. green fluorescent protein GFP labelling) due to its well-described low toxicity
and labelling sustainability in progenitor-like cells [27-30]. A preliminary experiment on
PB-MSCs in 2D culture confirmed detectability of the fluorescent signal until day 18 (data
not shown), yet moderately diluted due to cell division, as previously reported for pig
chondrocytes [31]. Although successful fluorescent labelling of MSCs with PKH26 could
be confirmed at the beginning of the organ culture study (on day 1 after seeding), the
fluorescent signal could not be detected in the histological sections of the seeded cartilage
explants. In contrast, other studies have previously described successful fluorescence-based
detection of implanted, PKH-labelled MSCs and chondrocytes after 6 weeks (sheep) [32] or
12 weeks (mice) [31] in vivo, respectively. The exact reasons for the loss of fluorescent signal
will have to be investigated in detail in future studies, but may be related to differences in the
experimental condition and histological processing.
Although fluorescence labelling of MSCs was not detectable in the cartilage explants,
MSCs could be identified based on their typical spindle-shaped morphology as well as by
immunohistochemistry staining for MSC markers (data not shown). The spindle-shaped
morphology at each analysis time point (after adding to the explants) indicates that MSCs
did not attain fully differentiated chondrogenic features during two weeks of explant culture.
This might be explained by the brief culture period (before adding to the explants) and the
fact that, after a three-day induction period, no more cartilage inducing growth factors were
used. A previous study using cartilage specific medium reports chondrogenic differentiation
of integrated porcine bone marrow-derived MSCs after 6 weeks of culture in cartilage explant
defects coated with agarose hydrogels [33].
In this study, the effects of chondrogenic induction, PEMF treatment, seeding density
and mechanical loading on MSC adherence and functionality were analysed. After mechanical
loading, significantly (p < 0.0001) less explants exhibited lesion filling (3.7% vs 40.7%) or
cell adherence (1.9% vs 92.6%). As physiological pressures (ca. 0.8 MPa) were applied that
are also expected to arise during moderate training, this finding indicates that mechanical
loading - if applied soon after implantation/injection - may mechanically interfere with the
integration of MSCs into isolated explants and undermine a potentially beneficial outcome.
Cartilage contact pressures of 0.8 MPa can be considered to be in the range of that acting
in vivo on e.g. the equine stifle joint during normal ambulation, as peak pressures up to 1.5
– 2.0 MPa have been measured by Fowlie et al. [34]. Due to the weight and physiology of
equine joints together with the robustness of equine subchondral bone [35], our findings
may expose some restrictions for future clinical application. However, it has been reported
that joint loading is highly dependent on the joint type and joint compartment [36]. In the
present study, patellar cartilage was taken and it has been reported that this compartment is
relatively unloaded during stall confinement and supervised walking, which could explain the
sensitivity to loading [36, 37]. The weight-bearing medial and lateral compartments on the
other hand experience more in vivo loading, which might compromise the treatment. Further
in vitro bioreactor tests with different loading patterns as well as tests under physiological
circumstances will need to be performed to eventually make exercise recommendations for
MSC-treated OA patients.
Chondrogenically-induced MSCs displayed superior adhesion and integration capacity
in comparison to uninduced MSCs in this study. Homogenous layers of chondrogenic induced
MSCs could be noticed all over the explants, whereas in 39% of the uninduced MSCs large
clumps of at least 8 cell layers were observed predominantly at areas on top of the explants
which detached before day 14. Overall, a more efficient cell adhesion and integration
198.143.55.1 - 10/27/2015 12:26:51 PM
Cell Physiol Biochem 2015;37:651-665
DOI: 10.1159/000430384
2015 S. Karger AG, Basel
Published online: September 08, 2015
Spaas et al.: Chondrogenic Priming Enhances MSC Adhesion
could be noticed in explants seeded with chondrogenic induced MSCs. On the other hand,
the adhered non-clump cell layers remained relative constant over time, independent of
predifferentiation. The beneficial effect of predifferentiation has also been reported in vivo in
165 horses, where clinical outcomes were improved upon chondrogenic predifferentiation
[20].Seeding density also had a significant effect on the outcome (in unloaded specimens).
Interestingly, low cell densities (27 replicates = 3 horses, 3 treatment circumstances at 3 time
points) demonstrated significantly more lesion filling than higher cell density treatments. In
addition, all low cell density treated explants demonstrated adhered MSCs to their surface,
whereas this was not the case for the high cell density treatment. Although dose-dependent
effects have been reported for allogeneic MSCs for the treatment of myocardial infarction in
rats [38] and graft versus host disease in mice [39], others have also demonstrated a superior
outcome using a lower density of allogeneic MSCs for treatment of injured medial collateral
ligaments of rat knees [40] or for the treatment of human knee osteoarthritis [41]. As clump
formation did not differ between the cell density groups, the difference was unlikely due to
steric obstruction. Hence, more research is warranted to identify the optimal cell density
of MSC treatments (rather than the lowest effective dose) such as dose-response studies
typical for clinical medicine.
Whereas predifferentiation, loading and seeding density had significant impact on this
study's outcome measures, PEMF treatment did not obviously improve any of the evaluated
cartilage regeneration parameters in this organ culture study. On the other hand, in the cell
culture study an increase in COL II expression was observed in response to PEMF stimulation.
Alternative approaches to improve regeneration capacity in explants treated with PEMFs may
include: 1) increasing exposure amplitude; 2) increasing exposure duration; 3) increasing
number of exposures to a value greater than 1; 4) exposing MSC-seeded explants directly
to PEMFs or; 5) a combination of the above. In fact, Esposito et al. have previously reported
induction of chondrogenic differentiation upon PEMF treatment [14] and several in vivo
studies have demonstrated beneficial effects of this therapy on clinical symptoms of animals
and humans with osteoarthritis [11, 12]. It has to be mentioned though that these studies
applied 6 weeks of daily treatment of the injured joint, whereas our study employed single 10
minute prestimulation of chondrogenically induced MSCs and unstimulated ex vivo cartilage
explants. Future research focussing on PEMF-treated cartilage explants might provide more
insights into the mechanisms of PEMF stimulation at a tissue level. Such studies might also
clarify whether PEMF-treatment evokes cellular cascades of non-cartilage origin.
In conclusion, our results indicate that chondrogenic induction was successful in PB-
MSCs and resulted in improved outcome with regard to MSC adhesion and integration.
PEMF-treatment was able to enhance the expression of COL II in chondrogenically-induced
MSCs, which was previously insensitive to induction alone. The application of lower densities
of MSCs to explants proved superior to higher MSCs densities, whereas PEMF-treatment
exerted no obvious benefit over induction alone in explants. Importantly, immediate
physiological loading negatively influenced MSC adhesion to explants, which may have
significant implications for the early rehabilitation strategy of patients undergoing MSCs
treatment for cartilage regeneration. Future studies will aim to analyse the time-dependent
effect of loading of MSC-seeded cartilage explant.
The authors would like to thank Dr. Luk Van Esbroeck of the Euro Meat Group and
Chevideco for allowing an optimal and sterile cartilage collection. Sarah Loomans, Christian
Puttevils and Delphine Ameye from the Department of Pathology, Bacteriology and Poultry
Diseases (Ghent University) as well as Suchandrima Das and Helen Greutert (ETH Zurich)
for their technical assistance. We would also like to thank Dr. Jürg Fröhlich and Dr. Christian
Beyer of the Department Information Technology and Electrical Engineering of the ETH,
198.143.55.1 - 10/27/2015 12:26:51 PM
Cell Physiol Biochem 2015;37:651-665
DOI: 10.1159/000430384
2015 S. Karger AG, Basel
Published online: September 08, 2015
Spaas et al.: Chondrogenic Priming Enhances MSC Adhesion
Zurich, for supporting the PEMF apparatus used in this study. In addition, the Federal Public
Service of Health should be acknowledged for providing GST with a laboratory recognition
number (LA1700607), allowing us to perform this study.
This study was supported by a grant (number 130543) to JHS and SYB from the agency
for innovation by science and technology Flanders (IWT Vlaanderen). Furthermore, the
authors would like to acknowledge GST and the ANACURA group with the sources of private
funding, which have provided the basis for this study.
The author JHS declares competing financial interests as shareholder in Global Stem
cell Technology (GST). SYB and JHS are both employed by GST and inventors of several
pending patents owned by GST (BE2012/0656; WO2014053418A9; WO2014053420A1;
PCT/EP2013/075782). All other authors declare no conflicts of interests. The content of
this manuscript contains a product under development owned by GST.
Barbour KE, Helmick CF, Theis KA, Murphy LB, Hootman JM, Brady TJ, Cheng YJ: Prevalence of Doctor-Diagnosed Arthritis and Arthritis-Attributable Activity Limitation — United States, 2010–2012. Morbidity and Mortality Weekly Report (MMWR), National Center for Chronic Disease Prevention and Health Promotion, CDC 2013;62:869-873.
Murphy L, Schwartz TA, Helmick CG, Renner JB, Tudor G, Koch G, Dragomir A, Kalsbeek WD, Luta G, Jordan JM: Lifetime risk of symptomatic knee osteoarthritis. Arthritis Rheum 2008;59:1207-1213.
Hiligsmann M, Cooper C, Arden N, Boers M, Branco JC, Luisa Brandi M, Bruyere O, Guillemin F, Hochberg MC, Hunter DJ, Kanis JA, Kvien TK, Laslop A, Pelletier JP, Pinto D, Reiter-Niesert S, Rizzoli R, Rovati LC, Severens JL, Silverman S, Tsouderos Y, Tugwell P, Reginster JY: Health economics in the field of osteoarthritis: an expert's consensus paper from the European Society for Clinical and Economic Aspects of Osteoporosis and Osteoarthritis (ESCEO). Semin Arthritis Rheum 2013;43:303-313.
Caron JP, Genovese RL: Principals and practices of joint disease treatment; in: Ross MW, Dyson S (eds): Diagnosis and management of lameness in the horse. 1st ed. Philadelphia, Saunders, 2003, pp 746–764.
Frisbie DD: Future directions in treatment of joint disease in horses. Vet Clin North Am Equine Pract 2005;21:713-724,viii.
Jeffcott LB, Rossdale PD, Freestone J, Frank CJ, Towers-Clark PF: An assessment of wastage in thoroughbred racing from conception to 4 years of age. Equine Vet J 1982;14:185-198.
Hart BA, Bontrop RE: The relevance of arthritis research in non-human primates. Br J Rheumatol 1998;37:239-242.
Charlesworth B, Charlesworth D: Evolution of the Genome; in Ruse M, Travis J (eds): Evolution: The First Four Billion Years. Harvard College, 2009, pp 141-161.
Frisbie DD, Cross MW, McIlwraith CW: A comparative study of articular cartilage thickness in the stifle of animal species used in human pre-clinical studies compared to articular cartilage thickness in the human knee. Vet Comp Orthop Traumatol 2006;19:142-146.
10 U.S. Food and Drug Administration: Cellular Products for Joint Surface Repair. Cellular, Tissue, and Gene
Therapies Advisory Committee. 2005; Meeting # 38:27.
11 Boopalan PR, Arumugam S, Livingston A, Mohanty M, Chittaranjan S: Pulsed electromagnetic field therapy
results in healing of full thickness articular cartilage defect. Int Orthop 2011;35:143-148.
12 Iannitti T, Fistetto G, Esposito A, Rottigni V, Palmieri B: Pulsed electromagnetic field therapy for
management of osteoarthritis-related pain, stiffness and physical function: clinical experience in the elderly. Clin Interv Aging 2013;8:1289-1293.
13 Trock DH, Bollet AJ, Markoll R: The effect of pulsed electromagnetic fields in the treatment of osteoarthritis
of the knee and cervical spine. Report of randomized, double blind, placebo controlled trials. J Rheumatol 1994;21:1903-1911.
198.143.55.1 - 10/27/2015 12:26:51 PM
Cell Physiol Biochem 2015;37:651-665
DOI: 10.1159/000430384
2015 S. Karger AG, Basel
Published online: September 08, 2015
Spaas et al.: Chondrogenic Priming Enhances MSC Adhesion
14 Esposito M, Lucariello A, Costanzo C, Fiumarella A, Giannini A, Riccardi G, Riccio I: Differentiation of human
umbilical cord-derived mesenchymal stem cells, WJ-MSCs, into chondrogenic cells in the presence of pulsed electromagnetic fields. In Vivo 2013;27:495-500.
15 Chen CH, Lin YS, Fu YC, Wang CK, Wu SC, Wang GJ, Eswaramoorthy R, Wang YH, Wang CZ, Wang YH, Lin SY,
Chang JK, Ho ML: Electromagnetic fields enhance chondrogenesis of human adipose-derived stem cells in a chondrogenic microenvironment in vitro. J Appl Physiol (1985) 2013;114:647-655.
16 Frisbie DD, Kisiday JD, Kawcak CE, Werpy NM, MccIlwraith CW: Evaluation of adipose-derived stromal
vascular fraction or bone marrow-derived mesenchymal stem cells for treatment of osteoarthritis. J Orthop Res 2009;27:1675-1680.
17 McIlwraith CW, Frisbie DD, Rodkey WG, Kisiday JD, Werpy NM, Kawcak CE, Steadman JR: Evaluation
of intra-articular mesenchymal stem cells to augment healing of microfractured chondral defects. Arthroscopy 2011;27:1552-1561.
18 Wilke MM, Nydam DV, Nixon AJ: Enhanced early chondrogenesis in articular defects following arthroscopic
mesenchymal stem cell implantation in an equine model. J Orthop Res 2007;25:913-925.
19 Broeckx S, Zimmerman M, Crocetti S, Suls M, Marien T, Ferguson SJ, Chiers K, Duchateau L, Fraco-Obregon
A, Wuertz K, Spaas JH: Regenerative therapies for equine degenerative joint disease: a preliminary study. PLoS One. 2014;9:e85917.
20 Broeckx S, Suls M, Beerts C, Vandenberghe A, Seys B, Wuertz-Kozak K, Duchateau L, Spaas JH: Allogenic
mesenchymal stem cells as a treatment for equine degenerative joint disease: a pilot study. Curr Stem Cell Res Ther 2014;9:497-503.
21 Chen K, Man C, Zhang B, Hu J, Zhu SS: Effect of in vitro chondrogenic differentiation of autologous
mesenchymal stem cells on cartilage and subchondral cancellous bone repair in osteoarthritis of temporomandibular joint. Int J Oral Maxillofac Surg 2013;42:240-248.
22 Broeckx S, Zimmerman M, Aerts D, Seys B, Suls M, Mariën T, Spaas JH: Tenogenesis of equine peripheral
blood-derived mesenchymal stem cells: in vitro versus in vivo. J Tissue Sci Eng 2012;S11-001:1-6.
23 Spaas JH, Schauwer CD, Cornillie P, Meyer E, Van Soom A, Van de Walle GR: Culture and characterisation of
equine peripheral blood mesenchymal stromal cells. Vet J 2013;195:107-113.
24 Crocetti S, Beyer C, Schade G, Egli M, Frohlich J, Franco-Obregon A: Low intensity and frequency pulsed
electromagnetic fields selectively impair breast cancer cell viability. PLoS One 2013;8:e72944.
25 Krupkova O, Sekiguchi M, Klasen J, Hausmann O, Konno S, Ferguson SJ, Wuertz-Kozak K: Epigallocatechin
3-gallate suppresses interleukin-1beta-induced inflammatory responses in intervertebral disc cells in vitro and reduces radiculopathic pain in rats. Eur Cell Mater 2014;28:372-386.
26 Dudli S, Haschtmann D, Ferguson SJ: Persistent degenerative changes in the intervertebral disc after burst
fracture in an in vitro model mimicking physiological post-traumatic conditions. Eur Spine J 2014.
27 Hemmrich K, Meersch M, von Heimburg D, Pallua N: Applicability of the dyes CFSE, CM-DiI and PKH26
for tracking of human preadipocytes to evaluate adipose tissue engineering. Cells Tissues Organs 2006;184:117-127.
28 Shuai H, Shi C, Lan J, Chen D, Luo X: Double labelling of human umbilical cord mesenchymal stem cells with
Gd-DTPA and PKH26 and the influence on biological characteristics of hUCMSCs. Int J Exp Pathol 2015.
29 Qu B, Xin GR, Zhao LX, Xing H, Lian LY, Jiang HY, Tong JZ, Wang BB, Jin SZ: Testing stem cell therapy in a
rat model of inflammatory bowel disease: role of bone marrow stem cells and stem cell factor in mucosal regeneration. PLoS One 2014;9:e107891.
30 Progatzky F, Dallman MJ, Lo Celso C: From seeing to believing: labelling strategies for in vivo cell-tracking
experiments. Interface focus 2013;3:20130001.
31 Weinand C, Johnson TS, Gill TJ: In vitro and in vivo cell tracking of chondrocytes of different origin by
fluorescent PKH 26 and CMFDA. J Biomed Sci Eng 2008;1:163-169.
32 Ude CC, Sulaiman SB, Min-Hwei N, Hui-Cheng C, Ahmad J, Yahaya NM, Saim AB, Idrus RB: Cartilage
regeneration by chondrogenic induced adult stem cells in osteoarthritic sheep model. PLoS One 2014;9:e98770.
33 Vinardell T, Thorpe SD, Buckley CT, Kelly DJ: Chondrogenesis and integration of mesenchymal stem cells
within an in vitro cartilage defect repair model. Ann Biomed Eng 2009;37:2556-2565.
34 Fowlie J, Arnoczky S, Lavagnino M, Maerz T, Stick J: Resection of Grade III cranial horn tears of the equine
medial meniscus alter the contact forces on medial tibial condyle at full extension: an in-vitro cadaveric study. Vet Surg 2011;40:957-965.
198.143.55.1 - 10/27/2015 12:26:51 PM
Cell Physiol Biochem 2015;37:651-665
DOI: 10.1159/000430384
2015 S. Karger AG, Basel
Published online: September 08, 2015
Spaas et al.: Chondrogenic Priming Enhances MSC Adhesion
35 Murray RC, Vedi S, Birch HL, Lakhani KH, Goodship AE: Subchondral bone thickness, hardness and
remodelling are influenced by short-term exercise in a site-specific manner. J Orthop Res 2001;19:1035-1042.
36 Chu CR, Szczodry M, Bruno S: Animal models for cartilage regeneration and repair. Tissue Eng Part B,
37 Ahern BJ, Parvizi J, Boston R, Schaer TP: Preclinical animal models in single site cartilage defect testing: a
systematic review. Osteoarthr Cartil 2009;17:705-713.
38 Richardson JD, Bertaso AG, Psaltis PJ, Frost L, Carbone A, Paton S, Nelson AJ, Wong DT, Worthley MI,
Gronthos S, Zannettino AC, Worthley SG: Impact of timing and dose of mesenchymal stromal cell therapy in a preclinical model of acute myocardial infarction. J Card Fail 2013;19:342-353.
39 Joo SY, Cho KA, Jung YJ, Kim HS, Park SY, Choi YB, Hong KM, Woo SY, Seoh JY, Cho SJ, Ryu KH: Mesenchymal
stromal cells inhibit graft-versus-host disease of mice in a dose-dependent manner. Cytotherapy 2010;12:361-370.
40 Saether EE, Chamberlain CS, Leiferman EM, Kondratko-Mittnacht JR, Li WJ, Brickson SL, Vanderby R:
Enhanced medial collateral ligament healing using mesenchymal stem cells: dosage effects on cellular response and cytokine profile. Stem Cell Rev 2014;10:86-96.
41 Vangsness CT, Farr J, Boyd J, Dellaero DT, Mills CR, LeRoux-Williams M: Adult human mesenchymal
stem cells delivered via intra-articular injection to the knee following partial medial meniscectomy: a randomized, double-blind, controlled study. J Bone Joint Surg Am 2014;96:90-98.
198.143.55.1 - 10/27/2015 12:26:51 PM
Source: http://magnetoterapia-weterynaria.pl/wp-content/uploads/2016/02/Cellular-Physiology-and-Biochemistry.2015.pdf
ISRAEL FÜR „ANFÄNGER UND KENNER" UMWELT – NATUR – GESCHICHTE – NACHHALTIGKEIT Die Reise führt Sie zu ökologischen, sozialen, landwirtschaftlichen Projekten des modernen Israel, ohne dabei die geschichtlichen Orte und Aspekte zu vergessen. 1. Tag, Samstag: Anreise Ankunft Flughafen Tel Aviv. Treffen im Hotel mit unserer Reiseleitung. Je nach Ankunftszeit Rundgang in Tel Aviv oder Yaffo. Ü/HP Tel Aviv.
FISH STOCK AND FISHERY ENHANCEMENT IN WESTERN AUSTRALIA A DISCUSSION PAPER FISHERIES MANAGEMENT PAPER No 175 Department of Fisheries 168 St Georges Terrace Fisheries Management Paper No. 175 Fish stock and fishery enhancement in Western Australia A Discussion Paper Fisheries Management Paper No. 175 Please note the public comment period for Fisheries Management Paper No. 175 Fish Stock and Fishery Enhancement in Western Australia and its companion document, Fisheries Management Paper 176 Fish Stock and Fishery Enhancement in Western Australia (Summary of Fisheries Management Paper No. 175), has been adjusted. The Department of Fisheries advises that the public comment period for both documents is open until 31 May 2004.